upland rice Recently Published Documents
Total documents.
- Latest Documents
- Most Cited Documents
- Contributed Authors
- Related Sources
- Related Keywords

Designing low-input upland rice-based cropping systems with conservation agriculture for climate change adaptation: A six-year experiment in M’bé, Bouaké, Côte d’Ivoire
Research on lncrna related to drought resistance of shanlan upland rice.
Abstract Background Drought has become the major abiotic stress that causes losses in rice yields and consequently is one of the main environmental factors threatening food security. Long non-coding RNA (lncRNA) is known to play an important role in plant response to drought stress, while the mechanisms of competing endogenous RNA (ceRNA) in drought resistance in upland rice have been rarely reported. Results In our study, a total of 191 lncRNAs, 2115 mRNAs and 32 miRNAs (microRNAs) were found by strand-specific sequencing and small RNA sequencing to be differentially expressed in drought-stressed rice. Functional analysis of results indicate that they play important roles in hormone signal transduction, chlorophyll synthesis, protein synthesis and other pathways. Construction of a ceRNA network revealed that MSTRG.28732.3 may interact with miR171 in the chlorophyll biosynthesis pathway and affect the ability of plants to withstand drought stress by regulating Os02g0662700, Os02g0663100 and Os06g0105350. The accuracy of the regulatory network was verified by qRT-PCR. Conclusion Our results provide a theoretical basis for future studies on the potential function of lncRNA in plant drought resistance, and they provide new genetic resources for drought-resistant rice breeding.
Application of local microbes increases growth and yield of several local upland rice cultivars of Southeast Sulawesi, Indonesia
Abstract Rice is the major food commodity in Indonesia and many other countries, as the main source of carbohydrate. Rice production must be increased continuously to meet food needs, one of which is by utilizing largely available dry land areas. Two important factors required to increase rice production on marginal soils are the use of high-production adaptive varieties and biological agents. It is necessary to conduct research on the application of local microbes to the cultivation of upland rice cultivars, with the aim to determine the best genotypes and/or suitable microbes. This research was carried out at the Research Field and laboratories of Faculty of Agriculture, University of Halu Oleo, from April 2020 to April 2021. The first tested factor was biological agents (local microbes), consisting of three levels, namely: without microbes (M0), the fungus Trichoderma sp. (M1), and the bacteria Pseudomonas sp. (M2). The second factor was the upland rice cultivar, which consisted of five levels, namely: V1 (Tinangge cultivar), V2 (Enggalaru cultivar), V3 (Bakala cultivar), V4 (Momea cultivar), and 1 nasional variety, namely V5 (Inpago-12 variety), as check variety. The research results showed that the interaction between local microbes Trichoderma sp. (M1) and Pseudomonas sp. (M2) on Tinangge (V1) and Momea (V4) cultivars gave a better effect on crop production. The best cultivar based on the growth and yield variables was generally obtained from the Momea cultivar (V4), although in many variables it was not significantly different from the Tinangge cultivar (V1). These cultivars could be further studied and possibly developed for promising cultivars in Kendari areas.
Seaweed extract effect on arbuscular mycorrhizae spore in soil engineered by earthworm, and the soil effect on upland rice growth
Abstract Seaweed extract is known to contain nutrients and growth-regulating substances that affect soil biota, and a source of protection against pests and diseases. Earthworm, which is an example of a soil biota and playing the role of ecosystem engineer, has the ability to produce suitable land biostructures, for the inhabitation of arbuscular mycorrhizal fungi (AMF), which has an impact on upland rice growth. Therefore, this study aims to determine, (i) the effect of seaweed extract on the population of earthworms and spores of arbuscular mycorrhizal fungi, and (ii) the impact of the engineered soil on the growth of local upland rice varieties. Furthermore, the extract of seaweed, such as Kappapychus alvarezii, was divided into five concentration levels, namely 0%, 20%, 40%, 60%, and 80%. Each treatment was drenched into the soil from the cogongrass vegetated area, mixed with 20 Pheretima sp., and maintained for 49 days in the greenhouse. The result showed that the total difference in the earthworms’ concentration treatments was not significant. It also showed that the total AMF spores in the engineered soil products of 20% concentration was the highest. Based on treatment with the earthworm engineered soil products, the highest and lowest vegetative growth and yield components of upland rice were observed at the concentrations of 80% and 0%, respectively. In conclusion, the application of seaweed extract to the soil did not significantly reduce the earthworm population. The extract concentration of 20% also increased the total AMF spore in the engineered soil. Moreover, highly treated engineered soil products increased the growth and yield components of upland Kambowa rice on cogongrass soils.
Determination of antifungal volatile organic compounds of upland rice vinegar and their inhibition effects on Aspergillus flavus in dried chili pepper
Continuous maize cropping accelerates loss of soil organic matter in northern thailand as revealed by natural 13c abundance.
Abstract AimsThe loss of soil organic matter (SOM) has widely been reported in the tropics after changing land use from shifting cultivation to continuous cropping. We tested whether continuous maize cultivation accelerates SOM loss compared to upland rice and forest fallow. Methods: Because litter sources include C4 plants (maize in maize fields and Imperata grass in upland rice fields) in Thailand, C3-derived and C4-derived SOM can be traced using the differences in natural 13C abundance (δ13C) between C3 and C4 plants. We analyzed the effects of land use history (cultivation or forest fallow period) on C stocks in the surface soil. Soil C stocks decreased with the cultivation period in both upland rice and maize fields. ResultsThe rate of soil organic carbon loss was higher in maize fields than in upland rice fields. The decomposition rate constant (first order kinetics) of C3-plant-derived SOM was higher in the maize fields than in the upland rice fields and the C4-plant-derived SOM in the forest fallow. Soil surface exposure and low input of root-derived C in the maize fields are considered to accelerate SOM loss. Soil C stocks increased with the forest fallow period, consistent with the slow decomposition of C4-plant-derived SOM in the forest fallows. ConclusionsContinuous maize cultivation accelerates SOM loss, while forest fallow and upland rice cultivation could mitigate the SOM loss caused by continuous maize cultivation.
Upland rice intercropped with green manures and its impact on the succession with common bean
Abstract The aim of this work was to verify the possibility of intercropping rice with green manures, as well as the impact of the dry biomass yield of these intercropping systems on common bean in succession, evaluating the agronomic and qualitative performance of grains from both crops. The experiment was conducted in Southeastern Brazil in the years 2018 and 2019, with succession of rice (spring/summer) and common bean (autumn/winter). The treatments were composed of cropping systems with rice as a sole crop and intercropped with forage peanut, calopo, Crotalaria breviflora, Crotalaria spectabilis, stylo, jack bean and dwarf pigeon pea. No intercropping increased the system's yield compared to sole-crop rice, but intercropping of rice with forage peanut and stylo promoted grain yield and quality similar to those of sole-crop rice. Intercropping with C. breviflora affected the agronomic and qualitative performance of rice. Common bean yield after rice intercropped with dwarf pigeon pea, C. spectabilis and C. breviflora was similar in yield after sole-crop rice, while the other intercrops reduced common bean yield. Common bean grain quality was not affected by the cultivation of rice as sole crop and intercropped with green manures. Although none of the intercropping systems increased yield compared to sole-crop rice (control), it can be concluded that the intercropping of upland rice is viable depending on the green manure species, allowing greater biomass production per area that can help long-term soil conservation and increase the system's yield.
Technical Efficiency and Technological Gaps of Rice Production in Anambra State, Nigeria
The traditional approach to modeling productive efficiency assumes that technology is constant across the sample. However, farms in different regions may face different production opportunities, and the technologies they employ may differ due to environmental factors. Therefore, rather than using a traditional stochastic frontier model in such cases, a stochastic meta-frontier (SMF) analysis is recommended to account for environmental factors between regions. It follows that differences in environmental factors between the upland and lowland regions in Anambra State, Nigeria, may result in farmers producing rice under different production and environmental conditions. Using the SMF model, this study, for the first time, determines technical efficiency (TE) and technological gap ratios (TGRs) of rice production from the upland and lowland regions in the Awka North Local Government Area of Anambra State, Nigeria. Our data are from a cross-section sample of randomly selected rice farmers. Results reveal that lowland regional rice producers are on average, significantly more technically efficient (91.7%) than their upland counterparts (84.2%). Additionally, mean TGRs associated with lowland rice farmers are higher (92.1%) than their corresponding upland producers (84.7%). While the upland rice producers are less technically efficient and further away from their full potential, results indicate that both sets of farmers do not use advanced technologies to match the industry’s potential. We suggest that agricultural policy should focus on providing regionally specific technologies, such as improved rice varieties that fit the working environment of the lagging area, to help rice farmers improve their resource efficiency and minimize technological gaps.
Agronomic Response of Local Upland Rice Cultivars on Growing under Two Cultivation Systems
Background: This study aimed to observe the agronomic response of 18 Southeast Sulawesi local upland rice cultivars that were grown under two cultivation systems (dry land and wet rice field) and optimize local potential to support self-sufficiency and food security. Methods: The research used a split-plot design with the following main plot: cultivation system (L) including upland (L1) and rice field cultivation system (L2). The subplots were 18 local upland rice cultivars such as Wangkomina (K1), Wuna Lapodidi (K2), Waburi-buri (K3), Wapantoga (K4), Nggalaru (K5), Wuna Parigi (K6), Bakala (K7), Biu (K8), Ikulaku (K9), Bou (K1), Momea (K11), Daindomoronene (K12), Konkep (K13), Tinangge (K14), Ndoamoito (K15), Uwa (K16), Ndowatu (K17) and Indalibana (K18). Result: The local upland rice responded better to the wetland cultivation system than the upland cultivation system. The local upland rice cultivar Ndowatu showed the highest production potential, which was statistically similar to the Biu, Ikulaku, Momea, Konkep and Uwa cultivars. Ndowatu cultivar showed high production potential (842.80 g.m-2). Thus, this cultivar can be considered suitable for development in the rainfed lowlands to increase the planting index and to support the self-sufficiency and food security of the region.
Effect of media size and dosage of NPK fertilizer on growth and yield of upland rice lines in polybags
Export citation format, share document.
- Research article
- Open access
- Published: 11 June 2014
A genomic perspective on the important genetic mechanisms of upland adaptation of rice
- Jun Lyu 1 ,
- Baoye Li 2 ,
- Weiming He 3 ,
- Shilai Zhang 2 ,
- Zhiheng Gou 1 ,
- Jing Zhang 2 ,
- Liyun Meng 4 ,
- Dayun Tao 2 ,
- Wangqi Huang 2 ,
- Fengyi Hu 2 &
- Wen Wang 1
BMC Plant Biology volume 14 , Article number: 160 ( 2014 ) Cite this article
4926 Accesses
29 Citations
1 Altmetric
Metrics details
Cultivated rice consists of two important ecotypes, upland and irrigated, that have respectively adapted to either dry land or irrigated cultivation. Upland rice, widely adopted in rainfed upland areas in virtue of its little water requirement, contains abundant untapped genetic resources, such as genes for drought adaptation. With water shortage exacerbated and population expanding, the need for breeding crop varieties with drought adaptation becomes more and more urgent. However, a previous oversight in upland rice research reveals little information regarding its genetic mechanisms for upland adaption, greatly hindering progress in harnessing its genetic resources for breeding and cultivation.
In this study, we selected 84 upland and 82 irrigated accessions from all over the world, phenotyped them under both irrigated and dry land environments, and investigated the phylogenetic relations and population structure of the upland ecotype using whole genome variation data. Further comparative analysis yields a list of differentiated genes that may account for the phenotypic and physiological differences between upland and irrigated rice.
Conclusions
This study represents the first genomic investigation in a large sample of upland rice, providing valuable gene list for understanding upland rice adaptation, especially drought-related adaptation, and its subsequent utilization in modern agriculture.
Asian cultivated rice ( Oryza sativa type indica and Oryza sativa . type japonica) has phenotypically divergent ecotypes [ 1 ]. Irrigated and upland rice, respectively adapted to growing in paddy fields and rain-fed dry lands, are two main ecotypes that are generally considered to show apparent phenotypic differentiation. The upland type is more drought tolerant, taller, of lower tillering potential, more resistant to blast, and tends to have longer and thicker roots than its irrigated counterpart [ 2 , 3 ]. Accordingly, upland varieties are widely adopted in upland areas to optimize crop yields because of their more efficient water usage and adaptation to dry land. Though the yield of upland rice is comparatively lower than the irrigated rice, it provides the daily staple food on which nearly one hundred million people depends [ 4 ]. Because we are now facing global population expansion and a growing shortage of water resources, upland rice and especially knowledge about its genetic mechanisms for dry land adaptation become more important as they allow the possibility of transforming or modifying high-yield irrigated varieties of rice to upland ones.
Drought resistance is an important component of rice adaption to most rainfed upland areas because in this ecosystem water could not be well preserved and frequent or sporadic drought events could occur each year [ 5 ]. Previous research proposed three different mechanisms for plant drought resistance: drought escape, dehydration avoidance, and dehydration tolerance [ 6 ]. Through drought escape, timing of plant lifecycle is modulated to escape the time of drought when drought time is predictable in each year. Dehydration avoidance refers to the case that plants constitutively avoid excess loss of water through more developed root systems or a thick layer of cutin [ 6 ]. Dehydration tolerance refers to the inducible mechanisms, like osmotic adjustment, which help plants to prevent damage and survive when excess loss of water does happen [ 6 ]. For the frequent and unpredictable drought stress in upland areas, the dehydration avoidance and dehydration tolerance should be the appropriate options for upland crops. In fact, upland rice seems to depend more on the constitutive dehydration avoidance than the inducible dehydration tolerance in its adaptation [ 7 – 9 ]. By far, some genes have been reported to be related with drought resistance[ 10 ], such as genes in abscisic acid (ABA) synthetic pathway (such as 9- cis -epoxycarotenoid dioxygenase genes) [ 11 , 12 ], transcription factors interacting with the cis-acting dehydration response element (DRE) [ 13 ], genes synthesizing osmoprotectants [ 14 , 15 ], and mitogen-activated protein kinase (MAPK) genes [ 16 ]. However, these studies focused on single gene’s effect, while adaptation to dry land may involve many genes in the genome. Furthermore, the valuable genetic resources within upland rice, which were ignored by most previous studies, are also worthy of enhanced utilization.
Upland and irrigated rice evolve their respective characteristic traits under both natural and artificial selections, which lead to phenotypic adaptation to respective environment and simultaneously genetic differentiation between ecotypes. Some statistical tools for identifying selected regions during population differentiation have been well established. Fixation index ( F ST ) is a commonly used statistics that measure population differentiation based on genetic polymorphism data. F ST is positively associated with allele frequency difference. Cross-population composite likelihood ratio test (XP-CLR), a newly invented approach, incorporates not only genetic differentiation but also allele frequency spectrum information [ 17 ]. By jointly modeling multilocus allele frequency differentiation and frequency spectrum under neutrality and selection, XP-CLR was demonstrated to have high power in detecting selective sweeps during population differentiation. Besides, Nielsen’s CLR, a parametric test also based on composite likelihood ratio, detects selective sweeps using the spatial distribution of site frequency spectrum [ 18 ]. This approach only uses SNP data of one population and thus detects selective sweeps in single population without consideration of population differentiation.
To investigate the genetic mechanisms for upland adaptation and identify genes for its important component, ‘drought resistance’, we use our whole-genome re-sequencing data for a large panel of accessions, including 84 upland accessions and 82 irrigated accessions reported by our previous work [ 19 ] , where these data were used to test that a low-diversity region containing a key enzyme gene of abscisic acid synthesis was due to human selection. Considering the wide distribution of upland rice in the world, we obtained our samples from Asia, Africa and South America, covering most major distribution areas of upland rice. We explored phylogenetic relationship between upland and irrigated ecotypes and population structure of current upland rice population. More importantly, by detecting selective sweep regions using F ST and XP-CLR, we compiled a list of candidate genes that are likely responsible for upland adaptation or that are related to phenotypic differentiation between the upland and irrigated ecotypes, providing valuable insights about crop breeding for dry land.
Phenotypes of the upland accessions differ from those of the irrigated ones
For the 84 globally sampled and representative upland rice accessions (see Additional files 1 and 2 ), we want to confirm whether they possess the reported phenotypes that distinguish them from irrigated rice [ 2 , 3 ]. Therefore we grew the varieties to do phenotyping (see Additional files 3 and 4 ). Our examination focused on four non-yield phenotypes that are reported to be differentiated between upland and irrigated rice: root weight, maximal main root length, tiller number, and plant height. Moreover, we also examined four yield traits, including thousand grain (1000-grain) weight, number of productive panicles, number of filled grain per panicle and number of empty grain per panicle. Under both well-watering and dry land (rainfed upland) environments, the upland accessions consistently showed significantly taller plant architecture, fewer tillers, longer main roots and heavier root weights, which supports the previously reported phenotypic differences (Table 1 , see Additional file 5 for the phenotypic difference). Regarding the yield traits, under both environments upland accessions show larger 1000-grain weight and less productive panicles as compared to irrigated accessions (Table 1 ). The numbers of filled and empty grain per panicle show no significant differences between the two ecotypes under the irrigated condition. However, under the rainfed upland condition, the upland accessions perform much better, i.e. have significantly more filled grain (Student’s t-test, P = 0.016) and less empty grain (Student’s t-test, P = 5.3e-05) per panicle than the irrigated accessions.
Using software SPSS statistics 17.0, we calculated the pearson correlation coefficients (ρ) between each of the four non-yield traits and each of the yield traits under upland condition (Additional file 6 ). The plant height is significantly associated with the 1000-grain weight (ρ = 0.263, P = 0.002), the number of empty grain per panicle (ρ = −0.226, P = 0.009) and the number of productive panicles (ρ = −0.2, P = 0.022). The tiller number is significantly associated with the number of productive panicles (ρ ≈ 1, P < 0.001) and the 1000-grain weight (ρ = −0.169, P = 0.05). The root weight is significantly associated with the number of filled grain per panicle (ρ = 0.295, P = 0.001). The maximal root length is significantly associated with the thousand grain weight (ρ = 0.443, P < 0.001) and the number of empty grain per panicle (ρ = −0.232, P = 0.008). Therefore all of the four non-yield traits are significantly associated with yield traits under rainfed upland condition. From the ρ values it could be speculated that larger root weight and greater root length of upland rice would contribute to net yield increase under upland condition probably because better developed root systems could contribute to better dehydration avoidance. However, the effects of plant height and tiller number on yield are complex. The larger plant height of upland accessions would possibly cause the reduction of productive panicles, but can increase 1000-grain weight and reduce the number of empty grain. The less tiller numbers of upland accessions can cause reduction of productive panicles, but would increase 1000-grain weight.
When comparing these phenotypes between the two culture conditions, we found that dry land environment can slightly reduce plant height and 1000-grain weight, but will dramatically decrease the tiller number, root weight, number of productive panicles and number of filled grain per panicle of both the upland and the irrigated ecotypes. However, the main root length and the number of empty grain per panicle responded differently to the different environments between the two ecotypes. We observed a minor decrease (−2.5%) of main root length of irrigated rice but a significant increase (+9.9%) of upland rice root length in dry land as compared with that in the irrigated condition (Table 1 , Student’s t-test, P = 0.002, Additional file 7 ), indicating an adaptive feature of upland rice. Specifically, 61 percent of the upland accessions showed increase of root length in the upland condition, while only about 38 percent of the irrigated accessions showed root length increase in the upland condition. Moreover, for the number of empty grain per panicle, we observed a slight and nonsignificant reduction of it for the irrigated accessions in the upland condition (Student’s t-test, P = 0.29, Additional file 7 ) but a dramatic decrease of it for the upland accessions (Student’s t-test, P = 5.9e-07, Additional file 7 ), suggesting another adaptive trait of upland rice. Based on the phenotypic differentiation between the upland and irrigated accessions and the correlations between the non-yield phenotypes and yield phenotypes in the upland condition, we define ‘upland adaptation’ in this study as the characteristic non-yield phenotypes of upland rice that are associated with the yield performance in upland conditions, such as plant height, tiller number, root weight and maximal root length which are directly associated with drought resistance.
SNPs calling and annotation for the 166 accessions
For the 82 irrigated and 84 upland accessions, we obtained 402,052,644 pair-end reads that passed the reads Pass Filter, amounting to around 8 G base pairs (NCBI accession code SRA066116). The re-sequencing data for 84 upland and 82 irrigated accessions was reported in our previous work [ 19 ]. Here we comprehensively analyze these re-sequencing data to determine the genetic components that might have caused the upland adaptation and phenotypic differentiation between the two ecotypes, which has been a long-term open question in rice study.
About 87 percent of these reads could be aligned to the reference genome (IRGSP/RAP build 5 Nipponbare genome) using SOAP-2.20 software [ 20 ]. The average mapping ratios of reads for the type indica and type japonica are 81% and 92%, respectively. On average, 29.54% of the reference genome was covered for each accession (ranging from 18.65% to 46.58%). The mean depth for each accession was about 0.50 × on average (ranging from 0.27 × to 0.96 ×) (see Additional file 8 ). In total, the genome coverage of the upland and irrigated population reached 41 × and 43 ×, respectively. After SNP calling and a series of filtering for quality control (see Methods), 3,571,104 high-quality SNPs were identified (Additional file 9 includes all the SNPs), of which 3,563,681 are non-singleton SNPs. By randomly choosing 56 SNPs and genotyping them via Sanger sequencing, we were able to validate our SNP calling accuracy at 96.4%. The sequencing data, though relatively low in depth, are enough to well satisfy our analyses, as we shall discuss below.
In the following analyses, only 3,029,822 SNPs with genotype data covering more than 10 individuals in both populations were used. We annotated these SNPs by classifying them into different categories according to the genomic context (see Additional file 10 ). The non-synonymous-to-synonymous ratio of SNPs is 1.32, consistent with the previously reported ratio of 1.29 in rice [ 21 ]. SNPs causing radical mutations, such as premature stop codons or frame disruptions, were also identified (Additional file 10 ).
Phylogenetics and population genetics analysis for upland rice
SNP genotypes were used in phylogenetic analysis for the 166 accessions. Additionally, 25 wild accessions from our previous data (10 O. nivara and 15 O. rufipogon , Additional file 11 ) were added to help address the phylogenetics of upland accessions [ 21 ]. We constructed the accessions’ phylogenetic tree using a Neighbor-Joining approach with 100 bootstraps (Figure 1 ). Consistent with some of the previous reports [ 21 ], our results support that Indica and Japonica usually considered as two subspecies are very different groups, as they largely clustered in respective group and both have closely related wild accessions (Figure 1 , also see Additional file 12 ). The results also show that probably the majority of current upland accessions belong to the type japonica (in our sample 76% upland accessions are japonica) (Figure 1 , Additional file 12 ), consistent with the classification of the collection center based on traditional phenotypic characteristics and previous reports that upland rice mainly pertains to Japonica [ 22 ]. The phylogenetic tree showed that all the upland japonica accessions cluster together, suggesting that upland japonica likely have a single origin. However, for the type indica, the scattering pattern of upland indica indicates that upland indica accessions may have been separately bred multiple times using different irrigated rice as the basic genetic materials.
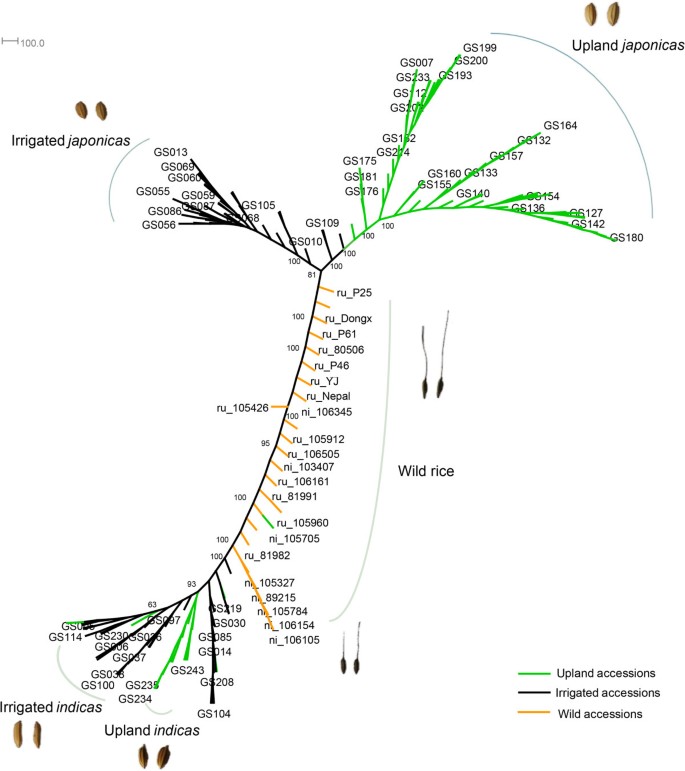
Phylogenetic tree of rice accessions. Green, black and orange branches refer to upland, irrigated and wild accessions respectively. Analysis showed that differentiation between Indica and Japonica has existed within the wild population, since there are strains of wild rice close to both Indica and Japonica respectively, supporting the double domestication model. The tree shows multiple origins for upland rice, though the upland japonicas may bear a single origin. Bootstrap values are indicated in some of the major internal nodes. Some of the leaf nodes are labeled with the sample number of the rice accessions. ‘ru’ refers to ‘ rufipogon ’, and ‘ni’ refers to ‘ nivara ’.
Population structure in upland rice and admixture in upland indica
We use 24,890 SNPs with genotype data covering more than 100 accessions to analyze the population structure within our samples (see Methods). Principle component analysis (PCA) summarizes the population structure for the entire population (Figure 2 a, b). The top four principle components explain about 65% of the genetic variation. The huge differentiation on principle component 1 distinguishes Indica from Japonica. By contrast, genetic differentiations between upland and irrigated ecotypes seem to be much lower. The F ST value for the two ecotypes calculated based on genome-wide SNPs is only 0.06. Even in the type japonica, where the upland accessions approximate monophyly and the ecotype differentiation is clear-cut (Figure 1 , Figure 2 a), the upland and irrigated ecotypes still have a limited genetic differentiation ( F ST = 0.13), much lower than the reported differentiation between type indica and type japonica ( F ST = 0.55) [ 23 ].
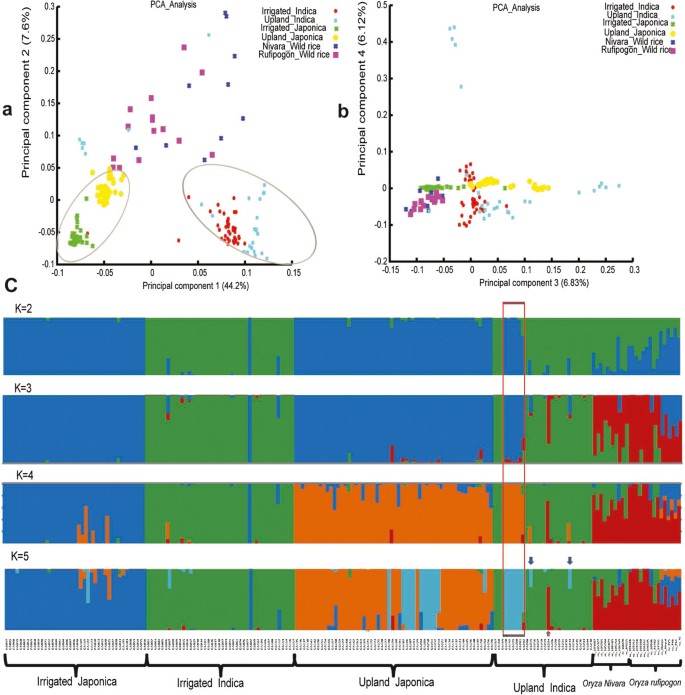
Population structure estimation. PCA analysis was conducted with the top four components shown in (a) and (b) . The four components together explain about 65% of the genetic variance. (a) , Principal component 1 distinguishes the type japonica from the type indica. Both types include two ecotypes (upland and irrigated ones). The ecotype differentiation in Japonica is clear-cut. (b) , principal components 3 and 4. (c) , the population structure reported by FRAPPE. Results were shown from K = 2 to K = 5. When K = 2, there is only division of Indica and Japonica. Then wild rice is separated when K = 3. When K = 4, the ecotype differentiation in Japonica emerges, i.e. upland japonica begins to differ from irrigated japonica. Our results show that upland-irrigated ecotype differentiation is genetically lower than the Indica-Japonica differentiation. The red rectangle indicates the six upland accessions, which were previously classified as upland indica accessions but are genetically more close to the upland japonica group. The blue arrows in K = 5 panel indicates the two upland indica, GS215 and GS233, which have a large genomic proportion close to upland japonica. The red arrow indicates upland indica, GS224, which is close to wild rice.
We also investigated the population structure using FRAPPE to estimate the ancestry and population admixture of each accessions [ 24 ]. By increasing K (the number of populations) from 2 to 5, the population structure of upland and irrigated accessions are shown (Figure 2 c). For K = 2, we see a differentiation between type indica and type japonica, and this differentiation also exists for Oryza rufipogon and Oryza nivara in the wild population. When K = 3, the wild rice and the cultivars become separated. When K = 4, the division between irrigated japonica and upland japonica emerge, while the irrigated indica and upland indica still share the same structure, indicating the ecotype differentiation is higher within the type japonica than in the type indica. When K = 5, a new subgroup in upland japonica appears, suggesting some accessions in upland japonica forms a unique subgroup which is differentiated from the others in it.
From the structure analysis, we found that some upland indica accessions, though primarily classified as type indica according to their phenotypes, turn out to be quite complicated in terms of their genomic composition. For example, six accessions (GS190, GS192, GS199, GS200, GS201, GS202) (enclosed in the red rectangular in Figure 2 c), previously classified as upland indica according to their phenotypes (e.g., the seed shape, see Additional file 13 ), turn out to be more close to upland japonica (Figure 2 c), indicating that for the upland ecotype, seed shape phenotype could be misleading in distinguishing type indica from type japonica. Besides the six accessions, GS215 and GS233 also have a large genomic proportion close to upland japonica(indicated with blue arrow in Figure 2 c), which makes them cluster with japonica in the phylogenetic tree (Figure 1 , Additional file 12 ). And upland indica GS224 (Dular), indicated with a red arrow, has a large proportion of wild rice ancestry, and correspondingly it clusters with the wild rice in the phylogenetic tree (Figure 1 , Additional file 12 ). These results suggest that current upland indica group is a admixture, containing accessions of different origins. They are classified as belong to indica probably because some of their traits are more close to type indica than type japonica. Besides, the 19 upland indica accessions which remain in the indica group in the tree also seem to be polyphyletic, indicating they might originate multiple times and their genetic mechanisms for upland adaptation might be heterogenous. On the contrary, upland japonica accessions that form a monophyletic group are more likely to have similar mechanisms for upland adaption. Considering the well-known hybridization between indica and japonica, it’s probable that upland japonica and some upland indica share similar adaptation genes, while the rest upland indica might be different. Therefore, in the following study on the ecotype differentiated genes, we first use a large sample size by comparing the merged upland and irrigated population to identify genes that show strongest signals and might be shared between upland japonica and some upland indica accessions. Then we mainly focus on the type japonica to find the japonica-specific upland adaptation genes. More data and other experiments would be needed to comprehensively address the adaptation mechanism in the complicated indica group.
Ecotype differentiated genomic regions (EDRs) in genomes
EDRs probably contain genes that account for the adaptive differentiation. Using a sliding window approach to screen regions with both the top 5‰ F ST and cross-population composite likelihood ratio test (XP-CLR) scores (Methods), EDRs were identified with selective signals to narrow down the genes underlying the phenotypic differentiation between upland and irrigated rice (Figure 3 ). After merging the neighboring EDRs, we eventually identified 74 EDRs in the genome with a median length of 22.7 kb that were differentiated between the upland and irrigated rice (Additional file 14 ). Within these EDR regions, there are a total of 8980 SNPs (Additional file 15 ). Compared with the whole genome SNPs used in our analysis, the SNPs in EDRs are significantly enriched in genic regions (Chi-square test, P < 2.2e-16) (see Additional file 16 ). Among the EDR SNPs, 2623 have allele frequency differences larger than 60% between ecotypes, which we subsequently labeled “ecotype differentiated SNPs” (EDSs) (see Additional file 17 ).
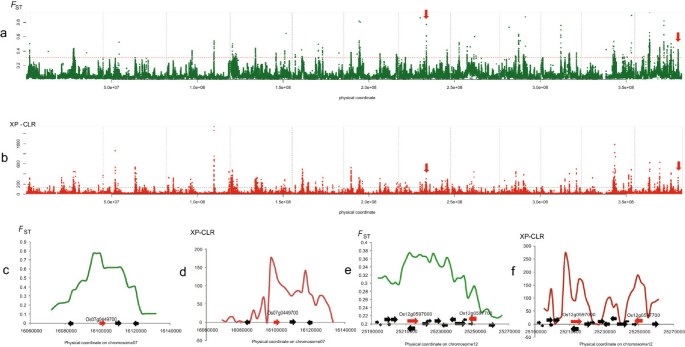
EDRs scanning. (a) , F ST values were plotted against physical coordinates over the genome; the red horizontal dashed line refers to the top 5‰ threshold. (b) , XP-CLR scores were plotted against genomic coordinates; the green horizontal dashed line refers to the top 5‰ threshold. EDRs were obtained by taking the intersection of the EDRs given by the two approaches. The vertical dashed lines in (a) and (b) indicate the boundaries between two chromosomes; the vertical red arrows indicate the interesting genes shown in detail in (c - f). (c) and (d) respectively show the F ST and XP-CLR values around Os07g0449700 , an interesting gene annotated as a type A response regulator (indicated using a red arrow). (e) and (f ) respectively show the F ST and XP-CLR around Os12g0597700 , which is similar to WRKY2 and Os12g0597000 encoding a SOS3-like calcium-binding protein 1 (indicated with red arrows). All of these interesting genes have strong F ST and XP-CLR values and are biologically related to upland adaptation in rice.
Ecotype differentiated genes (EDGs) in EDRs
There are 154 genes in the EDRs, which we called EDGs (see Additional file 18 ). For these EDGs, 74 genes have EDSs in the gene regions and the other 53 genes have EDSs located within 1 kb upstream or downstream regions. By gene enrichment analysis, we found that the EDGs are enriched in several classes (Table 2 , Additional file 19 ), such as lipase containing GDSL domain class ( P = 7.49e-05), peroxidases ( P = 1.80e-03), glutathione-related classes ( P = 5.44e-08), and auxin signaling pathway ( P = 3.80e-06).
The GDSL domain-containing lipase has been reported to be involved in response to abiotic stress, such as drought [ 25 – 27 ], indicating that the genetic differentiation of these genes in upland rice may be relevant to their adaption to dry land. Peroxidases and glutathione-related proteins bear antioxidant activity, another crucial factor in plant adaptation to a drought-like environment [ 6 , 7 ]. Furthermore, auxin, the famous growth-promoting hormone, plays an important role in determining plant architecture, and has been proved to control the formation of adventitious roots [ 8 , 28 – 30 ]. Accordingly, differences in auxin signaling between upland and irrigated rice could potentially contribute to their different architectures and root systems. Additionally, we also found several individual EDGs of interest that might also be critical in upland adaptation or the phenotypic differentiation between the two ecotypes. Os07g0449700 , a type-A response regulator, is of special interest (Figure 3 c, d). Type-A response regulators belong to ARR gene family induced by cytokinin and play important roles in cytokinin signaling [ 31 ]. Quite a few pieces of evidence have shown that ARR genes impact root and shoot development and apparent functional overlap exists among type-A ARRs [ 31 – 34 ]. Moreover, the relevance of type-A ARR gene to the root and shoot phenotypes that are differentiated between upland and irrigated rice has also received experimental support; over-expression of a type-A response regulator in transgenic rice has been shown to cause dwarfness and poorly developed root systems [ 32 ]. Hence the ecotype differentiation on this type-A ARR gene, Os07g0449700 , may be able to explain certain differences of root and plant height phenotypes between upland and irrigated rice. Further reciprocal transgenic experiments between upland and irrigated rice will eventually address the contribution of Os07g0449700 in the adaptation of upland rice.
Other EDGs were also implied by direct or indirect evidence to be relevant to upland adaptation. Os12g0597700 (Figure 3 e, f) has a WRKY DNA-binding domain and WRKY genes have been well established to be important elements in drought tolerance [ 35 – 38 ]. Os12g0597000 encodes a SOS3-like calcium-binding protein 1 and its homologs in Arabidopsis are related to responses to water deprivation [ 39 ]. Os11g0474600 encodes a terpenoid synthase, and terpenoid plant hormones have been shown to inhibit rice tillering [ 40 ]. This gene may, therefore, explain the difference in tiller numbers between upland and irrigated rice. Besides, several pentatricopeptide repeat protein-coding genes ( Os01g0646000 , Os06g0184866 , Os06g0185800 ) and two serine/threonine protein kinase genes ( Os04g0616400 , Os04g0616600 ) may also be related to drought response as these classes of genes have been reported to be involved in responses to drought/osmotic stress and root development [ 38 , 41 ]. These results illustrate how these 154 EDGs may represent a valuable candidate gene list for investigating the phenotypic differentiation of the two ecotypes and upland rice adaptation.
EDGs selected in individual populations
The investigated EDGs indicate selection during the differentiation of the two ecotypes. To further pinpoint in which population the selection occurred, we used Nielsen’s CLR test to identify possible selective sweeps in a single population (see Methods). We identified 661 selective sweep regions in the upland population with a median length of 27 kb, and 351 selective sweep regions in the irrigated population with a median length of 41 kb (Additional file 20 ). In the selected regions of these upland and irrigated populations, there are 2542 and 1285 genes, respectively. Upland rice then has twice as many genomic regions/genes selected as irrigated rice, indicating that upland rice varieties probably suffered more selection pressure imposed by adaptation or breeding. By overlapping the 154 EDGs with these potentially selected genes, we found that almost all of these selected EDGs (150/154) could be reconfirmed as selected genes using Nielsen’s CLR approach. Among these, 60 were potentially specifically selected in upland rice, 19 were potentially specifically selected in the irrigated population, while 71 were likely selected in both populations (Figure 4 , Additional file 18 ).
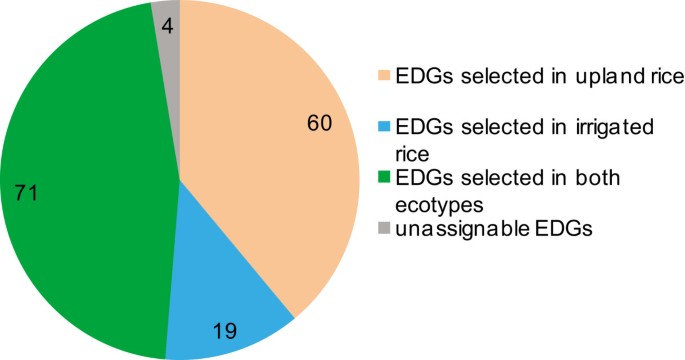
EDGs population assignment. Using CLR, 154 EDGs were assigned to specific populations. In total, 60 genes were selected in upland rice, 19 genes in irrigated rice, and 71 genes were selected as probably being in both populations, while four were not able to be assigned to a specific population.
Japonica-specific and Indica-specific EDGs
Genetic architectures of complex traits in rice are often both rich and complicated since they are usually heterogeneous across the subspecies [ 42 ]. In light of this, we were also interested in the ecotype-differentiated genes within the type indica or type japonica. As Figure 1 depicts, upland indica have multiple origins, and thus may be genetically heterogeneous in terms of upland adaptation. When investigating the type-specific EDRs, we accordingly mainly focused on the type japonica.
We carried out a similar pipeline to screen the EDRs in type japonica (Additional file 21 ) and found 52 EDRs with a median length of 24 kb (see Additional file 22 ). In these EDRs, 169 EDGs were identified (see Additional file 23 ), among which 47 EDGs are overlapped with the previous EDG list from the total population while the remaining 122 are japonica-specific. For these japonica-specific EDGs, the significantly enriched gene families include mitogen-activated protein kinase ( P = 1.37e-14), WRKY genes ( P = 0.03) and thioredoxin coding genes ( P = 0.04) (Table 2 ). Previous studies showed that mitogen-activated protein kinase plays an important role in response to biotic stress and root development [ 11 , 43 ]; one japonica-specific EDG of this class is very similar to blast induced kinase, which may then explain why upland rice are generally more blast tolerant than irrigated rice. Moreover, as mentioned previously, WRKY genes and thioredoxin may be critical for drought response. Intriguingly, among our list we found Os09g0410500 , a homolog to the famous maize-domestication gene, teosinte-branched 1( tb1 ), indicating this gene may also be related to the different tillering abilities displayed by the upland and irrigated types.
For the type indica, because of the admixture of upland indica only 19 upland indica accessions that remain to cluster in the indica group could be used for analysis. But the F ST between upland and irrigated accessions in indica is quite lower (0.06) than that in japonica (0.13), which means that a much larger sample would be needed to detect the ecotype differentiation in indica. However, because of the small population size of upland indica accessions in the world it is difficult to collect enough samples so far. Analysis using only the 19 upland indica accessions yield a list of 56 genes identified by intersecting F ST and XP-CLR top signals (Additional file 24 , Additional file 25 ). Hence, it is not surprising that none of the 56 genes were overlapped with the previous EDG list from the total population, considering the small sample size and possibly heterogeneous adaptation mechanisms in indica. However, we noticed that nine and four of the genes from the indica XP-CLR top signals overlap with the previous EDGs of the merged populations and EDGs of the japonica population, respectively (Additional file 26 , Additional file 27 ), indicating that some genes related to upland ecotype might be shared among some indica accessions and japonica but are more difficult to detect because of the limited and probable heterogenous samples. More experiments and data are needed to comprehensively address the indica-specific upland adaptation genes.
Largely due to the popularity and fecundity of irrigated rice, there has been limited inquiry about the phenotypes that distinguish upland rice. Some work in this area has been done by Chang et al., who investigated the rate of leaf production, tillering production, plant height, root system and resistance to drought for 11 upland varieties as explanatory factors in the different phenotypes [ 3 ]. Likewise, Ono pointed out that Japanese upland varieties are tall and low tillering with broad leaves, long panicles, drought and blast resistant, and have a less efficient utilization of fertilizer [ 44 ]. Despite interesting findings, these studies were based on only a few varieties of upland rice. To our knowledge, the sample size of the phenotypic comparisons between upland and irrigated rice in this study represents a very large sample of upland varieties to date and the sample covers most distribution regions of upland rice over the world, providing solid evidence supporting Chang et. al’s observations [ 3 ]. Our phenotyping also observed some new features, e.g., the phenomenon that dry land culture increases main root length of upland rice but decreases that of irrigated rice indicates a remarkable superiority of upland rice to adapt to an upland environment.
Beyond our thorough phenotypic analysis, this study, to our knowledge, also provides the first genomic perspective as to the phylogenetics and population structure of current upland rice. Consistent with our classification based on phenotypic characteristics and Glaszmann’s report which indicated most upland cultivars belong to the type japonica [ 22 ], phylogenetic analysis revealed that a large proportion of upland accessions belong to the type japonica, which form a monophyly. The population structure inference reveals much less genetic differentiation between the two ecotypes than exists between the type indica-japonica, which could be due to either much more frequent gene flow between ecotypes than that between Indica and Japonica, or recent origin of upland rice.
Though the sequencing depth for each accession used in our study is relatively low, the data set itself is informative enough to address the questions we wanted to address about upland rice adaptation. Firstly, the SNPs identified have a high accuracy (96.4%), even taking into account the low coverage. Secondly, the average sequencing depth for each site in the upland or irrigated population is more than 40×, providing a sufficient sample size for allele frequency estimation. Likewise, only SNP sites with the genotype determined in more than 10 individuals within each population were used for analysis such as calculating allele frequencies, and only SNP sites with no less than 100 individuals’ genotypes were used for estimating population structure. Thirdly, though the genome coverage rate for each accession is not high, for each pair-wise comparison there are enough informative sites for the distance between any two accessions to be precisely computed (see Additional file 28 ). Thus, as some previous studies have shown, we presented an interesting example substantiating the feasibility of genetic analysis with low coverage population genomic data [ 45 , 46 ].
Except for some QTL mapping efforts, very few reports have touched upon the genetic mechanisms that underlie the adaptive phenotypes of upland rice [ 47 , 48 ]. In this study, we identified some interesting candidate genes or gene families that likely contribute to the upland adaptive traits or phenotypic differentiation between the upland and irrigated ecotypes, not only providing exciting new evidence useful in harnessing the genetic adaptations of upland rice but also demonstrating the efficiency of our methodology. Though pending more experimental evidence to validate the precise phenotypic effects of all the identified alleles, a limited number of genes (around 150) provided a highly feasible list for functional investigation as well as for the upland breeding. Crucially, we would like to stress that our approach most likely caught most of the major genomic regions that account for ecotype differentiation and upland rice adaptation including those specific regions in type japonica, but identifying some indica accession specific adaptation genes still need further work and additional data.
In summary, we surveyed the phenotypes across a large sample of upland rice in both dry land and irrigated conditions, and by comparing them to irrigated rice in similar conditions we were able to demonstrate some significant phenotypic differentiations between the two ecotypes. Using high-throughput re-sequencing of a large batch of rice accessions, for the first time, to our knowledge, we clarified a single-origin pattern of upland japonica rice but a novel multiple origin pattern in upland indica accessions. With comprehensive population genetic analysis, we identified the ecotype differentiated regions and important selection genes during the differentiation between the upland and irrigated ecotypes, and more poignantly, provided great insights into upland rice adaption and the phenotypic differentiation between the two ecotypes. These findings have important implications in the spread of upland rice varieties in breeding. For example, the genes that likely account for drought resistance can be used to breed highly drought resistant varieties from the existing high-yield irrigated varieties, and concurrently, the genes likely related to tillering ability can be used to enhance the tillering ability of upland rice, a major factor limiting its yield and subsequently its widespread usage.
Investigating phenotypes of upland accessions
To assess the reported phenotypes of upland and irrigated rice, we grew both varieties. Due to the limited amount of seeds for some accessions, only 131 accessions were phenotyped (Additional file 3 ). Phenotyping was performed both in irrigated and dry land conditions (i.e., preventing submergence in water to simulate the rainfed upland environment). For the irrigated condition, seeds were germinated and seedlings then transplanted to a paddy field with submergence in water for the whole growth and development stage. For the rainfed upland condition, we conducted direct-seeding. To fully simulate the rainfed condition, no irrigation was conducted for the whole growth stage. When the rain came, we released the water to prevent submergence. According to our records, several moderate drought events occurred and caused a few individual plants death in the upland condition. For each accession, we planted 12 individuals in two rows (6 individuals in each row) with the row spacing of 30 centimeters and plant spacing of 20 centimeters. Before the earing period, we counted the tillering numbers. After the plants entered maturity, we surveyed the root weight, main root length, plant height and the yield traits. For each accession, about five individuals were randomly selected and phenotyped (Additional file 4 ). Because in the rainfed upland condition the percentage of earbearing tiller is close to 100%, in this study tiller number and productive panicle number in the upland condition share the same result.
The re-sequencing data
The re-sequencing data for the 84 upland and 82 irrigated accessions was reported by our previous work [ 19 ]. In our previous study, these data were simply used to test that a selected region containing a key enzyme gene of ABA synthesis was not due to demographic reason. They have neither been used to investigate the population structure for upland rice, nor been used to study the population differentiated regions/genes between the two ecotypes. Here we use these re-sequencing data to further exploit the genetic components that underlie the upland adaptation and phenotypic differentiation between the two ecotypes. The 84 upland and 82 irrigated accessions were obtained from the Yunnan Academy of Agricultural Sciences (YAAS), Yunnan, China. The ecotype information was documented by the genetic resources center of YAAS. Both indica and japonica were selected for either the irrigated or upland groups (Additional file 2 ). For the irrigated ecotype, we included 42 indica accessions and 40 japonica accessions. For the upland ecotype, we selected 28 indica and 56 japonica accessions since in our genetic resources center most upland rice accessions belong to the type japonica (Additional file 2 ). The origins of the accessions cover a wide geographic range (Additional file 1 ) including most major regions of upland rice distribution (South Asia, Southeast Asia, South America, West Africa, etc.). All seeds were sown under irrigated conditions. When the seedlings turned to trefoil-stage, genomic DNA was extracted from the leaves using the CTAB method [ 49 ].
Reads mapping
Our previously obtained re-sequencing data of these upland and irrigated accessions were deposited in the NCBI Short Read Archive under the accession number SRA066116 [ 19 ]. The raw reads of the wild rice came from our previous work (NCBI Short Read Archive accession number SRA023116)[ 21 ]. The IRGSP 5.0 Nipponbare genome was downloaded from the RAP-DB database ( http://rapdblegacy.dna.affrc.go.jp/download/latest/IRGSPb5.fa.masked.gz ). We mapped the short reads to the Nipponbare genome using SOAP for each accession separately [ 20 ].
Calling SNPs and SNP annotation
After mapping the reads, we used SOAPsnp -v1.02 to call SNPs for the whole population of the irrigated and upland accessions [ 50 ]. A series of filtering was conducted to ensure the quality of the SNPs. For example, the SNPs with a quality value less than 15 or with a nearby copy number > 1.5 were removed. Also we eliminated SNPs sites with depths less than 6 or larger than 300 because sites with too low a depth are prone to sequencing errors while sites with too high depths are likely located within repeat or duplication regions [ 51 ]. In order to confirm the accuracy of SNP calling, we randomly selected 60 SNPs, amplified the sequences around them with PCR and subjected them to Sanger sequencing. In our experiment, 56 of the 60 pairs of primers worked. Afterward, genomic annotation was used to assign these SNPs into different categories related to transcription and translation, including genic regions (CDS, introns, and UTRs), promoter regions and intergenic regions using our in-house PERL scripts. SNPs that resulted in premature stop codons, disrupted start/stop codons or splice donor/acceptor sites were defined as radical SNPs.
Determining SNP genotypes for each accession
For each accession, we extracted the SNP genotype information from the output of SOAPsnp. Again, we conducted a series of quality control procedures to ensure the reliability of the genotype. The consensus files contained information including the best base genotype and its average quality score, the second best base genotype and its average quality score, and the consensus genotype and its quality scores for each site, which can be used as filters [ 50 ]. We used very stringent criterion to ensure that only genotypes with a quality score > =20 (with error rate no more than 1%) were taken into consideration. More explicitly, if the average quality score of the best base is > =20 while the average quality score of the second best base is < 20, then that genotype is the best base. If both the average quality score of the best and second best base are > =20, then the genotype is heterozygous. If both the average quality score of the best and second best base are < 20 but the quality score of the consensus genotype is > =20, then the consensus genotype is used. On average 67% of the SNP genotypes were missing for each accession due to the relatively low coverage of our re-sequencing data. However, this missing data will likely not undermine our analyses because the phylogenetic analysis is based on pair-wise distances averaged over many sites and the population genetics analyses are based on allele frequencies. As such, the data are sufficient to estimate the pair-wise distances and allele frequencies. Besides the 166 accessions we re-sequenced, the genotypes of the SNPs for the wild accessions were determined using the same method.
Constructing the phylogenetic tree
Incorporating the genotype information of wild rice accessions, we use the SNP genotypes of each accession to calculate the pair-wise distances between any two accessions using PHYLIP [ 52 ]. We used default parameters to calculate the distances, i.e. using F84 model, a transition/transversion ratio of 2.0 and no Gamma distributed rates across sites. We assumed a uniform mutation rate across the genome and thus gave same weight for different SNPs. Bootstrap with 100 replicates was conducted for the whole genome SNP data set. After the genetic distances were calculated for the 100 replicate data sets, phylogenetic trees were constructed using the Neighbor-Joining (NJ) approach based on the genetic distances [ 52 ]. The consensus tree was then built using the built-in ‘consense’ program of PHYLIP with the parameters, ‘strict majority rule’ and ‘trees to be treated as unrooted’.
Population structure inference
We first conducted a PCA analysis using the procedure as reported [ 53 ]. 24890 SNPs with genotype data covering more than 100 accessions was used in the analysis. Moreover, the FRAPPE software [ 24 ], which used a frequentist approach to estimate individual admixture, was used to examine the population structure as well. The maximum iteration of EM to run is set as 10000.
Calculating F ST
The allele frequencies of SNP alleles were determined based on the accessions’ genotypes. SNPs with less than 10 individuals’ genotype information in one population were discarded when calculating the allele frequencies for that particular population. Based on the allele frequencies, we calculated the ‘ F ST ’ using the method described by Nei [ 54 ]. In the whole-genome scan, 20-kb sliding windows were used with 2-kb sliding step. By averaging the F ST values over SNP sites in each window, F ST values for the windows were obtained.
Scanning selective sweep using composite likelihood approaches
Cross-population composite likelihood ratio test (XP-CLR) and Nielsen’s composite likelihood ratio test (CLR) are two approaches for identifying selective sweep signals using SNP data sets [ 17 , 18 ]. XP-CLR incorporates the allele frequency differentiation patterns between two populations and multilocus frequency spectrum to identify selective sweeps occurring in population differentiation. The Nielsen’s CLR method uses the distribution pattern of allele frequency spectrum in a particular population to search for selective sweep signals in that population. In our analysis, we combined XP-CLR and Nielsen’s CLR using the software XP-CLR and SweepFinder, respectively. In the XP-CLR analysis, we used the allele frequencies of both upland and irrigated populations, calculating XP-CLR scores and picking the top 5‰ regions. When calculating the XP-CLR scores, we used a window size of 0.1 cM, a 2-kb grid size and a maximum SNP number of 150 for each window. By overlapping these regions of top XP-CLR scores with those of top 5‰ F ST , we obtained a conservative set of ecotype differentiation regions (EDRs) between upland and irrigated rice and the ecotype differentiated genes (EDGs) within these regions. In Nielsen’s CLR analysis, we used allele frequencies of a single population (upland or irrigated population) and found the top 5% grid points with the highest likelihood ratio (LR) values in the genome. Genes within 10 kb of these grid points were considered as the potential selection targets in either rice population [ 55 ]. Fine-scale grid points (a grid point at every 1000 nucleotides) were used when calculating the LR values.
Gene enrichment analysis for the EDGs
Gene enrichment analysis was done using chi-square test. For a specific category of genes, we calculated the gene number in the category within the whole genome using the rice gene annotation information [ 56 ]. For example, for the gene category “lipase-containing GDSL domain”, we counted the number of lipase genes annotated with this domain in the rice gene annotation database [ 56 ]. The number of genes in this category was also counted in the EDGs. A contingency table was then constructed based on the number of interesting genes in the EDGs, the total gene number of EDGs, the number of interesting genes in the whole genome, and the total gene number in the whole genome. We then conducted the Chi-square test and obtained P -values.
Accession codes
The raw sequence data of the 166 varieties has been deposited into the NCBI Short Read Archive (SRA, http://www.ncbi.nlm.nih.gov/sra/ ) under accession number SRA066116.
Abbreviations
Cross-population composite likelihood ratio
Composite likelihood ratio
Ecotype differentiated region
Genes in EDR
Ecotype differentiated SNPs.
Khush GS: Origin, dispersal, cultivation and variation of rice. Plant Mol Biol. 1997, 35 (1–2): 25-34.
Article CAS PubMed Google Scholar
Chang TT VS: Varietal diversity and morpho-agronomic characteristics of upland rice. 1975, Baiios, Philippines: Major research in upland rice, International Rice Research Institute, 72-90.
Google Scholar
Chang TT LC, Tagumpay O: Agronomic and growth characteristics of upland and lowland rice varieties. 1972, Baiios, Philippines: Rice breeding, International Rice Research Institute, 645-661.
IRRI: An Overview of Upland Rice Research. Proceedings of the 1982 Bouake. 1984, LOS BAÑOS, LAGUNA, PHILIPPINES: Ivory Coast Upland Rice Workshop, International Rice Research Institute(IRRI), 1-500.
Institute IRR: Major Research in Upland Rice. 1975, Baiios, Philippines: International Rice Research Institute(IRRI)
Bernier J, Atlin GN, Serraj R, Kumar A, Spaner D: Review: breeding upland rice for drought resistance. 2008
Price AH, Steele KA, Moore BJ, Barraclough PB, Clark LJ: A combined RFLP and AFLP linkage map of upland rice (Oryza sativa L.) used to identify QTLs for root-penetration ability. Theor Appl Genet. 2000, 100 (1): 49-56.
Article CAS Google Scholar
Cairns JE, Audebert A, Mullins CE, Price AH: Mapping quantitative trait loci associated with root growth in upland rice (Oryza sativa L.) exposed to soil water-deficit in fields with contrasting soil properties. Field Crop Res. 2009, 114 (1): 108-118.
Article Google Scholar
Price AH, Steele KA, Moore BJ, Jones RGW: Upland rice grown in soil-filled chambers and exposed to contrasting water-deficit regimes II. Mapping quantitative trait loci for root morphology and distribution. Field Crop Res. 2002, 76 (1): 25-43.
Zhu JK: Salt and drought stress signal transduction in plants. Annu Rev Plant Biol. 2002, 53: 247-273.
Article PubMed Central CAS PubMed Google Scholar
Thompson AJ, Jackson AC, Symonds RC, Mulholland BJ, Dadswell AR, Blake PS, Burbidge A, Taylor IB: Ectopic expression of a tomato 9-cis-epoxycarotenoid dioxygenase gene causes over-production of abscisic acid. Plant J. 2000, 23 (3): 363-374.
Iuchi S, Kobayashi M, Taji T, Naramoto M, Seki M, Kato T, Tabata S, Kakubari Y, Yamaguchi-Shinozaki K, Shinozaki K: Regulation of drought tolerance by gene manipulation of 9-cis-epoxycarotenoid dioxygenase, a key enzyme in abscisic acid biosynthesis in Arabidopsis. Plant J. 2001, 27 (4): 325-333.
Kasuga M, Liu Q, Miura S, Yamaguchi-Shinozaki K, Shinozaki K: Improving plant drought, salt, and freezing tolerance by gene transfer of a single stress-inducible transcription factor. Nat Biotechnol. 1999, 17 (3): 287-291.
Garg AK, Kim JK, Owens TG, Ranwala AP, Choi YD, Kochian LV, Wu RJ: Trehalose accumulation in rice plants confers high tolerance levels to different abiotic stresses. Proc Natl Acad Sci U S A. 2002, 99 (25): 15898-15903.
Jang IC, Oh SJ, Seo JS, Choi WB, Song SI, Kim CH, Kim YS, Seo HS, Choi YD, Nahm BH, Kim JK: Expression of a bifunctional fusion of the Escherichia coli genes for trehalose-6-phosphate synthase and trehalose-6-phosphate phosphatase in transgenic rice plants increases trehalose accumulation and abiotic stress tolerance without stunting growth. Plant Physiol. 2003, 131 (2): 516-524.
Xiong L, Yang Y: Disease resistance and abiotic stress tolerance in rice are inversely modulated by an abscisic acid-inducible mitogen-activated protein kinase. Plant Cell. 2003, 15 (3): 745-759.
Chen H, Patterson N, Reich D: Population differentiation as a test for selective sweeps. Genome Res. 2010, 20 (3): 393-402.
Nielsen R, Williamson S, Kim Y, Hubisz MJ, Clark AG, Bustamante C: Genomic scans for selective sweeps using SNP data. Genome Res. 2005, 15 (11): 1566-1575.
Lyu J, Zhang S, Dong Y, He W, Zhang J, Deng X, Zhang Y, Li X, Li B, Huang W, Wan W, Yu Y, Li Q, Li J, Liu X, Wang B, Tao D, Zhang G, Wang J, Xu X, Hu F, Wang W: Analysis of elite variety tag SNPs reveals an important allele in upland rice. Nat Commun. 2013, 4: 2138-
Article PubMed Central PubMed Google Scholar
Li R, Li Y, Kristiansen K, Wang J: SOAP: short oligonucleotide alignment program. Bioinformatics. 2008, 24 (5): 713-714.
Xu X, Liu X, Ge S, Jensen JD, Hu F, Li X, Dong Y, Gutenkunst RN, Fang L, Huang L, Li J, He W, Zhang G, Zheng X, Zhang F, Li Y, Yu C, Kristiansen K, Zhang X, Wang J, Wright M, McCouch S, Nielsen R, Wang W: Resequencing 50 accessions of cultivated and wild rice yields markers for identifying agronomically important genes. Nat Biotechnol. 2012, 30 (1): 105-111.
Glaszmann JC: Isozymes and classification of Asian rice varieties. Theor Appl Genet. 1987, 74 (1): 21-30.
Huang X, Kurata N, Wei X, Wang ZX, Wang A, Zhao Q, Zhao Y, Liu K, Lu H, Li W, Guo Y, Lu Y, Zhou C, Fan D, Weng Q, Zhu C, Huang T, Zhang L, Wang Y, Feng L, Furuumi H, Kubo T, Miyabayashi T, Yuan X, Xu Q, Dong G, Zhan Q, Li C, Fujiyama A, Toyoda A, et al: A map of rice genome variation reveals the origin of cultivated rice. Nature. 2012, 490 (7421): 497-501.
Tang H, Peng J, Wang P, Risch NJ: Estimation of individual admixture: analytical and study design considerations. Genet Epidemiol. 2005, 28 (4): 289-301.
Article PubMed Google Scholar
Hu HH, You J, Fang YJ, Zhu XY, Qi ZY, Xiong LZ: Characterization of transcription factor gene SNAC2 conferring cold and salt tolerance in rice. Plant Mol Biol. 2008, 67 (1–2): 169-181.
Hong JK, Choi HW, Hwang IS, Kim DS, Kim NH, du Choi S, Kim YJ, Hwang BK: Function of a novel GDSL-type pepper lipase gene, CaGLIP1, in disease susceptibility and abiotic stress tolerance. Planta. 2008, 227 (3): 539-558.
Gupta S, Bharalee R, Bhorali P, Bandyopadhyay T, Gohain B, Agarwal N, Ahmed P, Saikia H, Borchetia S, Kalita MC, Handique AK, Das S: Identification of drought tolerant progenies in tea by gene expression analysis. Funct Integr Genomics. 2012, 12 (3): 543-563.
Wu Q, Lin J, Liu JZ, Wang X, Lim W, Oh M, Park J, Rajashekar CB, Whitham SA, Cheng NH, Hirschi KD, Park S: Ectopic expression of Arabidopsis glutaredoxin AtGRXS17 enhances thermotolerance in tomato. Plant Biotechnol J. 2012, 10 (8): 945-955.
Price AH, Steele KA, Gorham J, Bridges JM, Moore BJ, Evans JL, Richardson P, Jones RGW: Upland rice grown in soil-filled chambers and exposed to contrasting water-deficit regimes I. Root distribution, water use and plant water status. Field Crop Res. 2002, 76 (1): 11-24.
Liu DD, Dong QL, Fang MJ, Chen KQ, Hao YJ: Ectopic expression of an apple apomixis-related gene MhFIE induces co-suppression and results in abnormal vegetative and reproductive development in tomato. J Plant Physiol. 2012, 169 (18): 1866-1873.
To JP, Haberer G, Ferreira FJ, Deruere J, Mason MG, Schaller GE, Alonso JM, Ecker JR, Kieber JJ: Type-A Arabidopsis response regulators are partially redundant negative regulators of cytokinin signaling. Plant Cell. 2004, 16 (3): 658-671.
Hirose N, Makita N, Kojima M, Kamada-Nobusada T, Sakakibara H: Overexpression of a type-A response regulator alters rice morphology and cytokinin metabolism. Plant Cell Physiol. 2007, 48 (3): 523-539.
Argyros RD, Mathews DE, Chiang YH, Palmer CM, Thibault DM, Etheridge N, Argyros DA, Mason MG, Kieber JJ, Schaller GE: Type B response regulators of Arabidopsis play key roles in cytokinin signaling and plant development. Plant Cell. 2008, 20 (8): 2102-2116.
Hill K, Mathews DE, Kim HJ, Street IH, Wildes SL, Chiang YH, Mason MG, Alonso JM, Ecker JR, Kieber JJ, Schaller GE: Functional characterization of type-B response regulators in the Arabidopsis cytokinin response. Plant Physiol. 2013, 162 (1): 212-224.
Ulker B, Somssich IE: WRKY transcription factors: from DNA binding towards biological function. Curr Opin Plant Biol. 2004, 7 (5): 491-498.
Zhang Y, Wang L: The WRKY transcription factor superfamily: its origin in eukaryotes and expansion in plants. BMC Evol Biol. 2005, 5: 1-
Eulgem T, Rushton PJ, Robatzek S, Somssich IE: The WRKY superfamily of plant transcription factors. Trends Plant Sci. 2000, 5 (5): 199-206.
Rabello AR, Guimaraes CM, Rangel PH, da Silva FR, Seixas D, de Souza E, Brasileiro AC, Spehar CR, Ferreira ME, Mehta A: Identification of drought-responsive genes in roots of upland rice (Oryza sativa L). BMC Genomics. 2008, 9: 485-
Cheong YH, Pandey GK, Grant JJ, Batistic O, Li L, Kim BG, Lee SC, Kudla J, Luan S: Two calcineurin B-like calcium sensors, interacting with protein kinase CIPK23, regulate leaf transpiration and root potassium uptake in Arabidopsis. Plant J. 2007, 52 (2): 223-239.
Umehara M, Hanada A, Yoshida S, Akiyama K, Arite T, Takeda-Kamiya N, Magome H, Kamiya Y, Shirasu K, Yoneyama K, Kyozuka J, Yamaguchi S: Inhibition of shoot branching by new terpenoid plant hormones. Nature. 2008, 455 (7210): 195-200.
Wang H, Huang Z, Chen Q, Zhang Z, Zhang H, Wu Y, Huang D, Huang R: Ectopic overexpression of tomato JERF3 in tobacco activates downstream gene expression and enhances salt tolerance. Plant Mol Biol. 2004, 55 (2): 183-192.
Zhao K, Tung CW, Eizenga GC, Wright MH, Ali ML, Price AH, Norton GJ, Islam MR, Reynolds A, Mezey J, McClung AM, Bustamante CD, McCouch SR: Genome-wide association mapping reveals a rich genetic architecture of complex traits in Oryza sativa. Nat Commun. 2011, 2: 467-
Xing T, Wang XJ, Malik K, Miki BL: Ectopic expression of an Arabidopsis calmodulin-like domain protein kinase-enhanced NADPH oxidase activity and oxidative burst in tomato protoplasts. Mol Plant Microbe Interact. 2001, 14 (10): 1261-1264.
Ono S: Upland Rice Breeding in Japan. 1971, Japan Agricultural Research Quarterly: Tokyo
Turner TL, Bourne EC, Von Wettberg EJ, Hu TT, Nuzhdin SV: Population resequencing reveals local adaptation of Arabidopsis lyrata to serpentine soils. Nat Genet. 2010, 42 (3): 260-263.
Huang X, Wei X, Sang T, Zhao Q, Feng Q, Zhao Y, Li C, Zhu C, Lu T, Zhang Z, Li M, Fan D, Guo Y, Wang A, Wang L, Deng L, Li W, Lu Y, Weng Q, Liu K, Huang T, Zhou T, Jing Y, Lin Z, Buckler ES, Qian Q, Zhang QF, Li J, Han B: Genome-wide association studies of 14 agronomic traits in rice landraces. Nat Genet. 2010, 42 (11): 961-967.
Huang N, Courtois B, Khush GS, Lin HX, Wang GL, Wu P, Zheng KL: Association of quantitative trait loci for plant height with major dwarfing genes in rice. Heredity. 1996, 77: 130-137.
Mu P, Li ZC, Li CP, Zhang HL, Wu CM, Li C, Wang XK: QTL mapping of the root traits and their correlation analysis with drought resistance using DH lines from paddy and upland rice cross. Chinese Science Bulletin. 2003, 48 (24): 2718-2724.
Richards E, Reichardt M, Rogers S: Preparation of genomic DNA from plant tissue. Curr Protoc Mol Biol. 2001, Chapter 2:Unit2 3
Li R, Li Y, Fang X, Yang H, Wang J, Kristiansen K: SNP detection for massively parallel whole-genome resequencing. Genome Res. 2009, 19 (6): 1124-1132.
Lam HM, Xu X, Liu X, Chen W, Yang G, Wong FL, Li MW, He W, Qin N, Wang B, Li J, Jian M, Wang J, Shao G, Sun SS, Zhang G: Resequencing of 31 wild and cultivated soybean genomes identifies patterns of genetic diversity and selection. Nat Genet. 2010, 42 (12): 1053-1059.
Felsenstein J: PHYLIP -- Phylogeny Inference Package (Version 3.2). Cladistics. 1989, 164-166. 5
Patterson N, Price AL, Reich D: Population structure and eigenanalysis. PLoS Genet. 2006, 2 (12): e190-
Nei M: Molecular Evolutionary Genetics. 1987, New York: Columbia University Press
Williamson SH, Hubisz MJ, Clark AG, Payseur BA, Bustamante CD, Nielsen R: Localizing recent adaptive evolution in the human genome. PLoS Genet. 2007, 3 (6): e90-
Tanaka T, Antonio BA, Kikuchi S, Matsumoto T, Nagamura Y, Numa H, Sakai H, Wu J, Itoh T, Sasaki T, Aono R, Fujii Y, Habara T, Harada E, Kanno M, Kawahara Y, Kawashima H, Kubooka H, Matsuya A, Nakaoka H, Saichi N, Sanbonmatsu R, Sato Y, Shinso Y, Suzuki M, Takeda J, Tanino M, Todokoro F, Yamaquchi K, Rice Annotation Project, et al: The Rice Annotation Project Database (RAP-DB): 2008 update. Nucleic Acids Res. 2008, 36 (Database issue): D1028-D1033.
CAS PubMed Google Scholar
Download references
Acknowledgments
The Southwest ultra-Computer Center of Chinese Academy of Sciences provided the computing resources. We would like to thank Dr. Hua Chen from the Harvard School of Public Health for his constructive suggestions about data analysis. And thank Dr. Hui Xiang for her help editing the manuscript. This work was supported by the Department of Sciences and Technology of Yunnan Province (2011BB015, 2013HA004 and 2013GA004), the Chinese 973 Program (2013CB835200, 2013CB835201).
Author information
Authors and affiliations.
CAS-Max Planck Junior Research Group, State Key Laboratory of Genetic Resources and Evolution, Kunming Institute of Zoology, Chinese Academy of Sciences, Kunming, 650223, China
Jun Lyu, Zhiheng Gou & Wen Wang
Food Crops Research Institute, Yunnan Academy of Agricultural Sciences, Kunming, 650205, China
Baoye Li, Shilai Zhang, Jing Zhang, Dayun Tao, Wangqi Huang & Fengyi Hu
BGI-Shenzhen, Shenzhen, 518083, China
Inner Mongolia Agricultural University, Hohhot, 010018, China
Center for Epigenetics, Johns Hopkins University School of Medicine Baltimore, Baltimore, MD, 21205, USA
You can also search for this author in PubMed Google Scholar
Corresponding authors
Correspondence to Jun Lyu , Fengyi Hu or Wen Wang .
Additional information
Competing interests.
The authors declare that they have no competing interests.
Authors’ contributions
JL, WW, FH designed the project. FH and DT provided the rice materials. HL, JL, LM performed the sequencing, JL, ZG, WH and XL analyzed the data. BL did the phenotyping. JL, SZ, JZ, WH and BL cultivated rice. JL and WW wrote the manuscript. All authors read and approved the final manuscript.
Electronic supplementary material
12870_2014_1574_moesm1_esm.docx.
Additional file 1: Geographic distribution of accessions. Blue dots show the origins of these accessions, while the dot sizes roughly correspond to the numbers of the accessions from certain areas. (DOCX 1 MB)
Additional file 2: Basic information of the 166 sequenced accessions.(DOCX 39 KB)
Additional file 3: basic information of the phenotyped accessions.(docx 19 kb), 12870_2014_1574_moesm4_esm.xlsx.
Additional file 4: The phenotypes of the accessions under rainfed upland condition (a) and irrigated condition (b).(XLSX 66 KB)
12870_2014_1574_MOESM5_ESM.docx
Additional file 5: Phenotype comparison between five upland and five irrigated accessions. The left five accessions are irrigated rice (in order from left to right, Hongyou 4, Diantun 502, Yunhui 290, Hexi 42, Taizhong 65). The right five accessions are upland rice (in order from left to right IRAT104, CNA4140, Sanlicun, Arias Halus, Dourado). This image indicates that the upland type generally have higher architecture, better developed roots, and fewer tillers. (DOCX 17 MB)
12870_2014_1574_MOESM6_ESM.xlsx
Additional file 6: The correlation between each of the four traits (root weight, maxiaml root length, tiller number and plant height) and the yeild trais (1000-grain weight, number of filled grains per panicle, number of empty grains per panicle and number of productive panicles) under the upland condition.(XLSX 14 KB)
12870_2014_1574_MOESM7_ESM.xlsx
Additional file 7: The maximal main root length (a) and the number of empty grain (b) under irrigated and upland conditions and statistical test.(XLSX 21 KB)
Additional file 8: Mean depth and coverage rate for each accession.(DOCX 18 KB)
Additional file 9: the snps for the whole population.(zip 14 mb), 12870_2014_1574_moesm10_esm.docx.
Additional file 10: SNP annotation for the 166 accessions. a, using genome annotation information we classify the SNPs into intergenic SNPs and SNPs in gene region, which are further classified as SNPs in intron or UTR regions, non-synonymous SNPs and synonymous SNPs. b, SNPs causing radical mutations including disrupting stop/start codons, causing premature stop codons or disupting splicing sites. (DOCX 14 KB)
Additional file 11: Wild rice information.(DOCX 14 KB)
12870_2014_1574_moesm12_esm.docx.
Additional file 12: Phylogenetics analysis of the upland accessions. Green, black and orange branches respectively refer to upland, irrigated and wild accessions. The leaf nodes are labeled with the sample number of the accessions. This tree indicates that upland rice seems to have originated multiple times for the type Indica, while upland japonicas probably derived from a single origin. (DOCX 660 KB)
12870_2014_1574_MOESM13_ESM.docx
Additional file 13: Five of the six erroneously classified accessions. (a), typical indica seed; (b), typical japonica seed; (c) The accessions, GS190(TOS2300), GS192(WAB56-125), GS199(CNA4140), GS200 (GUARANI), GS201(Dourado), GS202(TGR78), were previously classified as upland indica according to their phenotypes (i.e. seed shape, etc.). According to our whole genome phylogenetics analysis, they are more close to upland japonica. GS200 was not photographed due to seeds being unavailable. (DOCX 432 KB)
Additional file 14: EDR length distribution. Median length is 22721 bp. (DOCX 13 KB)
12870_2014_1574_moesm15_esm.docx.
Additional file 15: Annotation of SNPs in EDRs. In EDRs, there are 8980 SNPs, of which 2409 are located within gene regions. In the gene regions, 241 and 266 SNPs are synonymous and non-synonymous, respectively. (DOCX 12 KB)
Additional file 16: Distribution pattern of SNPs in EDRs.(DOCX 12 KB)
Additional file 17: ecotype differentiated snps (eds) between upland and irrigated populations.(docx 12 kb), additional file 18: the 154 edgs.(docx 23 kb), additional file 19: enrichment analysis for interesting gene categories in edgs and japonica-specific edgs.(docx 17 kb), 12870_2014_1574_moesm20_esm.docx.
Additional file 20: Length distribution of selective sweep regions in the upland(a) and irrigated(b) population. Median length is 27002 bp(a) and 41001 bp(b) respectively. (DOCX 14 KB)
12870_2014_1574_MOESM21_ESM.docx
Additional file 21: EDR scanning for type japonica. (a) F ST values between upland japonica and irrigated japonica were plotted against physical coordinates over the genome; the red horizontal dashed line refers to the top 5‰ threshold. (b), XP-CLR scores were plotted against genomic coordinates; the green horizontal dashed line refers to the top 5‰ threshold. EDRs were obtained by taking the intersection of the EDRs given by the two approaches. The vertical dashed lines in (a) and (b) indicate the boundaries between two chromosomes. (DOCX 278 KB)
Additional file 22: Length distribution of EDRs in type japonica. Median length is 24019 bp. (DOCX 13 KB)
Additional file 23: detailed information of the 169 japonica-specific edgs.(docx 21 kb), additional file 24: detailed information of the 56 indica-specific edgs.(docx 15 kb), 12870_2014_1574_moesm25_esm.docx.
Additional file 25: EDR scanning for type indica. (a) F ST values between upland indica and irrigated indica were plotted against physical coordinates over the genome; the red horizontal dashed line refers to the top 5‰ threshold. (b), XP-CLR scores were plotted against genomic coordinates; the green horizontal dashed line refers to the top 5‰ threshold. EDRs were obtained by taking the intersection of the EDRs given by the two approaches. The vertical dashed lines in (a) and (b) indicate the boundaries between two chromosomes. (DOCX 222 KB)
12870_2014_1574_MOESM26_ESM.docx
Additional file 26: Nine genes from the indica XP-CLR top signals overlap with the previous EDGs of merged population.(DOCX 12 KB)
12870_2014_1574_MOESM27_ESM.docx
Additional file 27: Four genes from the indica XP-CLR top signals overlap with the previous EDGs of the japonica population.(DOCX 11 KB)
12870_2014_1574_MOESM28_ESM.xls
Additional file 28: The numbers of informative sites for computing the pair-wise distance between any two accessions.(XLS 254 KB)
Authors’ original submitted files for images
Below are the links to the authors’ original submitted files for images.
Authors’ original file for figure 1
Authors’ original file for figure 2, authors’ original file for figure 3, authors’ original file for figure 4, rights and permissions.
This article is published under license to BioMed Central Ltd. This is an Open Access article distributed under the terms of the Creative Commons Attribution License ( http://creativecommons.org/licenses/by/4.0 ), which permits unrestricted use, distribution, and reproduction in any medium, provided the original work is properly credited. The Creative Commons Public Domain Dedication waiver ( http://creativecommons.org/publicdomain/zero/1.0/ ) applies to the data made available in this article, unless otherwise stated.
Reprints and permissions
About this article
Cite this article.
Lyu, J., Li, B., He, W. et al. A genomic perspective on the important genetic mechanisms of upland adaptation of rice. BMC Plant Biol 14 , 160 (2014). https://doi.org/10.1186/1471-2229-14-160
Download citation
Received : 12 February 2014
Accepted : 06 June 2014
Published : 11 June 2014
DOI : https://doi.org/10.1186/1471-2229-14-160
Share this article
Anyone you share the following link with will be able to read this content:
Sorry, a shareable link is not currently available for this article.
Provided by the Springer Nature SharedIt content-sharing initiative
- Upland rice
- Upland adaptation
- Genetic mechanisms
- Phylogenetics
- Population structure
- Artificial selection
BMC Plant Biology
ISSN: 1471-2229
- General enquiries: [email protected]
Upland rice: Challenges and opportunities in a less favorable ecosystem
- Published: March 1995
- Volume 35 , pages 325–328, ( 1995 )
Cite this article
- Arraudeau M. A. 1 , 2
201 Accesses
10 Citations
Explore all metrics
IRRI's upland rice research has made progress in solving some of the dilemmas in the upland rice ecosystem, although many challenges remain. IRRI researchers are gaining a better understanding of the ecosystem's constraints, opportunities, and research approaches. Cultivar improvement, use of farmer participatory methods to reduce erosion, and weed management are areas where research advances are being made. Developing perennial upland rice and managing weeds using less herbicide are exciting new projects being pursued. Enhancing rice productivity is seen as an entry point to alleviating the interrelated problems of declining productivity, lack of sustainability, and poverty in the uplands. Delay can irreparably damage the uplands and gravely affect the people who depend on them for survival.
This is a preview of subscription content, log in via an institution to check access.
Access this article
Price includes VAT (Russian Federation)
Instant access to the full article PDF.
Rent this article via DeepDyve
Institutional subscriptions
Adhikari, N. P.: Physio-morphological Responses of Upland Rice to Shade. M. S. Thesis, University of the Philippines at Los Baños, Laguna, Philippines 1990.
Google Scholar
Arraudeau, M.; Harahap, Z.: Relevant upland rice breeding objectives. In: Progress in Upland Rice Research. Proceedings of the 1985 Jakarta Conference, 189–197. International Rice Research Institute, Los Baños, Laguna, Philippines 1986.
Arraudeau, M. A.; Vergara, B. S.: A Farmer's Primer on Growing Upland Rice. International Rice Research Institute, Los Baños, Laguna, Philippines 1988.
Arya, L. M.: Properties and Processes in Upland Acid Soils in Sumatra and Their Management for Crop Production. Sukarami Research Institute for Food Crops, Padang 1990.
Castano, J.; Nurdin, F.; Hasan, N.: Major Diseases and Insect Pests of Upland Rice in Sumatra. Sukarami Research Institute for Food Crops, Padang 1990.
Chavez, R.C.; Moody, K.: Possible new approaches for chemical weed control in upland rice. Paper presented at the 20th Annual Conference of the Pest Control Council of the Philippines, Baguio City, Philippines 1989.
Clement, G: Upland Rice Improvement by Hybridization. Diallel Analysis of Eight Quantitative Characters in The Broad Sense Japonica Group — Application to Breeding. Montpellier, Ph.D. Thesis — l'Institut National Polytechnique de Toulouse (English summary) (CIRAD-IRAT) 1987.
Courtois, B.: Upland Rice Cropping Systems. Mem. Trav. IRAT 16, 96 (1988)
Courtois B.; Jacquot, M.: The Culture Systems of Upland Rice. Mem. Trav. IRAT 7, 98 (1984)
De Raissac, M.; Da Silva Pinheiro, B.: Annual Report, Season 1989–1990: Identification of Physiological Parameters for Drought Tolerance in Upland Rice. Goiania, CIRAD-IRAT (1990)
Dingkuhn, M.; Cruz, R. T.; O'Toole, J. C.; Doerffling, K.: Net photosynthesis, water use efficiency, leaf water potential and leaf rolling as affected by water deficit in tropical upland rice. Aust. J. Agric. Res. 40 (6):1171–1181 (1989)
Fukoshima, M.T.; Hinata, K.; Tsunoda, S.; Drought resistance in upland rice varieties ( Oryza sativa L.) 4. Effect of defoliation under dry conditions to the photosynthesis and yield. Jpn. J. Breed. 34 (Suppl. 1):356–357 (1984)
Fukoshima, M.T.; Hinata, K.; Tsunoda, S.; Drought resistance in upland rice varieties ( Oryza sativa L.) 5-6 Jpn. J. Breed. 34 (Suppl. 2): 136–137; 138–139 (1984)
Fukoshima, M. T.; Tsunoda, S.: Drought resistance in upland rice varieties ( Oryza sativa L.). 2. Morphological responses to drought conditions. Jpn. J. Breed. 33 (Suppl. 1): 252–253 (1983)
Fukoshima, M. T.; Tsunoda, S.; Hinata, K.: Drought resistance in upland rice varieties ( Oryza sativa L.). 3. The influence of soil moisture conditions on the physiological responses of rice varieties. Jpn. J. Breed. 33 (Suppl. 2): 168–169 (1983)
Garrity, D. P.; Movillon, M.; Moody, K.: Differential weed suppression ability in upland rice cultivars. Agron. J. 84(4): 586–591 (1992)
Garrity, D. P.: Upland Rice Research: An International Bibliography, 1965–1982. International Rice Research Institute, Los Baños, Philippines 1983.
Gupta, P. C.; O'Toole, J. C.: Upland Rice: A Global Perspective. International Rice Research Institute, Los Baños, Laguna, Philippines 1986.
IRRI-International Rice Research Institute: An Overview of Upland Rice Research: Proceedings of the 1982 Bouake, Ivory Coast, Upland Rice Workshop. Los Baños, Philippines 1984.
IRRI-International Rice Research Institute: Progress in Upland Rice Research: Proceedings of the 1985 Jakarta Conference. Los Baños 1986.
Jacquot, M.; Courtois, B: Upland Rice. Macmillan, London 1987. (The Tropical Agriculturalist). Translated by P. Skinner.
Jacquot, M.; Courtois, B.: Upland Rice. G.P. Maisonneuve et Larousse, Paris 1983.
Jacquot, M.; Mutagenesis of upland rice at IRAT. Mutat. Breed. Newsl. 28:10–12 (1986)
Kirk, G. J. D.; Hedley, M. J.; Bouldin, D. R.; Phosphorus efficiency in upland rice cultivars. In: Proceedings 2nd Annual Meeting of IBSRAM Asialand Network on Management of Acid Soils, 1–21. IBSRAM, Bangkok 1992.
Litsinger, J.A.; Libetario, E.M.; Maclean, R.H.; Batay-An, E.H.: Integrated Insect Pest Management in Upland Rice Farming Systems. Paper presented at the International Rice Research Conference, International Rice Research Institute 1988.
Maurya, D. M; Bottrall, A.; Farrington, J.: Improved livelihoods, genetic diversity and farmer participation: a strategy for rice breeding in rainfed areas of India. Exp. Agric. 24(3): 311–320 (1988)
Moody, K.: Weed control in upland rice with emphasis on grassy weeds. In: Baker, F. W.G. and Terry, P. J. (eds.), Tropical Grassy Weeds, 164–178. CAB International for CASAFA, Wallingford, United Kingdom 1981.
Notteghem, J. L.: Selection of Upland Rice Varieties With Horizontal Resistance: Toward Success or Toward Failure? Are the Work Rhythms of Plant Breeders and Plant Pathologists Compatible? 1985.
Pinnschmidt, H.: Field Studies on the Epidemiology of Pyricularia oryzae Cav. in Upland Rice. Thesis (Fachber.Agrarwiss.) — Justus-Liebig Universität Giessen, 1989.
Prot, J. C.: Plant Parasitic Nematodes Associated With Upland Rice in Sitiung, West Sumatra, Indonesia. In: Coop. CRIFC/IRRI Prog. Quarterly Report, January–March 1991, Appendix 21, 3, 1991.
Prot, J-C.: Monetary value estimates of nematode problems, research proposal and priorities: The rice example in South and Southeast Asia. Fundamental and Applied Nematology 16:385- 388 (1993)
Santoso, D.; Sudjadi, M.; Mamaril, C.P.: Integrated Nutrient Management for Sustainable Rice Farming in Upland Rice Environment. Paper presented at the International Rice Research Conference, International Rice Research Institute, 1988.
Sri Adiningsih, J.; Semali, A.; Effendi, S.; Hadiwegeno, S: Resources and problems associated with the development of upland areas in Indonesia. In: Blair, G and Lefroy R. (eds.), Technologies for Sustainable Agriculture on Marginal Uplands in Southeast Asia, 45–54. Australian Centre for International Agricultural Research, Canberra 1991.
Taher, A.: Physiological and lipid changes in some upland rice ( Oryza Sativa L.) cultivars grown under drought stress. PhD Thesis. University of the Philippines at Los Baños, Laguna, Philippines 1988.
Uexkull, H. R. von: Improvement and Maintenance of Soil Fertility in Tropical Upland Farming Systems. In: Potassium in the Agricultural Systems of the Humid Tropics 233–250, International Potash Institute, Bern 1985.
WARDA-West Africa Rice Development Association: Upland rice in West Africa. In: An Overview of Upland Rice Research, 21–43. International Rice Research Institute, Los Baños, Philippines 1984.
Yamauchi, A.; Kono, Y.; Tatsumi, J.: Quantitative analysis on root system structures of upland rice and maize. Jpn.J. Crop Sci. 56 (4):608–617 (1987) (Japanese summary)
Download references
Author information
Authors and affiliations.
International Rice Research Institute, PO Box 933, 1099, Manila, Philippines
Arraudeau M. A.
Moulin de Valenfroid, 86160, Sommieres du Clain, France
You can also search for this author in PubMed Google Scholar
Rights and permissions
Reprints and permissions
About this article
Arraudeau, M.A. Upland rice: Challenges and opportunities in a less favorable ecosystem. GeoJournal 35 , 325–328 (1995). https://doi.org/10.1007/BF00989139
Download citation
Issue Date : March 1995
DOI : https://doi.org/10.1007/BF00989139
Share this article
Anyone you share the following link with will be able to read this content:
Sorry, a shareable link is not currently available for this article.
Provided by the Springer Nature SharedIt content-sharing initiative
- Environmental Management
- Entry Point
- Participatory Method
- Research Approach
- Rice Productivity
- Find a journal
- Publish with us
- Track your research
Thank you for visiting nature.com. You are using a browser version with limited support for CSS. To obtain the best experience, we recommend you use a more up to date browser (or turn off compatibility mode in Internet Explorer). In the meantime, to ensure continued support, we are displaying the site without styles and JavaScript.
- View all journals
- My Account Login
- Explore content
- About the journal
- Publish with us
- Sign up for alerts
- Review Article
- Open access
- Published: 14 October 2022
Two decades of rice research in Indonesia and the Philippines: A systematic review and research agenda for the social sciences
- Ginbert P. Cuaton ORCID: orcid.org/0000-0002-5902-3173 1 &
- Laurence L. Delina ORCID: orcid.org/0000-0001-8637-4609 1
Humanities and Social Sciences Communications volume 9 , Article number: 372 ( 2022 ) Cite this article
8103 Accesses
2 Citations
3 Altmetric
Metrics details
- Development studies
- Environmental studies
While rice studies are abundant, they usually focus on macro-level rice production and yield data, genetic diversity, cultivar varieties, and agrotechnological innovations. Moreover, many of these studies are either region-wide or concentrated on countries in the Global North. Collecting, synthesizing, and analyzing the different themes and topic areas in rice research since the beginning of the 21st century, especially in the Global South, remain unaddressed areas. This study contributes to filling these research lacunae by systematically reviewing 2243 rice-related articles cumulatively written by more than 6000 authors and published in over 900 scientific journals. Using the PRISMA 2020 guidelines, this study screened and retrieved articles published from 2001 to 2021 on the various topics and questions surrounding rice research in Indonesia and the Philippines—two rice-producing and -consuming, as well as emerging economies in Southeast Asia. Using a combination of bibliometrics and quantitative content analysis, this paper discusses the productive, relevant, and influential rice scholars; key institutions, including affiliations, countries, and funders; important articles and journals; and knowledge hotspots in these two countries. It also discusses the contributions of the social sciences, highlights key gaps, and provides a research agenda across six interdisciplinary areas for future studies. This paper mainly argues that an interdisciplinary and comparative inquiry of potentially novel topic areas and research questions could deepen and widen scholarly interests beyond conventional natural science-informed rice research in Indonesia and the Philippines. Finally, this paper serves other researchers in their review of other crops in broader global agriculture.
Similar content being viewed by others
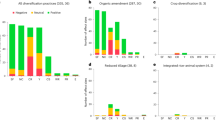
Agricultural diversification promotes sustainable and resilient global rice production
Xueqing He, Péter Batáry, … Thomas Cherico Wanger
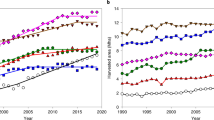

Southeast Asia must narrow down the yield gap to continue to be a major rice bowl
Shen Yuan, Alexander M. Stuart, … Patricio Grassini
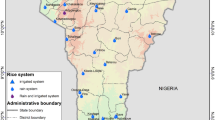
Characterization of rice farming systems, production constraints and determinants of adoption of improved varieties by smallholder farmers of the Republic of Benin
Yêyinou Laura Estelle Loko, Charlemagne D. S. J. Gbemavo, … François Sabot
Introduction
Rice feeds the majority of the world’s population and employs millions, especially in developing countries in the Global South (Muthayya et al., 2014 ). Rice consumption has increased globally over the last decade. Statista data show that, in the cropping year 2020/2021, the world population consumed about 504.3 million metric tons of rice, increasing from 437.18 million metric tons in 2008/2009 (Shabandeh, 2021 ). These data highlight the crop’s global contribution and importance, especially in realizing the Sustainable Development Goals (SDGs), the blueprint for global prosperity (Gil et al., 2019 ). The SDGs call for systems transformation, including in agriculture, guided by the principles of sustainability and equity, driven by the leave-no-one-behind aphorism, to address the root causes of perennial poverty and chronic hunger.
Pathologist M. B. Waite ( 1915 ) pointed out that the apparent indicator of progress in modern agriculture is the application of scientific research and the subsequent modification and improvement of farming systems based on those research. For example, the Green Revolution resulted in increased agricultural production in developing countries due to the transfer of agrotechnological innovations from countries in the Global North to countries in the Global South. Although, we acknowledge that this project came with a cost (Glaeser, 2010 ; Pielke and Linnér, 2019 ; Pingali, 2012 ).
Regional rice studies have proliferated in Europe (Ferrero and Nguyen, 2004 ; Kraehmer et al., 2017 ), the Americas (Singh et al., 2017 ), Africa (Zenna et al., 2017 ), the Asia Pacific (Papademetriou et al., 2000 ), and South Asia (John and Fielding, 2014 ). Country studies on rice production have also emerged in Australia (Bajwa and Chauhan, 2017 ), China (Peng et al., 2009 ), and India (Mahajan et al., 2017 ). Scholars have also systematically reviewed rice’s phytochemical and therapeutic potentials (Sen et al., 2020 ), quality improvements (Prom-u-thai and Rerkasem, 2020 ), and its role in alleviating the effects of chronic diseases and malnutrition (Dipti et al., 2012 ).
These extant studies, however, are limited on at least three fronts. First, their foci were on rice production, yield, and operational practices and challenges at the macro level. Second, there have been zero attempts at synthesizing this corpus since the 21st century. Third, there are also no attempts at examining the various rice research areas that scholars, institutions, and countries need to focus on, especially in developing country contexts, and their nexuses with the social sciences. This paper addresses these gaps by unpacking and synthesizing multiple rice studies conducted in the emerging Southeast Asian economies of Indonesia and the Philippines from 2001 to 2021. A focus on these developing countries matters since they are home to over 35 million rice farmers (IRRI, 2013 ).
We conducted our review from the Scopus database, using a combination of bibliometric and quantitative content analyses. Section “Results and discussions” reports our results, where we discuss (1) the most relevant and influential rice scholars and their collaboration networks; (2) the most rice research productive institutions, including author affiliations, their countries, and their research funders; and (3) the most significant articles and journals in rice research. This section also identifies 11 topic areas belonging to four major themes of importance for rice research in the two countries. Section “Contributions from and research agenda for the social sciences” provides a research agenda, where we identify and discuss the contributions of our review in terms of future work. Despite the preponderance of rice research in the last two decades and more in Indonesia and the Philippines, contributions from the social sciences remain marginal. Thus, in the section “Conclusion”, we conclude that emphasis is needed on expanding and maximizing the contributions of social scientists given the many opportunities available, especially for conducting interdisciplinary and comparative rice research in these Southeast Asian countries.
Review methods and analytical approach
We used bibliometric and quantitative content analyses to systematically categorize and analyze more than two decades of academic literature on rice in Indonesia and the Philippines. Bibliometric methods, also known as bibliometrics, have grown to be influential in evaluating various research fields and topic areas. Bibliometrics mushroomed because of the increasing availability of online databases and new or improved analysis software (Dominko and Verbič, 2019 ). Bibliometrics quantitatively and statistically analyze research articles using their bibliographic data, such as authors, affiliations, funders, abstracts, titles, and keywords. These data are analyzed to identify and assess the development, maturity, research hotspots, knowledge gaps, and research trends (Aria and Cuccurullo, 2017 ). For example, bibliometrics have been used in reviewing hydrological modeling methods (Addor and Melsen, 2019 ), business and public administration (Cuccurullo et al., 2016 ), and animals’ cognition and behavior (Aria et al., 2021 ).
This review article used bibliometrix , a machine-assisted program that offers multiple options and flexibility to map the literature comprehensively (Aria and Cuccurullo, 2017 ). We run this program using R Studio version 4.1.2 (2021-11-01; “Bird Hippie”) for its source code readability, understandability, and easy-to-do computer programming (Cuaton et al., 2021 ). We used bibliometrix in three critical analytical phases: (a) importing and converting data to R format, (b) identifying our dataset’s collaboration networks and intellectual and conceptual structures, and (c) processing, presenting, and analyzing our dataset. Bibliometrix, however, is unable to produce specific data that we want to highlight in this paper; examples of these are our coding criteria on interdisciplinarity and author gender, where such information was not captured in the articles’ bibliographic data in Scopus. We addressed these issues by conducting a quantitative content analysis (QCA) of our dataset. QCA is a method to record, categorize, and analyze textual, visual, or aural materials (Coe and Scacco, 2017 ). QCA has been applied in other reviews, such as in energy research development in the social sciences (Sovacool, 2014 ), the concepts of energy justice (Jenkins et al., 2021 ), and in examining agricultural issues in Botswana (Oladele and Boago, 2011 ) and Bangladesh (Khatun et al., 2021 ).
Search strategies
We constructed our dataset from the Scopus database, which we accessed via our institution’s online library on 14 November 2021. Scopus is a scientific database established in 2004 and owned by Elsevier Ltd. (Elsevier, 2021 ). We excluded other databases, such as Google Scholar, ScienceDirect, Web of Science, and EBSCO, suggesting one potential bias in our review (Waltman, 2016 ; Zupic and Čater, 2015 ). Our decision to exclusively use Scopus arises from two main reasons. First, the database has broader coverage than others, including the abovementioned (Falagas et al., 2008 ). Scopus includes new and emerging journals published in developing countries like Indonesia and the Philippines, our focus countries. Second, Scopus has a user-friendly interface and its search options allow researchers to flexibly explore its universe of indexed articles based on authors, institutions, titles, abstracts, keywords, and references (Donthu et al., 2021 ).
We followed the PRISMA 2020 Guideline (Preferred Reporting Items for Systematic reviews and Meta-Analyses) (Page et al., 2021 ) in our search for potential rice-related studies in Indonesia and the Philippines (see Fig. 1 ). We used the initial search string: “rice” AND “Indonesia*” OR “Philippine*” (asterisk or “*” was used as a wildcard search strategy) and limited the year coverage from 2001 to 2021. Our first round of searches resulted in 3846 documents (results as of 14 November 2021). We filtered these documents by including only peer-reviewed, full-text English articles on rice. We did not include any documents from the grey literature (e.g., news items, press releases, government or corporate reports), and other document types indexed in Scopus such as reviews, books, conference papers, errata, comments, editorials, and short reports.
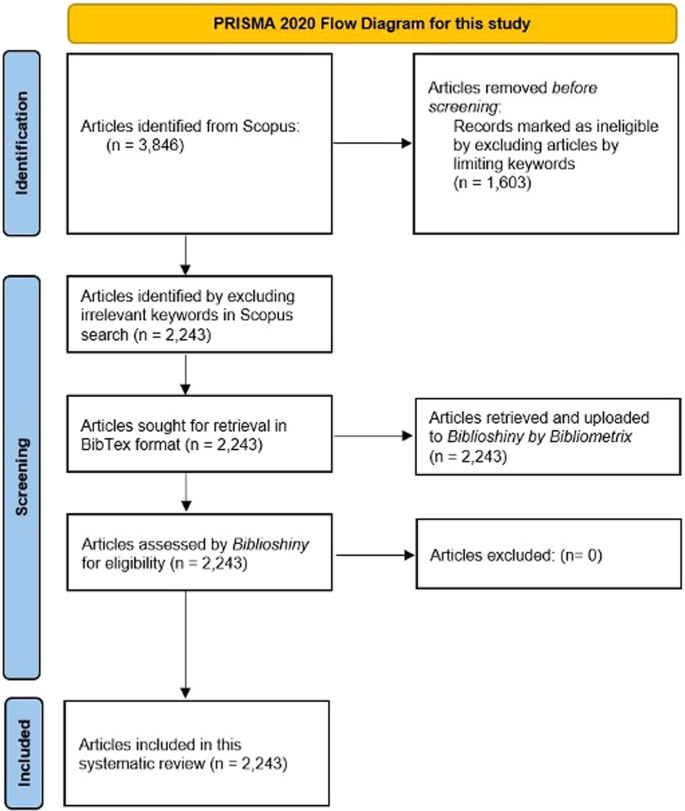
Our initial result of 3846 documents (results as of 14 November 2021) was filtered by including only peer-reviewed, full-text English articles on rice, resulting in 2243 eligible documents.
We also excluded articles with irrelevant keywords by using the following combined queries:
This resulted in 2243 eligible documents. We downloaded these documents as raw files in BibTex format and imported them to Biblioshiny , a web interface in Bibliometrix, where they were further filtered. Our verified final dataset comprises 2243 full-text English articles cumulatively written by 6893 authors and published across 909 journals (see Table 1 ).
Structure and analytical approach
We examined the authors’ profiles based on their gender, relevance in the study, and global impact. For gender, we coded them into ‘man,’ ‘woman,’ and ‘undetermined’ because some did not put enough information that helps in gender identification. We identified their gender by counter-checking their Scopus profiles to their verified accounts in Google Scholar, ResearchGate, Publons/Web of Science, or institutional profiles. We measured the authors’ relevance and impact against their (a) productivity, (b) citations, and (c) H-indices. We acknowledge, however, that some Filipino and Indonesian scholars, whose papers may not be indexed in Scopus, could also be prolific based on different parameters, but we excluded them. We proceeded to map the collaboration networks of these authors to identify “who works with whom on what.” A collaboration network illustrates nodes (circle shape) as authors and links (connecting lines) as co-authorships (Glänzel and Schubert, 2005 ).
Institutions, countries, funders
Following Sovacool ( 2014 ), we categorized the authors’ institutions into four: (1) University and research included authors who are researchers, instructors/lecturers/professors, other academic faculty from various non-university research think tanks, institutes, and national and local research centers; (2) Government consisted country or state departments, bureaus, ministries, and other government regulatory bodies; (3) Interest groups and NGOs included intergovernmental bodies, such as the United Nations Food and Agriculture Office (FAO) and international organizations like the International Rice Research Institute (IRRI) and Oxfam; and (4) Banking and finance encompassed players from the finance sector, including multilateral development banks such as the Asian Development Bank (ADB), World Bank, and the International Fund for Agricultural Development (IFAD). After coding and categorizing, we analyzed the authors’ institutional collaboration networks.
We identified the country’s productivity and coded them by global region based on their geographical location: (a) Asia, (b) Australia, New Zealand, and South Pacific, (c) Europe, (d) North America, (e) South America, and (f) Africa. We did this to show how various countries have been researching rice in Indonesia and the Philippines since the 21st century.
We then constructed a country collaboration map as a visual macro-representation of countries working together on rice research using these data. Bibliometrix, however, measured the country’s productivity based on the corresponding authors’ affiliations. We, therefore, noted two critical points here. First, many corresponding authors may have multiple institutional affiliations. For example, one corresponding author may belong to more than two affiliations (e.g., a corresponding Filipino author may have concurrent institutional affiliations in Japan, Australia, and New Zealand). Second, the corresponding authors may not necessarily be nationals of that country. Note that the unit of analysis is based on the corresponding authors’ institutional affiliations at the time of publication and not on their country/ies of citizenship or nationality. Despite these, our findings still provide insight into the macro-level productivity of countries conducting rice research in Indonesia and the Philippines.
We analyzed the funders using Scopus’ in-house Analytics Tool and determined their relevance based on the number of articles mentioning them in the Funding source or Acknowledgment section in the paper. We categorized the funders into six: (1) government (e.g., ministries, departments, or regulatory agencies), (2) research (e.g., research councils, research centers, and national academies), (3) foundations and non-government organizations (NGOs), (4) universities, (5) private companies and corporations, and (6) intergovernmental organizations/IGOs, including multilateral development banks.
Articles and journals
In terms of interdisciplinarity, we coded the articles as (a) interdisciplinary, (b) disciplinary, or (c) unidentified by using the authors’ department or division affiliation/s as a proxy to determine their disciplinary training. We coded an article as interdisciplinary if it belonged to any of the three criteria: (1) it had an author that had training or belonged to a department/division in at least two conventional disciplines (e.g., agriculture, anthropology, sociology, biology); (2) it had an author that had a self-identified interdisciplinary department (e.g., interdisciplinary division, sustainability, agriculture economics, etc.); or (3) it had at least two authors with different disciplinary training or expertise (e.g., business and economics; crop science and political science, etc.). We coded an article as disciplinary if its author/s had only belonged to one conventional department/division affiliation (e.g., Division of Agriculture, Department of Economics, Division of Environmental Science, etc.). On the other hand, we coded an article as undetermined when the authors had only indicated the name of their institutions or did not indicate their departmental or division affiliations (e.g., only the University of the Philippines, IRRI, Universitas Gadja Mada, etc.).
We examined the articles based on their local relevance and global influence. Bibliometrix measured the articles’ relevance based on their “local citations” or citations received from the 2243 articles of our sample dataset. We did this to determine which papers are considered relevant by authors studying various areas of rice research in Indonesia and the Philippines. Global influence is measured based on the articles’ citations from the global research community or other scientific works beyond our sample dataset. We also conducted a co-citation analysis of the cited references. Co-citation is the frequency by which articles cite together two or more articles relevant to the topic areas of inquiry (Aria and Cuccurullo, 2017 ). Bibliometrix had identified some co-cited articles published before our timeline of interest (i.e., pre-2001) which provide scholars with a more profound understanding of rice research in the two countries.
On the other hand, Bibliometrix identified the most relevant journals based on the number of papers the journals had published and the local citations of the articles. These data guide readers and researchers on which journals to look for on rice studies in Indonesia and the Philippines.
Knowledge hotspots
Bibliometrix creates a thematic map that allows researchers to identify which study areas have been adequately explored and which areas need further investigation or re-investigation to identify knowledge hotspots and research gaps (Aria and Cuccurullo, 2017 ). Della Corte et al. ( 2019 , pp. 5–6) discussed the major themes in Bibliometrix in the following:
“Themes in the lower-right quadrant are the Basic Themes , characterized by high centrality and low density. These themes are considered essential for a research field and concerned with general topics across different research areas.
Themes in the upper-right quadrant are the Motor Themes , characterized by high centrality and density. Motor themes are considered developed and essential for the research field.
Themes in the upper-left quadrant are the highly developed and isolated themes or Niche Themes . They have well-developed internal links (high density) but unimportant external links, which could be interpreted as having limited importance for the field (low centrality).
Themes in the lower-left quadrant are known as Emerging or Declining Themes . They have low centrality and density, making them weakly developed and marginal.”
Contributions from and research agenda for the social sciences
As interdisciplinary environmental and social scientists, we also focused our review on the social studies of rice in the two countries. This section highlighted the gaps between the natural and the social sciences in rice research and advanced a research agenda for interdisciplinary and comparative social scientists.
Limitations
As in any systematic review, we acknowledge certain limitations to our work. We discuss four of these.
First, to keep a certain level of reliability, we focused only on peer-reviewed full-length research articles written in the English language and indexed in the Scopus database. Therefore, we may have excluded some relevant articles, including those written in Filipino, Indonesian, and other local or indigenous languages and published in local or international journals but are not indexed in Scopus. Our review also excluded conference papers, commentaries, book reviews, book chapters, conference reviews, data papers, errata, letters, notes, and non-academic publications like policy briefings, reports, and white papers.
Second, in our quantitative content analysis, we acknowledge the highly cis-heteronormative approach we used in coding the author’s gender as “man” or “woman.” We identified these genders from the names and pictures of the authors in their verified Scopus, Publons/ Web of Science, and institutional profiles. It is not our deliberate intention to neglect the varying genders of researchers and scientists beyond the traditional binary of man or woman.
Third, we recognize that our analysis cannot directly identify how much each funder provided as the unit of analysis in bibliometrix may depend on how prolific researchers were in publishing articles despite smaller funds. For instance, one research project supported by Funder A with US$1 million may have published only one article based on their project design or the funder's requirement. Since the authors published only one paper from this project, the data could show that Funder A only funded one research. Another research project, supported by Funder B, with only US$300,000 in funding, may have published more than five papers; therefore, more articles counted as funded by Funder B. This issue is not within the scope of our review.
Lastly, it should be noted that the future research works we discussed were highly influenced by our research interests and the general overview of the literature, and thus neither intend to cover nor aim to discuss the entire research topics that other scholars could study.
Despite these limitations, we strongly argue that our review provided relevant insights and proposed potentially novel topic areas and research questions for other scholars to explore, especially social scientists, in deepening and widening rice research in Indonesia and the Philippines. To end, we hope that researchers heed our call to conduct more interdisciplinary and comparative rice-related studies in these two emerging Southeast Asian countries.
Results and discussions
Our dataset comprises 2243 peer-reviewed journal articles cumulatively written by 6893 authors who cited around 80,000 cumulative references. The average annual publications from 2001 to 2013 were only 57 papers but elevated to hundreds beginning in 2014 (Fig. 2 ).
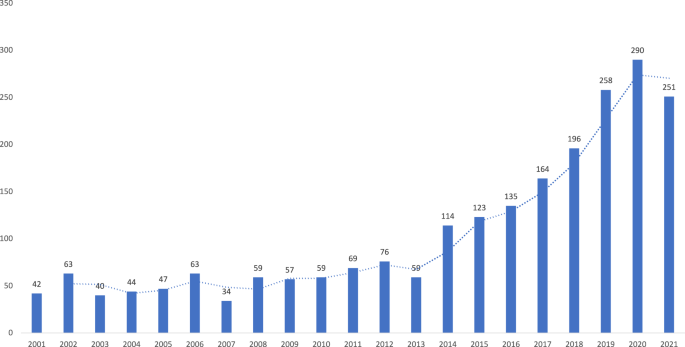
The average number of annual publications on rice research in Indonesia and the Philippines from 2001 to 2013 was only 57 papers but elevated to hundreds beginning in 2014.
Of the 159 authors, one had a duplicate profile; thus, we identified 158 authors publishing on rice studies; the majority (66%) are men. The top 50 most prolific scholars produced a little over 25% (567 articles) of the total articles. Australian ecologist Finbarr Horgan topped this list ( n = 21), followed by Bas Bouman and Grant Singleton—each with 20 articles. The top 10 authors with the highest number of publications have affiliations with the IRRI, the University of the Philippines, the University of Gadjah Mada, and the Philippine Rice Research Institute (PhilRice). For the full list of prolific scholars with at least 10 articles published, see Supplementary Table 1 .
In terms of the authors with the most local citations, although Finbarr Horgan has the most documents, Johan Iskandar ( n = 36 citations) from the Universitas Padjadjaran, who studies rice genetic diversity, is the most cited. Local citations refer to the citations received by authors from our sample dataset of 2243 articles. Muhidin Muhidin from the Universitas Halu Oleo and Ruhyat Partasasmita from the Universitas Padjadjaran, followed him with 30 and 28 local citations, respectively. Common to these three authors are their Biology background/expertise and interest in rice genetic diversity. To check the top 20 most locally cited scholars, refer to Supplementary Table 2 .
The H-index is the author-level measure of publications’ productivity and citation impacts (Hirsch, 2005 ). Bas Bouman (H-index = 18) leads the top 10 scholars among rice-related researchers in Indonesia and the Philippines. Yoshimichi Fukuta (H index = 13) and Shaobing Peng (H index = 13) followed him. These three authors are affiliated with or have collaborated with the IRRI. To check the top 10 scholars with the highest H-indices, refer to Supplementary Table 3 .
Figure 3 reveals the top 80 authors who collaborate across eight major clusters of rice research. The Red cluster shows Finbarr Horgan as the most prominent author with at least four significant collaborators in pest management, specifically on rice stemborers (Horgan et al., 2021 ), anthropods’ biodiversity in tropical rice ecosystems (Horgan et al., 2019 ), and virulence adaptations of rice leafhoppers (Horgan et al., 2018 ). In the Purple Cluster, Yoshimichi Fukuta has multiple publications with at least six collaborators in the study of rice blast (Ebitani et al., 2011 ; Kadeawi et al., 2021 ; Mizobuchi et al., 2014 ). In the Brown cluster, Bernard Canapi from the IRRI has collaborated with at least five scholars in the study of rice insect pest management (Cabasan et al., 2019 ; Halwart et al., 2014 ; Litsinger et al., 2011 ), farmers’ preference for rice traits (Laborte et al., 2015 ), and the drivers and consequences of genetic erosion in traditional rice agroecosystems in the Philippines (Zapico et al., 2020 ). The Gray cluster shows that Siti Herlinda has collaborated with at least four scholars to study anthropods in freshwater swamp rice fields (Hanif et al., 2020 ; Herlinda et al., 2020 ) and the benefits of biochar on rice growth and yield (Lakitan et al., 2018 ).
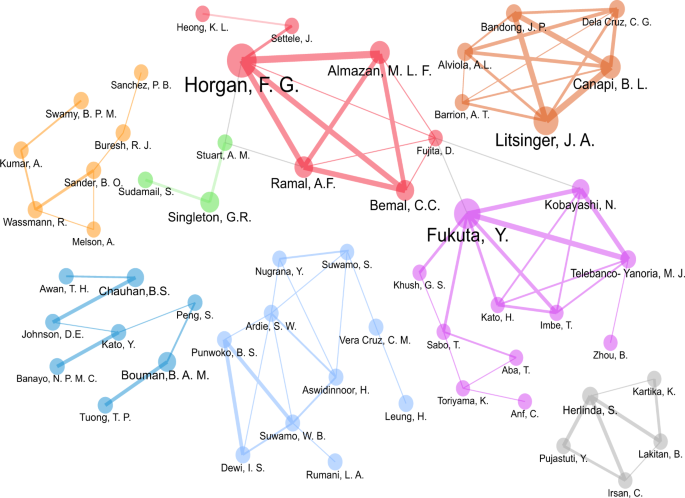
The authors’ collaboration networks show eight major clusters of rice research in Indonesia and the Philippines.
Institutions
Author affiliations.
In terms of institutional types, Fig. 4 shows that most rice researchers in Indonesia and the Philippines have affiliations with “University and research.” Figure 5 shows the top 20 institutions in terms of research productivity led by the IRRI, the University of the Philippines System, the PhilRice, the Institute Pertanian Bogor/IPB University, and the University of Gadja Mada. These 20 institutions produced 66% of the articles in our dataset.
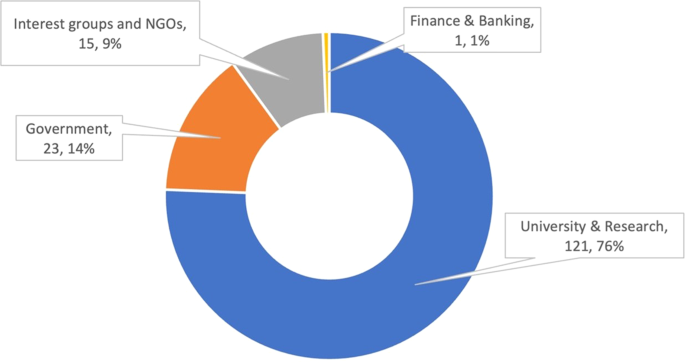
The majority of rice researchers in Indonesia and the Philippines have affiliations with “University and research”.
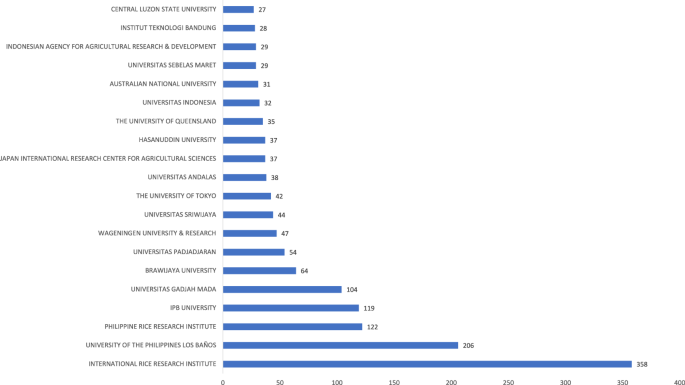
The top 5 most productive institutions in terms of rice research in Indonesia and the Philippines are the IRRI, the University of the Philippines System, the PhilRice, the Institute Pertanian Bogor/IPB University, and the University of Gadja Mada.
Scholars affiliated with the IRRI have written the most papers (at least 19% or 358 articles) in our dataset. The range of topics covers both regional and country studies. Some regional examples include the drivers of consumer demand for packaged rice and rice fragrance in South and Southeast Asia (Bairagi et al., 2020 ; Bairagi, Gustafson et al., 2021 ). Country studies, for example, include an investigation of rice farming in Central Java, Indonesia (Connor et al., 2021 ), the cultural significance of heirloom rice in Ifugao in the Philippines (Bairagi, Custodio et al., 2021 ), and the distributional impacts of the 2019 Philippine rice tariffication policy (Balié and Valera, 2020 ).
The University of the Philippines System, with rice scholars affiliated with their campuses in Los Baños, Diliman, Mindanao, and Manila, produced the next largest number of papers (more than 200 or 10%) on topics about rice pests and parasites (Horgan et al., 2019 , 2021 ; Vu et al., 2018 ), weed control (Awan et al., 2014 , 2015 ; Fabro and Varca, 2012 ), and climate change impacts on rice farming (Alejo and Ella, 2019 ; Ducusin et al., 2019 ; Gata et al., 2020 ). Social studies of rice conducted by the University of the Philippines researchers include indigenous knowledge on climate risk management (Ruzol et al., 2020 , 2021 ), management options in extreme weather events (Lopez and Mendoza, 2004 ), agroecosystem change (Aguilar et al., 2021 ; Neyra-Cabatac et al., 2012 ), and the development and change over time of rice production landscapes (Santiago and Buot, 2018 ; Tekken et al., 2017 ).
PhilRice, a government-owned corporation under the Department of Agriculture (Official Gazette of the Philippines, 2021 ), is the third most prolific rice research-producing institution (122 papers) on topics ranging from nematodes or rice worms (Gergon et al., 2001 , 2002 ) and arthropods (invertebrates found in rice paddies) (Dominik et al., 2018 ), hybrid rice (Perez et al., 2008 ; Xu et al., 2002 ), alternate wetting-and-drying technology (Lampayan et al., 2015 ; Palis et al., 2017 ), and community development strategies on rice productions (Romanillos et al., 2016 ).
The IPB University, a public agrarian university in Bogor, Indonesia, investigates rice productivity and sustainability (Arif et al., 2012 ; Mucharam et al., 2020 ; Setiawan et al., 2013 ), irrigation (Nugroho et al., 2018 ; Panuju et al., 2013 ), extreme weather events such as drought (Dulbari et al., 2021 ), floods (Wakabayashi et al., 2021 ), and emerging social issues such as food security (Putra et al., 2020 ), land-use change (Chrisendo et al., 2020 ; Munajati et al., 2021 ), and sustainability (Mizuno et al., 2013 ). This university has 23 research centers, including those which focus on environmental research; agricultural and village development; engineering applications in tropical agriculture; Southeast Asian food and agriculture; and agrarian studies.
Universitas Gadja Maja in Yogyakarta, Indonesia, hosts 21 research centers, including its Agrotechnology Innovation Centre. It carries out research incubation and development activities, product commercialization, and integration of agriculture, animal husbandry, energy, and natural resources into a sustainable Science Techno Park. Some of their published studies focused on drought-tolerant rice cultivars (Salsinha et al., 2020 , 2021 ; Trijatmiko et al., 2014 ), farmers’ technical efficiency (Mulyani et al., 2020 ; Widyantari et al., 2018 , 2019 ), systems of rice intensification (Arif et al., 2015 ; Syahrawati et al., 2018 ), and climate change adaptation (Ansari et al., 2021 ).
In terms of institutional collaboration, the IRRI tops the list with at least eleven collaborators (Fig. 6 ), including the Japan International Center for Agricultural Sciences, the PhilRice, the University of the Philippines System, and the Indonesian Center for Rice Research.
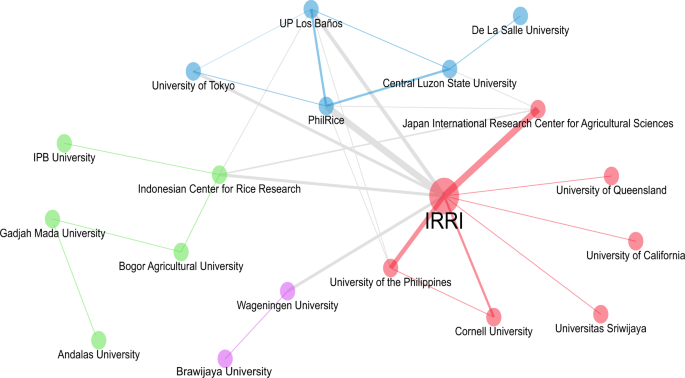
The IRRI, as an international organization focused on many aspects of rice, is not surprising to have the greatest number of institutional collaborators ( n = 11 institutions).
Rice studies’ authors are from at least 79 countries; the majority of them are working in Asia (79%), followed by Europe (13%) and North America (9%). At least 90% of rice scholars are in Indonesia, and more than 51% have affiliations in the Philippines, followed by Japan, the USA, and China. For the list of the top 20 most productive countries researching rice in Indonesia and the Philippines, see Supplementary Table 4 . Figure 7 shows a macro-level picture of how countries have collaborated on rice-related projects in Indonesia and the Philippines since 2001, suggesting that rice research in both countries has benefited from international partnerships.
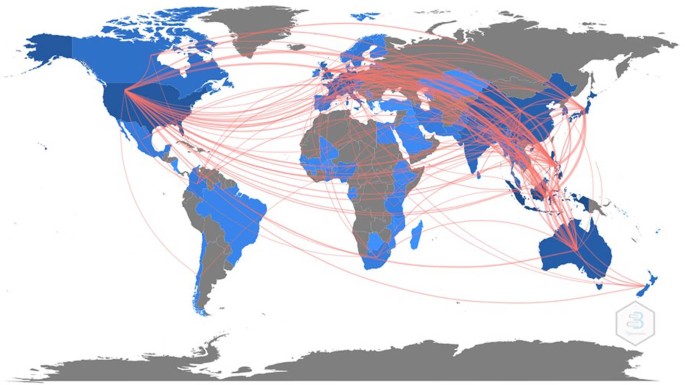
A macro-level picture of how countries have collaborated on rice-related projects in Indonesia and the Philippines since 2001. It suggests that rice research in both countries has benefited from international partnerships.
Only around 47% (1050 studies) of our dataset acknowledged their funding sources, where most received financial support either from governments (45%), research (27%), or university funders (16%) (Fig. 8 ). To see the top 15 funders that supported at least 10 rice-related research projects in Indonesia and the Philippines from 2001 to 2021, refer to Supplementary Table 5 . Of over 150 rice research funders, Indonesia’s Ministry of Education, Culture, and Research (formerly the Ministry of Research and Technology) funded ~6% (62 out of 1050 studies). The Japan Society for the Promotion of Science and Japan’s Ministry of Education, Culture, Sports, Science and Technology came in as the second and third largest funders, respectively.
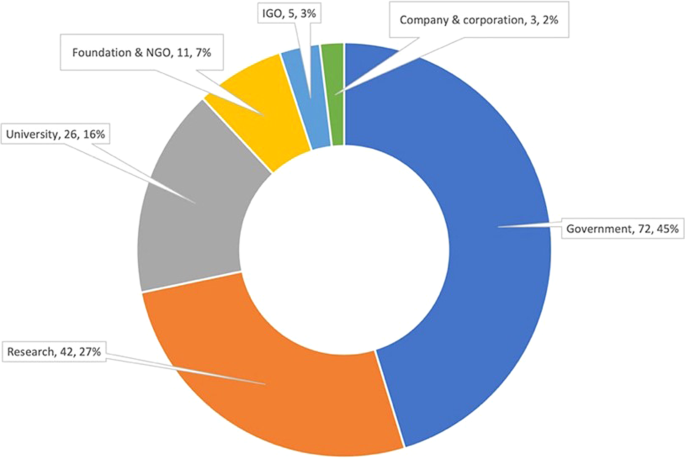
The majority of rice research projects in Indonesia and the Philippines were funded by governments (45%), research (27%), and university institutions (16%).
Half of all articles in the dataset were borne out of interdisciplinary collaboration. More than a quarter of the articles, however, were unidentified, showing an apparent undercount of the total number of disciplinary collaborations. Most of these collaborative pieces of work (~61%) belong to the natural science subject areas of agricultural and biological sciences; biochemistry, genetics, and molecular biology; and environmental science (see Table 2 ). Note that the cumulative number of articles in Table 2 is more than the total number of the sample dataset since an article may belong to multiple subject areas as indicated by its authors in Scopus. Less than 9% (354) of all papers were written by social scientists, highlighting their marginal contribution to rice research. The social studies of rice can increase our understanding of the many facets of rice production, including their socio-political, economic, and cultural aspects.
Our review shows that there are 10 major networks of rice research co-citations (Fig. 9 ). The papers by Bouman et al. ( 2005 ), Bouman et al. ( 2007 ), Bouman and Tuong ( 2001 ), and Tuong and Bouman ( 2003 ) were co-cited by scholars studying the relationship between water scarcity management vis-à-vis rice growth and yield (the purple cluster in Fig. 9 ). Papers by Yoshida et al. ( 2009 ), De Datta ( 1981 ), and Peng et al. ( 1999 ) were co-cited by scholars researching the genetic diversity, yield, and principles and practices of rice production in Indonesia (the red cluster in Fig. 9 ). Papers by Ou ( 1985 ), Mackill and Bonman ( 1992 ), Sambrook et al. ( 1989 ), Kauffman et al. ( 1973 ), Iyer and McCouch ( 2004 ), and Mew ( 1987 ) were considered essential references in studying rice diseases (blue cluster in Fig. 9 ). The top-cited article on rice research in Indonesia and the Philippines, based on their overall global citations, is a study on water-efficient and water-saving irrigation (Belder et al., 2004 ). This study detailed alternative options for typical water management in lowland rice cultivation, where fields are continuously submerged, hence requiring a continuous large amount of water supply (Belder et al., 2004 ). Global citations refer to the citations received by the articles within and beyond our sample dataset of 2243 articles. To see the top 10 most globally cited articles on rice research in Indonesia and the Philippines, refer to Supplementary Table 6 .
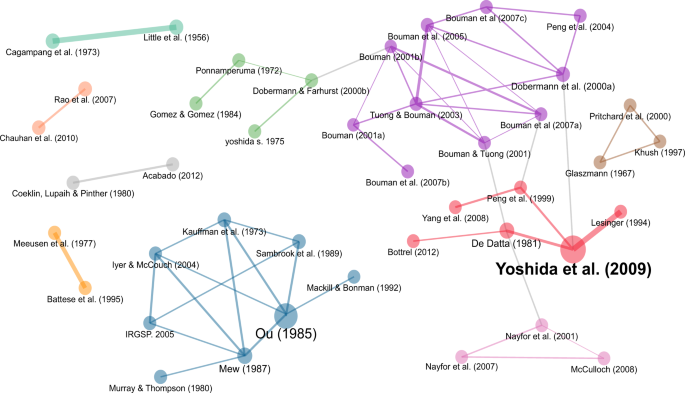
There are 10 major networks of rice research co-citations in Indonesia and the Philippines.
The journal Biodiversitas: Journal of Biological Diversity published the most number of papers on rice research in the two countries. Biodiversitas publishes papers “dealing with all biodiversity aspects of plants, animals, and microbes at the level of gene, species, ecosystem, and ethnobiology” (Biodiversitas, 2021 ). Following its indexing in Scopus in 2014, Biodiversitas has increasingly published rice studies, most of which were authored by Indonesian researchers. To see the top 10 most relevant journals for rice research in Indonesia and the Philippines based on the number of documents published since 2001, refer to Supplementary Table 7 .
Based on their local citations, the journals Field Crops Research , Theoretical & Applied Genetics , and Science are the most relevant. Field Crops Research focuses on crop ecology, crop physiology, and agronomy of field crops for food, fiber, feed, medicine, and biofuel. Theoretical and Applied Genetics publishes original research and review articles in all critical areas of modern plant genetics, plant genomics, and plant biotechnology. Science is the peer-reviewed academic journal of the American Association for the Advancement of Science and one of the world’s top academic journals. To see the top 30 most relevant journals for rice research in Indonesia and the Philippines based on the number of local citations, refer to Supplementary Table 8 .
The most used keywords found in 2243 rice research papers published between 2001 and 2021 in Indonesia and the Philippines are food security, climate change, drought, agriculture, irrigation, genetic diversity, sustainability, technical efficiency, and production (Fig. 10 ). We found 11 clusters across four significant themes of rice research in these countries (Fig. 11 ).
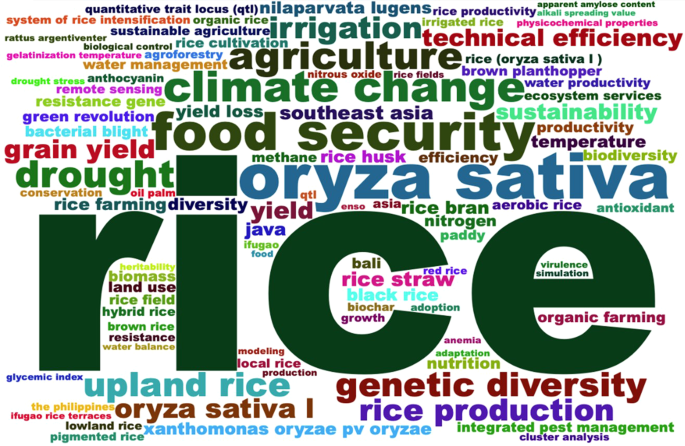
The most used keywords found in 2243 rice research papers published between 2001 and 2021 in Indonesia and the Philippines are food security, climate change, drought, agriculture, irrigation, genetic diversity, sustainability, technical efficiency, and production.
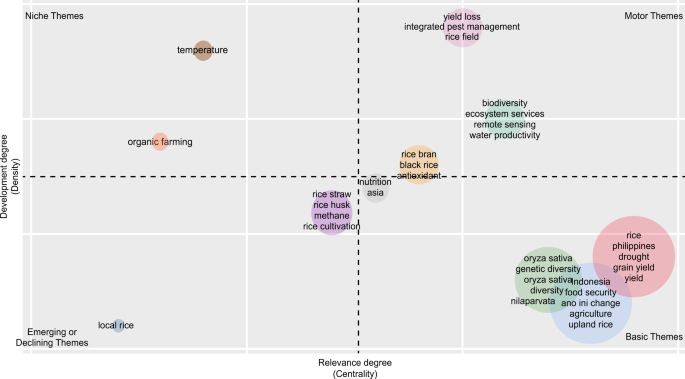
There are four major themes composed of 11 clusters of rice research in Indonesia and the Philippines since 2001.
Basic themes
We identified four major clusters under ‘basic themes’ (refer to Fig. 11 ):
The Red Cluster on studies in the Philippines related to rice yield and productivity, drought, nitrogen, the Green Revolution, and the use and potential of biomass;
The Blue Cluster on studies in Indonesia related to food security, climate change, agriculture, upland rice, irrigation, technical efficiency, and sustainability vis-à-vis rice production;
The Green Cluster on rice genetic diversity, bacterial blight diseases, resistant rice genes, aerobic rice, and brown planthoppers; and
The Gray Cluster on the nutritional aspects of rice, including studies on biofortified rice cultivars.
Agriculture suffers from climate change impacts and weather extremes. Rice researchers in Indonesia and the Philippines are identifying drought-tolerant rice cultivars that can produce high yields in abiotic stress-prone environments (Afa et al., 2018 ; Niones et al., 2021 ). These hybrid cultivars are vital for increasing rice productivity, meeting production demand, and feeding the growing Filipino and Indonesian populations (Kumar et al., 2021 ; Lapuz et al., 2019 ). Researchers have also looked at alternative nutrient and water management strategies that farmers can use, especially those in rainfed lowland areas during drought (Banayo, Bueno et al., 2018 ; Banayo, Haefele et al., 2018 ). There were also studies on the socio-cultural dynamics under which farmers adapt to droughts, such as how past experiences of hazards influence farmers’ perceptions of and actions toward drought (Manalo et al., 2020 ).
Motor themes
We identified three significant clusters of ‘motor themes’ (refer to Fig. 11 ):
The Pink Cluster on yield loss and integrated pest management of rice fields;
The Blue-Green Cluster on biodiversity, ecosystem services, remote sensing, and water productivity; and
The Orange Cluster on the antioxidant properties of rice bran and black rice.
In both countries, pests, including weeds (Awan et al., 2014 , 2015 ), insects (Horgan et al., 2018 , 2021 ), and rodents (Singleton, 2011 ; Singleton et al., 2005 , 2010 ), have significant impacts on yield loss in rice production and human health. To address these, many farmers have embraced chemical-heavy pest management practices to prevent yield loss and increase economic benefits. Pesticides began their use in Indonesia and the Philippines and rapidly expanded from the 1970s to the 1980s (Resosudarmo, 2012 ; Templeton and Jamora, 2010 ). However, indiscriminate use of pesticides caused an ecological imbalance that exacerbated pest problems (Templeton and Jamora, 2010 ) and contributed to farmers’ acute and chronic health risks (Antle and Pingali, 1994 ; Pingali and Roger, 1995 ).
Integrated pest management was introduced, applied, and studied in both countries to address these issues. This approach combines multiple compatible pest control strategies to protect crops, reduce pesticide use, and decrease farming costs (Gott and Coyle, 2019 ). For example, Indonesia’s 1989 National Integrated Pest Management Program trained hundreds of thousands of farmers and agricultural officials about its principles, techniques, and strategies (Resosudarmo, 2012 ). In the Philippines, the government of then-President Fidel V. Ramos (1992–1996) prohibited using hazardous pesticides and instituted a “multi-pronged approach to the judicious use of pesticides” (Templeton and Jamora, 2010 , p. 1). President Ramos’ suite of policies included deploying Integrated Pest Management “as a national program to encourage a more ecologically sound approach to pest control” (Templeton and Jamora, 2010 , p. 1). This pesticide policy package benefited the Philippine government in terms of private health costs avoided (Templeton and Jamora, 2010 ).
To address weed problems, farmers traditionally use manual weeding, a labor-intensive practice. However, as labor costs for manual weeding increased, herbicide use became economically attractive to farmers (Beltran et al., 2012 ). Herbicide experiments were made to address common rice weeds including barnyard grass ( Echinochloa crus-galli ) (Juliano et al., 2010 ), crowfoot grass ( Dactyloctenium aegyptium ) (Chauhan, 2011 ), three-lobe morning glory ( Ipomoea triloba ) (Chauhan and Abugho, 2012 ), and jungle rice ( Echinochloa colona ) (Chauhan and Johnson, 2009 ). Knowledge gained from these experiments contributed to the development of integrated weed management strategies.
Yet, many factors come into play when farmers decide to use herbicides. Beltran et al. ( 2013 ) reported that farmers’ age, household size, and irrigation use are significant determinants of adopting herbicides as an alternative to manual weeding. Beltran et al. ( 2013 ) further showed that economic variables, like the price of the herbicide, household income, and access to credit, determined farmers’ level of herbicide use (Beltran et al., 2013 ). Their study highlights the complex decision-making process and competing factors affecting weed management in the Philippines.
Apart from weeds, insects, like brown planthoppers ( Nilaparvata lugens ) and green leafhoppers ( Cicadella viridis ) and their accompanying diseases, affect rice production. In Java, Indonesia, Triwidodo ( 2020 ) reported a significant influence between the insecticide use scheme and the brown planthopper ( Nilaparvata lugens ) attack rates in rice fields. Brown planthopper attacks increased depending on the frequency of pesticide application, their varieties, and volume (Triwidodo, 2020 ). In the Philippines, Kim and colleagues ( 2019 ) developed a rice tungro epidemiological model for a seasonal disaster risk management approach to insect infestation.
Some social studies of integrated pest management included those that looked at the cultural practices that mitigate insect pest losses (Litsinger et al., 2011 ) and farmers’ knowledge, attitudes, and methods to manage rodent populations (Stuart et al., 2011 ). Other social scientists evaluated the value of amphibians as pest controls, bio-monitors for pest-related health outcomes, and local food and income sources (Propper et al., 2020 ).
Niche themes
We identified two ‘niche themes’ consisting of studies related to (a) temperature change and (b) organic rice production (refer to Fig. 11 ). Temperature change significantly affects rice farming. In the Philippines, Stuecker et al. ( 2018 ) found that El Niño-induced soil moisture variations negatively affected rice production from 1987–2016. According to one experiment, high night temperature stress also affect rice yield and metabolic profiles (Schaarschmidt et al., 2020 ). In Indonesia, a study suggests that introducing additional elements, such as Azolla, fish, and ducks, into the rice farming system may enhance rice farmers’ capacity to adapt to climate change (Khumairoh et al., 2018 ). Another study produced a rainfall model for Malang Regency using Spatial Vector Autoregression. This model is essential as rainfall pattern largely determines the cropping pattern of rice and other crops in Indonesia (Sumarminingsih, 2021 ).
Studies on organic rice farming in the Philippines include resource-poor farmers’ transition from technological to ecological rice farming (Carpenter, 2003 ) and the benefits of organic agriculture in rice agroecosystems (Mendoza, 2004 ). Other studies on organic rice focused on its impacts on agricultural development (Broad and Cavanagh, 2012 ) and climate resilience (Heckelman et al., 2018 ). In Indonesia, Martawijaya and Montgomery ( 2004 ) found that the local demand for organic rice produced in East Java was insufficient to generate revenue enough to cover its production costs. In West Java, Komatsuzaki and Syuaib ( 2010 ) found that organic rice farming fields have higher soil carbon storage capacity than fields where rice is grown conventionally. In Bali, farmers found it challenging to adopt organic rice farming vis-à-vis the complex and often contradictory and contested administration of the Subaks (MacRae and Arthawiguna, 2011 ) and the challenges they have to confront in marketing their produce (Macrae, 2011 ).
Emerging or declining themes
We identified two clusters of ‘emerging/declining themes’ or areas of rice research that are weakly developed and marginal (refer to Fig. 11 ). The Purple Cluster (emerging) studies rice straw, rice husk, methane, and rice cultivation, while the Light Blue Cluster (declining) pertains to local rice research.
In this section, we present and discuss the contributions of the social sciences, highlight key gaps, and provide a research agenda across six interdisciplinary areas for future studies. In Table 3 , we summarized the various topic areas that other scholars could focus on in their future studies of rice in Indonesia and the Philippines.
Economic, political, and policy studies
Political scientist Ernest A. Engelbert ( 1953 ) was one of the earliest scholars to summarize the importance of studying agricultural economics, politics, and policies. Engelbert ( 1953 ) identified three primary reasons scholars and laypeople alike need to understand the nature of political processes in agriculture. First, the rapid change and highly contested political environment where agriculture operates often places agriculture last on national policy agenda. Second, the formulation of agricultural policies intersects with contemporary national and economic contexts by which these policies revolve. Third, understanding the political processes around agriculture can help avoid political pressures and machinations aimed at undermining agricultural development.
Politics play a crucial role in better understanding rice- and agriculture-related policies, their evolution, dynamics, challenges, developments, and futures. Grant ( 2012 , p. 271) aptly asks, “Who benefits [from government policies, regulations, and programs]?” . Knowing, understanding, and answering this question is crucial since policymaking is a highly contested process influenced and negotiated not only by farmers and decision-makers but also by other interest groups, such as people’s organizations and non-government organizations. On the other hand, understanding macro- and micro-economic government arrangements come hand-in-hand in analyzing how policies impact farmers and consumers. Using tariffs as an example, Laiprakobsup ( 2014 , p. 381) noted the effects of government interventions in the agrarian market:
“… when the government implements consumer subsidy programs by requiring the farmers to sell their commodities at a cheaper price, it transfers the farmers’ incomes that they were supposed to earn to the consumers. Moreover, the government transfers tax burdens to the farmers via export taxes in that the agricultural industry is likely to purchase the farmers’ commodities as cheaply as possible in order to make up for its cost.”
The two countries have compelling economic, political, and policy-oriented rice studies. Some examples of this type of research in the Philippines are the following. Intal and Garcia ( 2005 ) argued that the price of rice had been a significant determinant in election results since the 1950s. Fang ( 2016 ) analyzed how the Philippines’ colonial history bolstered an oligarchy system, where landed elite politicians and patronage politics perpetuated corruption to the detriment of rice farmers. Balié and Valera ( 2020 ) examined rice trade policy reforms’ domestic and international impacts. San Juan ( 2021 ) contends that the 2019 Rice Tariffication Law of the Philippines only encouraged the country to rely on imports and failed to make the local rice industry more competitive.
In Indonesia, some political studies on rice production are the following. Putra et al. ( 2020 ) analyzed how urbanization affected food consumption, food composition, and farming performance. Noviar et al. ( 2020 ) provided evidence that households in the rice sub-sector have achieved an insufficient level of commercialization in their rice production. Rustiadi et al. ( 2021 ) investigated the impacts of land incursions over traditionally rice farming regions due to Jakarta’s continuous expansion. Satriawan and Shrestha ( 2018 ) evaluated how Indonesian households participated in the Raskin program, a nationwide rice price subsidy scheme for the poor. Misdawita et al. ( 2019 ) formulated a social accounting matrix and used a microsimulation approach to assess the impacts of food prices on the Indonesian economy.
Future work
Social science researchers could further explore and compare the local, regional, and national similarities and differences of the abovementioned issues or conduct novel research related to land-use change, land management, urbanization, food and agricultural policies, trade policies, irrigation governance, and price dynamics. Comparative social studies of rice could also lead to meaningful results. As social policy scholar Linda Hantrais noted:
“Comparisons can lead to fresh, exciting insights and a deeper understanding of issues that are of central concern in different countries. They can lead to the identification of gaps in knowledge and may point to possible directions that could be followed and about which the researcher may not previously have been aware. They may also help to sharpen the focus of analysis of the subject under study by suggesting new perspectives.” (Hantrais, 1995 , p. n/a).
Sociological, anthropological, and cultural studies
Biologists dominated agricultural research until the mid-1960s (Doorman, 1991 ). Agriculture, in other words, was no social scientist’s business. However, this situation gradually changed when governments and scholars realized the long-term impacts of the Green Revolution from the 1950s to the 1980s, which underscores that the development, transfer, and adoption of new agrotechnology, especially in developing countries, is driven not only by techno-biological factors but also by the socio-economic, political, and cultural realities under which the farmers operate. Since then, sociologists, anthropologists, and cultural scholars have become indispensable in answering the “how”, “what”, and “why” agrarian communities follow, adopt, utilize, or, in some cases, prefer local/traditional production technologies over the technological and scientific innovations developed by engineers, biologists, geneticists, and agriculturists. Nyle C. Brady, a soil scientist and the former Director-General of the IRRI pointed out:
“… we increasingly recognize that factors relating directly to the farmer, his family, and his community must be considered if the full effects of agricultural research are to be realized. This recognition has come partly from the participation of anthropologists and other social scientists in interdisciplinary teams … during the past few years.” (IRRI, 1982 ).
Since the late 19th century, many rice studies have tried to answer the roles of social scientists in agricultural research. Social sciences have contributed to agricultural research in many ways, especially regarding technology adoption by farmers (DeWalt, 1985 ; Doorman, 1990 ). Doorman ( 1991 , p. 4) synthesized these studies and offered seven roles for sociologists and anthropologists in agricultural research as follows:
“Accommodator of new technology, ex-post and ex-ante evaluator of the impact of new technology, an indicator of the needs for new technology, translator of farmer’s perceptions, broker-sensitizer, adviser in on-farm research, and trainer of team members from other disciplines.”
Social studies of rice are especially critical in Indonesia and the Philippines—home to hundreds of Indigenous cultural communities and Indigenous peoples (Asian Development Bank, 2002 ; UNDP Philippines, 2010 ). Regardless of the highly contested debates surrounding “indigeneity” or “being indigenous,” especially in Indonesia (Hadiprayitno, 2017 ), we argue that Indigenous cultural communities and Indigenous peoples have similarities (i.e., they are often farming or agrarian societies) but also recognize their differences and diversity in terms of their farming practices, beliefs, traditions, and rituals. These socio-cultural factors and human and non-human interactions influence rice production; thus, these differences and diversity bring front-and-center the importance of needs-based, community-driven, and context-sensitive interventions or projects for rice farming communities. These are research areas best explored by sociologists, anthropologists, and cultural scholars.
Today, agriculture’s sociological, anthropological, and cultural research have gone beyond the classic technology adoption arena. In Indonesia, studies have explored farmers’ technical efficiency in rice production (e.g., Muhardi and Effendy, 2021 ), the similarities and differences of labor regimes among them (e.g., White and Wijaya, 2021 ), the role of social capital (e.g., Salman et al., 2021 ), and the reciprocal human–environmental interactions in the rice ecological system (e.g., Sanjatmiko, 2021 ). Disyacitta Nariswari and Lauder ( 2021 ) conducted a dialectological study to examine the various Sundanese, Javanese, and Betawi Malay words used in rice production. Rochman et al. ( 2021 ) looked into the ngahuma (planting rice in the fields) as one of the inviolable customary laws of the Baduy Indigenous cultural community in Banten, Indonesia.
In the Philippines, Balogbog and Gomez ( 2020 ) identified upland rice farmers’ productivity and technical efficiency in Sarangani. Aguilar et al. ( 2021 ) examined the drivers of change, resilience, and potential trajectories of traditional rice-based agroecosystems in Kiangan, Ifugao. Pasiona et al. ( 2021 ) found that using the “modified listening group method” enables farmers’ peer-to-peer learning of technical concepts. Sociologist Shunnan Chiang ( 2020 ) examined the driving forces behind the transformation of the status of brown rice in the country.
Social scientists could further look into the social, cultural, technological, and human–ecological interactions in the temporal and spatial studies of different rice farming regions in Indonesia and the Philippines. Other topics could include the cultural practices and the techno-social relationships of rice farmers (e.g., Shepherd and McWilliam, 2011 ) and other players in the rice value chain, local and indigenous knowledge and practices on agrobiodiversity conservation, historical and invasive pests and diseases, agricultural health and safety, farmer education, and aging agricultural infrastructures. Lastly, future researchers can explore the impacts of adopting rice farming technologies in the different stages or processes of the rice value chain. They can look into its short- and longer-term effects on farmers’ livelihoods and conduct comparative analyses on how it improves, or not, their livelihoods, and whether farmers regard them better compared to the traditional and indigenous practices and beliefs that their communities apply and observe in rice farming.
Social and environmental psychology
Our review yielded no article published on the social and environmental psychology aspects of rice farming in Indonesia and the Philippines, suggesting a new research frontier. The increasing demand for and competition over agricultural and natural resources due to climate change and population expansion (Foley et al., 2011 ) opens up new and emerging sociopsychological dilemmas for society to understand, answer, and, hopefully, solve. Social and environmental psychologists can help shed light on these questions, such as those related to understanding farmers’ pro-environmental agricultural practices (Price and Leviston, 2014 ), sustainable sharing and management of agricultural and natural resources (Anderies et al., 2013 ; Biel and Gärling, 1995 ), and understanding the psychosocial consequences of resource scarcity (Griskevicius et al., 2013 ). Broadly, social psychology examines human feelings, thoughts, and behaviors and how they are influenced by the actual, imagined, and implied presence, such as the effects of internalized social norms (Allport, 1985 ). Social psychologists look at the many facets of personality and social interactions and explore the impacts of interpersonal and group relationships on human behavior (American Psychological Association, 2014b ). On the other hand, environmental psychology examines psychological processes in human encounters with their natural and built environments (Stern, 2000 ). Environmental psychologists are interested in studying and understanding people’s responses to natural and technological hazards, conservation, and perceptions of the environment (American Psychological Association, 2014a ).
Using the Asian Journal of Social Psychology and the Journal of Environmental Psychology as benchmarks, we recommend that scholars explore the following uncharted or least studied areas of rice research in Indonesia and the Philippines: sociopsychological processes such as attitude and behavior, social cognition, self and identity, individual differences, emotions, human–environmental health and well-being, social influence, communication, interpersonal behavior, intergroup relations, group processes, and cultural processes. Researchers could also investigate the psycho-behavioral areas of nature–people interactions, theories of place, place attachment, and place identity, especially in rice farming. Other topics may include farmers’ perceptions, behaviors, and management of environmental risks and hazards; theories of pro-environmental behaviors; psychology of sustainable agriculture; and the psychological aspects of resource/land management and land-use change.
Climate change, weather extremes, and disaster risk reduction
Indonesia’s and Philippines’ equatorial and archipelagic location in the Pacific Ring of Fire (Bankoff, 2016 ; Parwanto and Oyama, 2014 ), coupled with their political, social, and economic complexities (Bankoff, 2003 , 2007 ; UNDRR and CRED, 2020 ), expose and render these countries highly vulnerable to hazards, such as typhoons, strong winds, tsunamis, storm surges, floods, droughts, and earthquakes. The accelerating global climate change increases the frequency and intensity of some of these hazards, such as prolonged droughts, torrential rainfalls causing floods, and super typhoons (IPCC, 2014 ). For example, torrential flooding, induced by heavy rains caused by low pressures and southwest monsoons, has been damaging lives and livelihoods, including rice production (Statista, 2021 ). The 2020 droughts caused over 12 trillion pesos (~US$239.40 billion) of economic losses in the Philippines (Statista, 2021 ) and affected millions of Indonesians (UNDRR, 2020 ). Prolonged drought in Indonesia has also exacerbated fire hazards, which caused transboundary haze pollution in neighboring countries, like Singapore and the Philippines, inflecting environmental health damages (Aiken, 2004 ; Sheldon and Sankaran, 2017 ; Tan-Soo and Pattanayak, 2019 ). Increasing sea-level rise due to anthropogenic climate change puts cities like Jakarta and Manila at risk of sinking in the next 30–50 years (Kulp and Strauss, 2019 ). The high vulnerability, frequent exposure, and low capacities of marginalized and poor Indonesians and Filipinos turn these hazards into disasters (Gaillard, 2010 ; Kelman, 2020 ; Kelman et al., 2015 ), negatively affecting rice agriculture.
Given these contexts, climate change, weather extremes, and disaster risks, vis-à-vis its impacts on the rice sector, are issues of profound interest to scholars and the Indonesian and Philippine governments. In the Philippines, climate adaptation studies include re-engineering rice drying systems for climate change (Orge et al., 2020 ) and evaluating climate-smart farming practices and the effectiveness of Climate-Resiliency Field Schools in Mindanao (Chandra et al., 2017 ). In Indonesia, where some rice farming communities are vulnerable to sea-level rise, scholars are experimenting to identify rice cultivars with high yields under different salinity levels (Sembiring et al., 2020 ). Hohl et al. ( 2021 ) used a regional climate model to develop index-based drought insurance products to help the Central Java government make drought-related insurance payments to rice farmers. Aprizal et al. ( 2021 ) utilized land-use conditions and rain variability data to develop a flood inundation area model for the Way Sekampung sub-watershed in Lampung, Sumatra. Others also looked at the science behind liquefaction hazards caused by irrigation systems for wet rice cultivation in mountainous farming communities like the 2018 earthquake-triggered landslides in Palu Valley, Sulawesi (Bradley et al., 2019 ).
Examples of climate mitigation-related studies in the Philippines include investigating the social innovation strategies in engaging rice farmers in bioenergy development (Minas et al., 2020 ) and evaluating the environmental performance and energy efficiency of rice straw-generated electricity sources (Reaño et al., 2021 ). Doliente and Samsatli ( 2021 ) argue that it is possible to combine energy and food production to increase farm productivity and reduce GHG emissions with minimal land expansion. Other studies have looked into the potential of alternate wetting and drying irrigation practices to mitigate emissions from rice fields (Sander et al., 2020 ).
Future work could explore the following topic areas: demand-driven research and capacity building on climate information and environmental monitoring; nature-based solutions for climate mitigation and adaptation; water–energy–food nexus in rice farming; the nexus of climate change and conflict in rice farming communities; the potentials and pitfalls of social capital in farmer’s everyday adaptation; just energy transitions in rice farming; vulnerabilities from and traditional/local/indigenous ways of adapting to climate change, including the various learning strategies communities use for its preservation; and examples, potentials, and barriers in adopting climate-smart agriculture technologies and practices.
Demographic transitions and aging farmers
Farmers are in various stages and speeds of aging globally (Rigg et al., 2020 ). Evidence of aging farmers in the Global North has been reported in Australia (O’Callaghan and Warburton, 2017 ; Rogers et al., 2013 ), the Czech Republic (Zagata et al., 2015 ), England (Hamilton et al., 2015 ), Japan (Poungchompu et al., 2012 ; Usman et al., 2021 ), and the United States of America (Mitchell et al., 2008 ; Reed, 2008 ; Yudelman and Kealy, 2000 ). Similarly, in the Global South, HelpAge International ( 2014 , p. 21) reported that “there has been a universal trend of an increase in the proportion of older people… attached to agricultural holdings… across [Low and Middle-income Countries in] Asia, sub-Saharan Africa, Latin America, and the Caribbean.” Moreover, farming populations are aging rapidly in East and Southeast Asia (Rigg et al., 2020 ) and southern Africa (HelpAge, 2014 ). Despite this, the literature on aging farmers in Southeast Asian countries remains scant, except for case studies conducted in some villages and provinces in Thailand (Poungchompu et al., 2012 ; Rigg et al., 2018 , 2020 ) and the Philippines (Moya et al., 2015 ; Palis, 2020 ).
Rice farmers’ quiet but critical demographic transformation in Indonesia and the Philippines has not received much attention from scientists, policymakers, and development practitioners. The impacts of aging farmers on the micro-, meso-, and macro-level agricultural processes and outcomes are important issues that require urgent attention. Studies done in other countries could guide future work to explore these questions in Indonesia and the Philippines. These include aging’s potential negative implications in terms of agricultural efficiency and productivity (e.g., Tram and McPherson ( 2016 ) in Vietnam, and Szabo et al. ( 2021 ) in Thailand), food security (e.g., Bhandari and Mishra ( 2018 ) in Asia), farming continuity and sustainability (e.g., O’Callaghan and Warburton ( 2017 ) in Australia, Palis ( 2020 ) in the Philippines, and Rigg et al. ( 2018 , 2020 ) in Thailand), aging and feminization of farm labor (e.g., Liu et al. ( 2019 ) in China), cleaner production behaviors (e.g., Liu et al. ( 2021 ) in Northern China), youth barriers to farm entry (e.g., Zagata and Sutherland ( 2015 ) in Europe), and health and well-being of aging farmers (Jacka, 2018 ; Rogers et al., 2013 ; Ye et al., 2017 ).
Other critical new topics include the (dis)engagement and re-engagement of young people in rice farming; gender dynamics—including structures and systems of inclusion and/or exclusion—in rice production; the impacts of migration and return migration to farming households; community-based and policy-oriented case studies that provide examples of successfully engaging and retaining youth workers in farming; and social protection measures for aging farmers, to name a few.
Contemporary and emerging challenges
One of the biggest and most visible contemporary global challenges is the Covid-19 pandemic. Most pronounced is the pandemic’s impacts on the healthcare system and the economic toll it caused on the lives and livelihoods of people, including rice farmers. Only 0.18% (4 articles) of our dataset have investigated the impacts of Covid-19 on rice systems in Indonesia and the Philippines. Ling et al. ( 2021 ) assessed the effects of the pandemic on the domestic rice supply vis-à-vis food security among ASEAN member-states. They found that Singapore and Malaysia were highly vulnerable to a pandemic-induced rice crisis, while Brunei, Indonesia, and the Philippines are moderately vulnerable. They argued that Southeast Asian rice importers should consider alternative import strategies to reduce their high-risk reliance on rice supply from Thailand and Vietnam and look for other suppliers in other continents.
Rice prices did not change in the early months of the pandemic in Indonesia (Nasir et al., 2021 ); however, as the health emergency progressed, distributors and wholesalers incurred additional costs due to pandemic-induced mobility restrictions (Erlina and Elbaar, 2021 ). In the Philippines, San Juan ( 2021 ) argues that the global rice supply disruption due to the pandemic proves that the country cannot heavily rely on rice imports; instead, it should work on strengthening its domestic rice supply. To realize this, he recommended drastic investments in agriculture and research, rural solar electrification, and the promotion of research on increasing rice yields, boosting productivity, and planting sustainably as feasible steps on the road to rice self-sufficiency.
The ways and extent to which the pandemic negatively affected or exacerbated the vulnerabilities of rice farmers and other value chain actors remain an understudied area in the social studies of rice. Scholars could study the pandemic’s impacts in conjunction with other contemporary and emerging challenges like climate change, weather extremes, aging, conflict, and poverty. Scholars could also explore the medium- and longer-term impacts of the pandemic on rice production, unemployment risks, rice supply and nutrition security of farming households, and the potential and extent to which economic stimulus can benefit rice farmers, to name a few. Most importantly, the pandemic allows researchers and governments to assess the business-as-usual approach that resulted in the disastrous impacts of the pandemic on different sectors, including rice farmers, and hopefully devise strategies to learn from these experiences.
From our review of 2243 articles, cumulatively written by 6893 authors using almost 80,000 references, we conclude that a voluminous amount of rice research has been conducted in Indonesia and the Philippines since 2001. As in other reviews, (e.g., on energy research by Sovacool, 2014 ), our results show that women scholars remain underrepresented in rice research in Indonesia and the Philippines. While interdisciplinary collaboration is abundant, most of these studies belong to the natural sciences with minimal contributions from the social sciences, arts, and humanities. University and research institutions contributed the most to rice research in Indonesia and the Philippines: from hybrid rice cultivars, water management, and technology adoption to socio-cultural, political, economic, and policy issues. Influential scholars in the field were affiliated with the IRRI, which can be expected given the institute’s focus on rice, and key agriculture-focused universities and government bureaus such as the University of the Philippines and the PhilRice in the Philippines, and the Institut Pertanian Bogor University and the Universitas Gadja Maja in Indonesia. We also discussed some examples of economic, political, and policy studies; social, anthropological, and cultural research; social and environmental psychology; climate change, weather extremes, and disaster risk reduction; demographic transitions; and contemporary and emerging issues and studies on rice in the two Southeast Asian countries. Ultimately, we hope that this systematic review can help illuminate key topic areas of rice research in Indonesia and the Philippines and magnify the crucial contributions from and possible research areas and questions that interdisciplinary and comparative social scientists can further explore.
Data availability
The dataset analyzed in this study is available in the Figshare online repository via https://doi.org/10.6084/m9.figshare.17284814.v2 . All codes about Bibliometrix are available at https://bibliometrix.org/ .
Addor N, Melsen LA (2019) Legacy, rather than adequacy, drives the selection of hydrological models. Water Resour Res 55(1):378–390. https://doi.org/10.1029/2018WR022958
Article ADS Google Scholar
Afa LO, Purwoko BS, Junaedi A, Haridjaja O, Dewi IS (2018) Screening of hybrid rice tolerance through stimulated condition of drought stress in rainfed lowland. Biosci Res 15(3):1630–1637
Google Scholar
Aguilar CHM, Altoveros NC, Borromeo TH, Dayo MHF, Koohafkan P (2021) Traditional rice-based agroecosystem in Kiangan, Ifugao, Philippines: drivers of change, resilience, and potential trajectories. Agroecol Sustain Food Syst 45(2):296–316. https://doi.org/10.1080/21683565.2020.1813861
Article Google Scholar
Aiken SR (2004) Runaway fires, smoke‐haze pollution, and unnatural disasters in Indonesia. Geogr Rev 94(1):55–79. https://doi.org/10.1111/j.1931-0846.2004.tb00158.x
Alejo LA, Ella VB (2019) Assessing the impacts of climate change on dependable flow and potential irrigable area using the swat model. The case of maasin river watershed in Laguna, Philippines. J Agric Eng 50(2):88–98. https://doi.org/10.4081/jae.2018.941
Allport GW (1985) The historical background of social psychology. In: Lindzey G, Aronson E (eds.) The handbook of social psychology. McGraw Hill
American Psychological Association (2014a) A career in climate and environmental psychology. https://www.apa.org/education-career/guide/subfields/environment/education-training
American Psychological Association (2014b) Social psychology examines interpersonal relationships. https://www.apa.org/education-career/guide/subfields/social
Anderies JM, Janssen MA, Lee A, Wasserman H (2013) Environmental variability and collective action: experimental insights from an irrigation game. Ecol Econ 93:166–176. https://doi.org/10.1016/j.ecolecon.2013.04.010
Ansari A, Lin Y-P, Lur H-S (2021) Evaluating and adapting climate change impacts on rice production in Indonesia: a case study of the Keduang subwatershed, Central Java. Environments-MDPI 8(11) https://doi.org/10.3390/environments8110117
Antle JM, Pingali PL (1994) Pesticides, productivity, and farmer health: a Philippine case study. Am J Agric Econ 76(3):418–430. https://doi.org/10.2307/1243654
Aprizal, Alisjahbana SW, Nurhasanah A (2021) The development of the flood inundation area model in the way Sekampung subwatershed in Lampung. Rev Int Geogr Educ 11(3):1246–1256. https://doi.org/10.33403/rigeo.800567
Aria M, Alterisio A, Scandurra A, Pinelli C, D’Aniello B (2021) The scholar’s best friend: research trends in dog cognitive and behavioral studies. Animal Cogn 24(3):541–553. https://doi.org/10.1007/s10071-020-01448-2
Aria M, Cuccurullo C (2017) bibliometrix: an R-tool for comprehensive science mapping analysis. J Informetr 11(4):959–975. https://doi.org/10.1016/j.joi.2017.08.007
Arif C, Setiawan BI, Sofiyuddin HA, Martief LM, Mizoguchi M, Doi R (2012) Estimating crop coefficient in intermittent irrigation paddy fields using excel solver. Rice Sci 19(2):143–152. https://doi.org/10.1016/S1672-6308(12)60033-X
Arif C, Toriyama K, Nugroho BDA, Mizoguchi M (2015) Crop coefficient and water productivity in conventional and system of rice intensification (SRI) irrigation regimes of terrace rice fields in Indonesia. J Teknol 76(15):97–102. https://doi.org/10.11113/jt.v76.5958
Asian Development Bank (ed.) (2002) Indigenous peoples/ethnic minorities and poverty reduction. Environment and Social Safeguard Division, Regional and Sustainable Development Department, Asian Development Bank
Awan TH, Chauhan BS, Sta Cruz PC (2014) Influence of environmental factors on the germination of Urena lobata L. and its response to herbicides. PLoS ONE 9(3) https://doi.org/10.1371/journal.pone.0090305
Awan TH, Cruz PCS, Chauhan BS (2015) Efficacy and economics of different herbicides, their weed species selectivity, and the productivity of mechanized dry-seeded rice. Crop Prot 78:239–246. https://doi.org/10.1016/j.cropro.2015.09.016
Article CAS Google Scholar
Bairagi S, Custodio MC, Durand-Morat A, Demont M (2021) Preserving cultural heritage through the valorization of Cordillera Heirloom rice in the Philippines. Agric Hum Values 38(1):257–270. https://doi.org/10.1007/s10460-020-10159-w
Bairagi S, Demont M, Custodio MC, Ynion J (2020) What drives consumer demand for rice fragrance? Evidence from South and Southeast Asia. Br Food J 122(11):3473–3498. https://doi.org/10.1108/BFJ-01-2019-0025
Bairagi S, Gustafson CR, Custodio MC, Ynion J, Demont M (2021) What drives consumer demand for packaged rice? Evidence from South and Southeast Asia. Food Control 129 https://doi.org/10.1016/j.foodcont.2021.108261
Bajwa AA, Chauhan BS (2017) Rice production in Australia. In: Chauhan BS, Jabran K, Mahajan G (eds.) Rice production worldwide. Springer International Publishing, pp. 169–184
Balié J, Valera HG (2020) Domestic and international impacts of the rice trade policy reform in the Philippines. Food Policy 92 https://doi.org/10.1016/j.foodpol.2020.101876
Balogbog KJM, Gomez NU (2020) Determinants of productivity and technical efficiency of upland rice farming system in Sarangani Province, Phillipines. Mindanao J Sci Technol 18(2):125–137
Banayo NPMC, Bueno CS, Haefele SM, Desamero NV, Kato Y (2018) Site-specific nutrient management enhances sink size, a major yield constraint in rainfed lowland rice. Field Crops Res 224:76–79. https://doi.org/10.1016/j.fcr.2018.05.006
Banayo NPMC, Haefele SM, Desamero NV, Kato Y (2018) On-farm assessment of site-specific nutrient management for rainfed lowland rice in the Philippines. Field Crops Res 220:88–96. https://doi.org/10.1016/j.fcr.2017.09.011
Bankoff G (2003) Cultures of disaster: society and natural hazards in the Philippines. RoutledgeCurzon
Bankoff G (2007) Dangers to going it alone: social capital and the origins of community resilience in the Philippines. Contin Change 22(2):327–355. https://doi.org/10.1017/S0268416007006315
Bankoff G (2016) Hazardousness of place a new comparative approach to the Filipino past. Philipp Stud: Hist Ethnogr Viewp 64(3/4):335–357
Belder P, Bouman BAM, Cabangon R, Guoan L, Quilang EJP, Yuanhua L, Spiertz JHJ, Tuong TP(2004) Effect of water-saving irrigation on rice yield and water use in typical lowland conditions in Asia. Agric Water Manag 65(3):193–210. https://doi.org/10.1016/j.agwat.2003.09.002
Beltran JC, Pannell DJ, Doole GJ (2012) Economic implications of herbicide resistance and high labour costs for management of annual barnyardgrass ( Echinochloa crus-galli ) in Philippine rice farming systems. Crop Prot 31(1):31–39. https://doi.org/10.1016/j.cropro.2011.09.012
Beltran JC, White B, Burton M, Doole GJ, Pannell DJ (2013) Determinants of herbicide use in rice production in the Philippines. Agric Econ (UK) 44(1):45–55. https://doi.org/10.1111/j.1574-0862.2012.00631.x
Bhandari H, Mishra AK (2018) Impact of demographic transformation on future rice farming in Asia. Outlook Agric 47(2):125–132. https://doi.org/10.1177/0030727018769676
Biel A, Gärling T (1995) The role of uncertainty in resource dilemmas. J Environ Psychol 15(3):221–233. https://doi.org/10.1016/0272-4944(95)90005-5
Biodiversitas (2021) Aims and scope|biodiversitas. J Biol Divers https://smujo.id/biodiv/aimsandscope
Bouman BAM, Humphreys E, Tuong TP, Barker R (2007) Rice and water. In: Advances in agronomy, vol 92. Elsevier, pp. 187–237
Bouman BAM, Peng S, Castañeda AR, Visperas RM (2005) Yield and water use of irrigated tropical aerobic rice systems. Agric Water Manag 74(2):87–105. https://doi.org/10.1016/j.agwat.2004.11.007
Bouman BAM, Tuong TP (2001) Field water management to save water and increase its productivity in irrigated lowland rice. Agric Water Manag 49(1):11–30. https://doi.org/10.1016/S0378-3774(00)00128-1
Bradley K, Mallick R, Andikagumi H, Hubbard J, Meilianda E, Switzer A, Du N, Brocard G, Alfian D, Benazir B, Feng G, Yun S-H, Majewski J, Wei S, Hill EM (2019) Earthquake-triggered 2018 Palu Valley landslides enabled by wet rice cultivation. Nat Geosci 12(11):935–939. https://doi.org/10.1038/s41561-019-0444-1
Article ADS CAS Google Scholar
Broad R, Cavanagh J (2012) The development and agriculture paradigms transformed: reflections from the small-scale organic rice fields of the Philippines. J Peasant Stud 39(5):1181–1193. https://doi.org/10.1080/03066150.2012.722082
Cabasan MTN, Tabora JAG, Cabatac NN, Jumao-as CM, Soberano JO, Turba JV, Dagamac NHA, Barlaan E (2019) Economic and ecological perspectives of farmers on rice insect pest management. Global J Environ Sci Manag 5(1):31–42. https://doi.org/10.22034/gjesm.2019.01.03
Carpenter D (2003) An investigation into the transition from technological to ecological rice farming among resource poor farmers from the Philippine island of Bohol. Agric Hum Values 20(2):165–176. https://doi.org/10.1023/A:1024013509602
Chandra A, Dargusch P, McNamara KE, Caspe AM, Dalabajan D (2017) A Study of Climate-Smart Farming Practices and Climate-resiliency Field Schools in Mindanao, the Philippines. World Dev 98:214–230. https://doi.org/10.1016/j.worlddev.2017.04.028
Chauhan BS (2011) Crowfootgrass ( Dactyloctenium aegyptium ) germination and response to herbicides in the Philippines. Weed Sci 59(4):512–516. https://doi.org/10.1614/WS-D-11-00048.1
Chauhan BS, Abugho SB (2012) Threelobe morningglory ( Ipomoea triloba ) germination and response to herbicides. Weed Sci 60(2):199–204. https://doi.org/10.1614/WS-D-11-00137.1
Chauhan BS, Johnson DE (2009) Seed germination ecology of junglerice ( Echinochloa colona ): a major weed of rice. Weed Sci 57(3):235–240. https://doi.org/10.1614/WS-08-141.1
Chiang S-N (2020) Transecting the fall and rise of brown rice—the historic encounters of the global food system, nutrition science, and malnutrition in the Philippines. Food Cult Soc 23(2):229–248. https://doi.org/10.1080/15528014.2019.1682889
Chrisendo D, Krishna VV, Siregar H, Qaim M (2020) Land-use change, nutrition, and gender roles in Indonesian farm households. Forest Policy Econ 118 https://doi.org/10.1016/j.forpol.2020.102245
Coe K, Scacco J (2017) Content analysis, quantitative. In: Matthes J, Davis CS, Potter RF (eds.) The International Encyclopedia of Communication Research methods, 1st edn. Wiley
Connor M, de Guia AH, Pustika AB, Sudarmaji, Kobarsih M, Hellin J (2021) Rice farming in central java, Indonesia—adoption of sustainable farming practices, impacts and implications. Agronomy 11(5) https://doi.org/10.3390/agronomy11050881
Cuaton GP, Caluza LJB, Neo JFV (2021) A topic modeling analysis on the early phase of COVID-19 response in the Philippines. Int J Disaster Risk Reduct 61:102367. https://doi.org/10.1016/j.ijdrr.2021.102367
Article PubMed PubMed Central Google Scholar
Cuccurullo C, Aria M, Sarto F (2016) Foundations and trends in performance management. A twenty-five years bibliometric analysis in business and public administration domains. Scientometrics 108(2):595–611. https://doi.org/10.1007/s11192-016-1948-8
De Datta SK (1981) Principle and practices of rice production. John Wiley and Sons.
Della Corte V, Del Gaudio G, Sepe F, Sciarelli F (2019) Sustainable tourism in the open innovation realm: a bibliometric analysis. Sustainability 11(21):6114. https://doi.org/10.3390/su11216114
DeWalt BR (1985) Anthropology, sociology, and farming systems research. Hum Organ 44(2):106–114
Dipti SS, Bergman C, Indrasari SD, Herath T, Hall R, Lee H, Habibi F, Bassinello PZ, Graterol E, Ferraz JP, Fitzgerald M (2012) The potential of rice to offer solutions for malnutrition and chronic diseases. Rice 5(1):16. https://doi.org/10.1186/1939-8433-5-16
Disyacitta Nariswari RA, Lauder MRMT (2021) Agricultural terms in rice production: a dialectological study [TÉrminos agrÍcolas en la producciÓn de arroz: Un estudio dialectal]. Dialectologia 26:145–178. https://doi.org/10.1344/DIALECTOLOGIA2021.26.7
Doliente SS, Samsatli S (2021) Integrated production of food, energy, fuels and chemicals from rice crops: multi-objective optimisation for efficient and sustainable value chains. J Clean Prod 285 https://doi.org/10.1016/j.jclepro.2020.124900
Dominik C, Seppelt R, Horgan FG, Settele J, Václavík T (2018) Landscape composition, configuration, and trophic interactions shape arthropod communities in rice agroecosystems. J Appl Ecol 55(5):2461–2472. https://doi.org/10.1111/1365-2664.13226
Dominko M, Verbič M (2019) The economics of subjective well-being: a bibliometric analysis. J Happiness Stud 20(6):1973–1994. https://doi.org/10.1007/s10902-018-0022-z
Donthu N, Kumar S, Mukherjee D, Pandey N, Lim WM (2021) How to conduct a bibliometric analysis: an overview and guidelines. J Bus Res 133:285–296. https://doi.org/10.1016/j.jbusres.2021.04.070
Doorman F (1990) A social science contribution to applied agricultural research for the small farm sector: the diagnostic case study as a tool for problem identification. Agricult Syst 32(3):273–290. https://doi.org/10.1016/0308-521X(90)90005-B
Doorman F (1991) Adept at adapting. Contributions of sociology to agricultural research for small farmers in developing countries: the case of rice in the Dominican Republic. Wagening Stud Sociol https://www.researchgate.net/publication/40176828_Adept_at_adapting_Contributions_of_sociology_to_agricultural_research_for_small_farmers_in_developing_countries_the_case_of_rice_in_the_Dominican_Republic
Ducusin RJC, Espaldon MVO, Rebancos CM, De Guzman LEP (2019) Vulnerability assessment of climate change impacts on a Globally Important Agricultural Heritage System (GIAHS) in the Philippines: the case of Batad Rice Terraces, Banaue, Ifugao, Philippines. Clim Change https://doi.org/10.1007/s10584-019-02397-7
Dulbari, Santosa E, Koesmaryono Y, Sulistyono E, Wahyudi A, Agusta H, Guntoro D (2021) Local adaptation to extreme weather and it’s implication on sustainable rice production in Lampung, Indonesia. Agrivita 43(1):125–136. https://doi.org/10.17503/agrivita.v43i1.2338
Ebitani T, Hayashi N, Omoteno M, Ozaki H, Yano M, Morikawa M, Fukuta Y (2011) Characterization of Pi13, a blast resistance gene that maps to chromosome 6 in Indica rice ( Oryza sativa L. variety, Kasalath). Breed Sci 61(3):251–259. https://doi.org/10.1270/jsbbs.61.251
Elsevier (2021) About Scopus—Abstract and citation database. Elsevier
Engelbert EA (1953) Agriculture and the political process. In: Increasing understanding of public problems and policies. AgEconSearch Res Agric Appl Econ https://doi.org/10.22004/AG.ECON.17209
Erlina Y, Elbaar EF (2021) Impact of COVID-19 pandemic on local rice supply chain flow patterns in Kapuas regency, Central Kalimantan, Indonesia. WSEAS Trans Bus Econ 18:941–948. https://doi.org/10.37394/23207.2021.18.89
Fabro L, Varca LM (2012) Pesticide usage by farmers in Pagsanjan-Lumban catchment of Laguna de Bay, Philippines. Agric Water Manag 106:27–34. https://doi.org/10.1016/j.agwat.2011.08.011
Falagas ME, Pitsouni EI, Malietzis GA, Pappas G (2008) Comparison of PubMed, Scopus, Web of Science, and Google Scholar: strengths and weaknesses. FASEB J 22(2):338–342. https://doi.org/10.1096/fj.07-9492LSF
Article CAS PubMed Google Scholar
Fang AH (2016) Linkage between rural voters and politicians: effects on rice policies in the Philippines and Thailand: voter–politician linkage & rice policies. Asia Pacif Policy Stud 3(3):505–517. https://doi.org/10.1002/app5.150
Ferrero A, Nguyen NV (2004) The sustainable development of rice-based production systems in Europe. https://www.fao.org/3/y5682e/y5682e0g.htm
Foley JA, Ramankutty N, Brauman KA, Cassidy ES, Gerber JS, Johnston M, Mueller ND, O’Connell C, Ray DK, West PC, Balzer C, Bennett EM, Carpenter SR, Hill J, Monfreda C, Polasky S, Rockström J, Sheehan J, Siebert S, Zaks DPM (2011) Solutions for a cultivated planet. Nature 478(7369):337–342. https://doi.org/10.1038/nature10452
Article ADS CAS PubMed Google Scholar
Gaillard JC (2010) Vulnerability, capacity and resilience: perspectives for climate and development policy. J Int Dev 22(2):218–232. https://doi.org/10.1002/jid.1675
Article MathSciNet Google Scholar
Gata L, Losloso J, Nilo P (2020) Gender and the use of climate information in agricultural decision-making amidst climate change: the case of rice and corn production in oriental Mindoro, Philippines. Philipp Agric Sci 103(Special issue):93–104
Gergon EB, Miller SA, Davide RG, Opina OS, Obien SR (2001) Evaluation of cultural practices (surface burning, deep ploughing, organic amendments) for management of rice root-knot nematode in rice–onion cropping system and their effect on onion ( Allium cepa L.) yield. Int J Pest Manag 47(4):265–272. https://doi.org/10.1080/09670870110047118
Gergon EB, Miller SA, Halbrendt JM, Davide RG (2002) Effect of rice root-knot nematode on growth and yield of Yellow Granex onion. Plant Dis 86(12):1339–1344. https://doi.org/10.1094/PDIS.2002.86.12.1339
Gil JDB, Reidsma P, Giller K, Todman L, Whitmore A, van Ittersum M (2019) Sustainable development goal 2: Improved targets and indicators for agriculture and food security. Ambio 48(7):685–698. https://doi.org/10.1007/s13280-018-1101-4
Glaeser B (ed.) (2010) The green revolution revisited, 1st edn. Routledge.
Glänzel W, Schubert A (2005) Analysing scientific networks through co-authorship. In Moed HF, Glänzel W, Schmoch U (eds.) Handbook of quantitative science and technology research. Kluwer Academic Publishers, pp. 257–276
Gott RC, Coyle DR (2019) Educated and engaged communicators are critical to successful integrated pest management adoption. J Integr Pest Manag 10(1):35. https://doi.org/10.1093/jipm/pmz033
Grant WP (2012) Can political science contribute to agricultural policy. Policy Soc 31(4):271–279. https://doi.org/10.1016/j.polsoc.2012.09.001
Griskevicius V, Ackerman JM, Cantú SM, Delton AW, Robertson TE, Simpson JA, Thompson ME, Tybur JM (2013) When the economy falters, do people spend or save? Responses to resource scarcity depend on childhood environments. Psychol Sci 24(2):197–205. https://doi.org/10.1177/0956797612451471
Article PubMed Google Scholar
Hadiprayitno II (2017) The limit of narratives: ethnicity and indigenous rights in Papua, Indonesia. Int J Minor Group Rights 24(1):1–23. https://doi.org/10.1163/15718115-02401004
Halwart M, Litsinger JA, Viray MC, Kaule G (2014) Efficacy of common carp and Nile tilapia as biocontrol agents of the golden apple snail in the Philippines. Philipp J Sci 143(2):125–136
Hamilton W, Bosworth G, Ruto E (2015) Entrepreneurial younger farmers and the “young farmer problem” in England. Agric For 61(4):61–69
Hanif KI, Herlinda S, Irsan C, Pujiastuti Y, Prabawati G, Hasbi, Karenina T (2020) The impact of bioinsecticide overdoses of Beauveria bassiana on species diversity and abundance of not targeted arthropods in South Sumatra (Indonesia) freshwater swamp paddy. Biodiversitas 21(5):2124–2136. https://doi.org/10.13057/biodiv/d210541
Hantrais L (1995) Comparative research methods. Soc Res Update 13(Summer):2–11
Heckelman A, Smukler S, Wittman H (2018) Cultivating climate resilience: a participatory assessment of organic and conventional rice systems in the Philippines. Renew Agric Food Syst 33(3):225–237. https://doi.org/10.1017/S1742170517000709
HelpAge (2014) The ageing of rural populations: evidence on older farmers in low and middle-income countries. HelpAge International, p. 24
Herlinda S, Prabawati G, Pujiastuti Y, Susilawati, Karenina T, Hasbi, Irsan C (2020) Herbivore insects and predatory arthropods in freshwater swamp rice field in South Sumatra, Indonesia sprayed with bioinsecticides of entomopathogenic fungi and abamectin. Biodiversitas 21(8):3755–3768. https://doi.org/10.13057/biodiv/d210843
Hirsch JE (2005) An index to quantify an individual’s scientific research output. Proc Natl Acad Sci USA 102(46):16569–16572
Hohl R, Jiang Z, Tue Vu M, Vijayaraghavan S, Liong S-Y (2021) Using a regional climate model to develop index-based drought insurance for sovereign disaster risk transfer. Agric Finance Rev 81(1):151–168. https://doi.org/10.1108/AFR-02-2020-0020
Horgan FG, Bernal CC, Vu Q, Almazan MLP, Ramal AF, Yasui H, Fujita D (2018) Virulence adaptation in a rice leafhopper: exposure to ineffective genes compromises pyramided resistance. Crop Prot 113:40–47. https://doi.org/10.1016/j.cropro.2018.07.010
Horgan FG, Martínez EC, Stuart AM, Bernal CC, Martín EC, Almazan MLP, Ramal AF (2019) Effects of vegetation strips, fertilizer levels and varietal resistance on the integrated management of arthropod biodiversity in a tropical rice ecosystem. Insects 10(10) https://doi.org/10.3390/insects10100328
Horgan FG, Romena AM, Bernal CC, Almazan MLP, Ramal AF (2021) Differences between the strength of preference-performance coupling in two rice stemborers (Lepidoptera: Pyralidae, Crambidae) promotes coexistence at field-plot scales. Environ Entomol 50(4):929–939. https://doi.org/10.1093/ee/nvab034
Article CAS PubMed PubMed Central Google Scholar
Intal PS, Garcia MC (2005) Rice and Philippine politics. Working Paper No. 2005–13. PIDS Discussion Paper Series. https://www.econstor.eu/handle/10419/127899
IPCC (2014) Climate change 2014: mitigation of climate change. Contribution of Working Group III to the Fifth Assessment Report of the Intergovernmental Panel on Climate Change. Cambridge University Press
IRRI (1982) The role of anthropologists and other social scientists in interdisciplinary teams developing improved food production technology. International Rice Research Institute, p. 103
IRRI (2013) Rice almanac: source book for the most important economic activities on Earth, 4th edn. IRRI
Iyer AS, McCouch SR (2004) The rice bacterial blight resistance gene xa5 encodes a novel form of disease resistance. Mol Plant–Microbe Interact® 17(12):1348–1354. https://doi.org/10.1094/MPMI.2004.17.12.1348
Jacka T (2018) Translocal family reproduction and agrarian change in China: a new analytical framework. J Peasant Stud 45(7):1341–1359. https://doi.org/10.1080/03066150.2017.1314267
Jenkins KEH, Sovacool BK, Mouter N, Hacking N, Burns M-K, McCauley D (2021) The methodologies, geographies, and technologies of energy justice: a systematic and comprehensive review. Environ Res Lett 16(4):043009. https://doi.org/10.1088/1748-9326/abd78c
John A, Fielding M (2014) Rice production constraints and ‘new’ challenges for South Asian smallholders: Insights into de facto research priorities. Agric Food Secur 3(1):18. https://doi.org/10.1186/2048-7010-3-18
Juliano LM, Casimero MC, Llewellyn R (2010) Multiple herbicide resistance in barnyardgrass ( Echinochloa crus-galli ) in direct-seeded rice in the Philippines. Int J Pest Manag 56(4):299–307. https://doi.org/10.1080/09670874.2010.495795
Kadeawi S, Swaruno, Nasution A, Hairmansis A, Telebanco-Yanoria MJ, Obara M, Hayashi N, Fukuta Y (2021) Pathogenicity of isolates of the rice blast pathogen ( Pyricularia oryzae ) from Indonesia. Plant Disease 105(3):675–683. https://doi.org/10.1094/PDIS-05-20-0949-RE
Kauffman HE, Reddy APK, Hsieh SPY, Merca SD (1973) An improved technique for evaluating resistance of rice varieties to Xanthomonas oryzae . Plant Disease Report 57:537–541
Kelman I (2020) Disaster by choice: how our actions turn natural hazards into catastrophes (new product). Oxford University Press.
Kelman I, Gaillard JC, Mercer J (2015) Climate change’s role in disaster risk reduction’s future: beyond vulnerability and resilience. Int J Disaster Risk Sci 6(1):21–27. https://doi.org/10.1007/s13753-015-0038-5
Khatun MM, Siddik MS, Rahman MA, Khaled S (2021) Content analysis of Covid-19 and agriculture news in Bangladesh using topic modeling algorithm. Curr Appl Sci Technol 21(02):299–317
Khumairoh U, Lantinga EA, Schulte RPO, Suprayogo D, Groot JCJ (2018) Complex rice systems to improve rice yield and yield stability in the face of variable weather conditions. Sci Rep 8(1) https://doi.org/10.1038/s41598-018-32915-z
Kim K-H, Raymundo AD, Aikins CM (2019) Development of a rice tungro epidemiological model for seasonal disease risk management in the Philippines. Eur J Agron 109 https://doi.org/10.1016/j.eja.2019.04.006
Komatsuzaki M, Syuaib MF (2010) Comparison of the farming system and carbon sequestration between conventional and organic rice production in West Java, Indonesia. Sustainability 2(3):833–843. https://doi.org/10.3390/su2030833
Kraehmer H, Thomas C, Vidotto F (2017) Rice production in Europe. In Chauhan BS, Jabran K, Mahajan G (eds.) Rice production worldwide. Springer International Publishing, pp. 93–116
Kulp SA, Strauss BH (2019) New elevation data triple estimates of global vulnerability to sea-level rise and coastal flooding. Nat Commun 10(1):4844. https://doi.org/10.1038/s41467-019-12808-z
Article ADS CAS PubMed PubMed Central Google Scholar
Kumar S, Tripathi S, Singh SP, Prasad A, Akter F, Syed MA, Badri J, Das SP, Bhattarai R, Natividad MA, Quintana M, Venkateshwarlu C, Raman A, Yadav S, Singh SK, Swain P, Anandan A, Yadaw RB, Mandal NP, Henry A (2021) Rice breeding for yield under drought has selected for longer flag leaves and lower stomatal density. J Exp Bot 72(13):4981–4992. https://doi.org/10.1093/jxb/erab160
Laborte AG, Paguirigan NC, Moya PF, Nelson A, Sparks AH, Gregorio GB (2015) Farmers’ preference for rice traits: insights from farm surveys in Central Luzon, Philippines, 1966–2012. PLoS ONE 10(8):e0136562. https://doi.org/10.1371/journal.pone.0136562
Laiprakobsup T (2014) Populism and agricultural trade in developing countries: a case study of Thailand’s rice-pledging scheme. Int Rev Public Adm 19(4):380–394. https://doi.org/10.1080/12294659.2014.967000
Lakitan B, Alberto A, Lindiana L, Kartika K, Herlinda S, Kurnianingsih A (2018) The benefits of biochar on rice growth and yield in tropical riparian wetland, South Sumatra, Indonesia. Chiang Mai Univ J Nat Sci 17(2):111–126. https://doi.org/10.12982/CMUJNS.2018.0009
Lampayan RM, Samoy-Pascual KC, Sibayan EB, Ella VB, Jayag OP, Cabangon RJ, Bouman BAM (2015) Effects of alternate wetting and drying (AWD) threshold level and plant seedling age on crop performance, water input, and water productivity of transplanted rice in Central Luzon, Philippines. Paddy Water Environ 13(3):215–227. https://doi.org/10.1007/s10333-014-0423-5
Lapuz RR, Javier S, Aquino JDC, Undan JR (2019) Gene expression and sequence analysis of BADH1 gene in CLSU aromatic rice ( Oryza sativa L.) accessions subjected to drought and saline condition. J Nutr Sci Vitaminol 65:S196–S199. https://doi.org/10.3177/jnsv.65.S196
Ling TJ, Shamsudin MN, Bing WZ, Thi Cam Nhung P, Rabbany MG (2021) Mitigating the impacts of COVID-19 on domestic rice supply and food security in Southeast Asia. Outlook Agric 50(3):328–337. https://doi.org/10.1177/00307270211024275
Litsinger JA, Canapi BL, Bandong JP (2011) Cultural practices mitigate irrigated rice insect pest losses in the Philippines. Philipp J Sci 140(2):179–194
Liu J, Du S, Fu Z (2021) The impact of rural population aging on farmers’ cleaner production behavior: evidence from five provinces of the North China Plain. Sustainability 13(21):12199. https://doi.org/10.3390/su132112199
Liu J, Xu Z, Zheng Q, Hua L (2019) Is the feminization of labor harmful to agricultural production? The decision-making and production control perspective. J Integr Agric 18(6):1392–1401. https://doi.org/10.1016/S2095-3119(19)62649-3
Lopez MV, Mendoza TC (2004) Management options for salt-affected rice-based farming systems during the El Niño and La Niña phenomena. J Sustain Agric 23(4):19–37. https://doi.org/10.1300/J064v23n04_04
Mackill DJ, Bonman JM (1992) Inheritance of rice resistance in near-isogenic lines of rice. Phytopathology 82:746–749
Macrae G (2011) Rice farming in Bali organic production and marketing challenges. Crit Asian Stud 43(1):69–92. https://doi.org/10.1080/14672715.2011.537852
MacRae GS, Arthawiguna IWA (2011) Sustainable agricultural development in Bali: is the Subak an obstacle, an agent or subject. Hum Ecol 39(1):11–20. https://doi.org/10.1007/s10745-011-9386-y
Mahajan G, Kumar V, Chauhan BS (2017) Rice production in India. In: Chauhan BS, Jabran K, Mahajan G (eds.) Rice production worldwide. Springer International Publishing, pp. 53–91
Manalo IJA, van de Fliert E, Fielding E (2020) Rice farmers adapting to drought in the Philippines. Int J Agric Sustain 18(6):594–605. https://doi.org/10.1080/14735903.2020.1807301
Martawijaya S, Montgomery RD (2004) Bureaucrats as entrepreneurs: a case study of organic rice production in East Java. Bull Indones Econ Stud 40(2):243–252. https://doi.org/10.1080/0007491042000205303
Mendoza TC (2004) Evaluating the benefits of organic farming in rice agroecosystems in the Philippines. J Sustain Agric 24(2):93–115. https://doi.org/10.1300/J064v24n02
Mew TW (1987) Current Status and future prospects of research on bacterial blight of rice. Annu Rev Phytopathol 25(1):359–382. https://doi.org/10.1146/annurev.py.25.090187.002043
Minas AM, Mander S, McLachlan C (2020) How can we engage farmers in bioenergy development? Building a social innovation strategy for rice straw bioenergy in the Philippines and Vietnam. Energy Res Soc Sci 70. https://doi.org/10.1016/j.erss.2020.101717
Misdawita, Hartono D, Nugroho A (2019) Impacts of food prices on the economy: social accounting matrix and microsimulation approach in Indonesia. Rev Urban Reg Dev Stud 31(1–2):137–154. https://doi.org/10.1111/rurd.12099
Mitchell J, Bradley D, Wilson J, Goins RT (2008) The aging farm population and rural aging research. J Agromed 13(2):95–109. https://doi.org/10.1080/10599240802125383
Mizobuchi R, Sato H, Fukuoka S, Yamamato S, Kawasaki-Tanaka A, Fukuta Y (2014) Mapping of a QTL for field resistance to blast ( Pyricularia oryzae Cavara) in Ingngoppor-tinawon, a rice ( Oryza sativa L.) landrace from the Philippines. Japan Agric Res Q 48(4):425–431. https://doi.org/10.6090/jarq.48.425
Mizuno K, Mugniesyah SS, Herianto AS, Tsujii H (2013) Talun-Huma, Swidden agriculture, and rural economy in West Java, Indonesia. Southeast Asian Stud 2(2):351–381
Moya P, Kajisa K, Barker R, Mohanty S, Gascon F, San Valentin MR (2015) Changes in rice farming in the Philippines: insights from five decades of a household-level survey. International Rice Research Institute.
Mucharam I, Rustiadi E, Fauzi A, Harianto (2020) Assessment of rice farming sustainability: evidence from Indonesia provincial data. Int J Sustain Dev Plan 15(8):1323–13332. https://doi.org/10.18280/ijsdp.150819
Muhardi, Effendy (2021) Technical efficiency and the factors that affect it in rice production in Indonesia. Asian J Agric Rural Dev 11(3):230–235. https://doi.org/10.18488/journal.ajard.2021.113.230.235
Mulyani A, Darwanto DH, Widodo S, Masyhuri (2020) Production efficiency of inpago unsoed-1 and situbagendit rice farming in central Java, Indonesia. Biodiversitas 21(7):3276–3286. https://doi.org/10.13057/biodiv/d210751
Munajati SL, Kartodihardjo H, Saleh MB, Nurwadjedi (2021) Ecosystem services dynamics in Bogor Regency. Indones J Geogr 53(2):264–273. https://doi.org/10.22146/IJG.64493
Muthayya S, Sugimoto JD, Montgomery S, Maberly GF (2014) An overview of global rice production, supply, trade, and consumption: global rice production, consumption, and trade. Ann N Y Acad Sci 1324(1):7–14. https://doi.org/10.1111/nyas.12540
Article ADS PubMed Google Scholar
Nasir MA, Jamhar, Mulyo JH, Dumasari D (2021) Spatial study on How COVID-19 affects the Indonesian rice markets integration: period of March to July 2020. Rev Int Geogr Educ 11(4):672–683. https://doi.org/10.33403/rigeo.8006781
Neyra-Cabatac NM, Pulhin JM, Cabanilla DB (2012) Indigenous agroforestry in a changing context: the case of the Erumanen ne Menuvu in Southern Philippines. Forest Policy Econ 22:18–27. https://doi.org/10.1016/j.forpol.2012.01.007
Niones JM, Lipio PLG, Cruz AS, Cabral MCJ, Hautea DM, Lucob-Agustin N, Suralta RR (2021) Genome-wide association mapping for the identification of SNPs controlling lateral root plasticity in selected rice germplasms of the Philippines. Philipp J Sci 150(3):663–674
Noviar H, Masbar R, Aliasuddin, Syahnur S, Zulham T, Saputra J (2020) The agricultural commercialisation and its impact on economy management: an application of duality-neoclassic and stochastic frontier approach. Ind Eng Manag Syst 19(3):510–519. https://doi.org/10.7232/iems.2020.19.3.510
Nugroho BDA, Toriyama K, Kobayashi K, Arif C, Yokoyama S, Mizoguchi M (2018) Effect of intermittent irrigation following the system of rice intensification (SRI) on rice yield in a farmer’s paddy fields in Indonesia. Paddy Water Environ 16(4), 715–723. https://doi.org/10.1007/s10333-018-0663-x
O’Callaghan Z, Warburton J (2017) No one to fill my shoes: narrative practices of three ageing Australian male farmers. Ageing Soc 37(3):441–461. https://doi.org/10.1017/S0144686X1500118X
Official Gazette of the Philippines (2021) Executive order no. 1061, s. 1985|GOVPH. Official Gazette of the Republic of the Philippines. https://mirror.officialgazette.gov.ph/1985/11/05/executive-order-no-1061-s-1985/
Oladele OI, Boago C (2011) Content analysis of agricultural news in Botswana newspapers. J Hum Ecol 36(3):173–177. https://doi.org/10.1080/09709274.2011.11906432
Ou SK (1985) Rice diseases, 2nd edn. Commonwealth Agricultural Bureau.
Orge RF, Sawey DA, Leal LV, Gagelonia EC (2020) Re-engineering the paddy rice drying system in the Philippines for climate change adaptation Dry Technol 38(11):1462–1473. https://doi.org/10.1080/07373937.2019.1648289
Page MJ, McKenzie JE, Bossuyt PM, Boutron I, Hoffmann TC, Mulrow CD, Shamseer L, Tetzlaff JM, Akl EA, Brennan SE, Chou R, Glanville J, Grimshaw JM, Hróbjartsson A, Lalu MM, Li T, Loder EW, Mayo-Wilson E, McDonald S, Moher D (2021) The PRISMA 2020 statement: an updated guideline for reporting systematic reviews. BMJ 372:n71. https://doi.org/10.1136/bmj.n71
Palis FG (2020) Aging filipino rice farmers and their aspirations for their children. Philipp J Sci 149(2):321–331
Palis FG, Lampayan RM, Flor RJ, Sibayan E (2017) A multi-stakeholder partnership for the dissemination of alternate wetting and drying water-saving technology for rice farmers in the Philippines. AIMS Agric Food 2(3):290–309. https://doi.org/10.3934/agrfood.2017.3.290
Panuju DR, Mizuno K, Trisasongko BH (2013) The dynamics of rice production in Indonesia 1961–2009. J Saudi Soc Agric Sci 12(1):27–37. https://doi.org/10.1016/j.jssas.2012.05.002
Papademetriou M, Dent F, Herath E (eds.) (2000) Bridging the rice yield gap in the Asia-Pacific Region. UNFAO
Parwanto NB, Oyama T (2014) A statistical analysis and comparison of historical earthquake and tsunami disasters in Japan and Indonesia. Int J Disaster Risk Reduct 7:122–141. https://doi.org/10.1016/j.ijdrr.2013.10.003
Pasiona SP, Nidoy MGM, Manalo IV JA (2021) Modified listening group method as a knowledge-sharing and learning mechanism in agricultural communities in the Philippines. J Agric Educ Ext 27(1):89–106. https://doi.org/10.1080/1389224X.2020.1816477
Peng S, Cassman KG, Virmani SS, Sheehy J, Khush GS (1999) Yield potential trends of tropical rice since the release of IR8 and the challenge of increasing rice yield potential. Crop Sci 39(6):1552–1559. https://doi.org/10.2135/cropsci1999.3961552x
Peng S, Tang Q, Zou Y (2009) Current status and challenges of rice production in China. Plant Prod Sci 12(1):3–8. https://doi.org/10.1626/pps.12.3
Perez LM, Redoña ED, Mendioro MS, Vera Cruz CM, Leung H (2008) Introgression of Xa4, Xa7 and Xa21 for resistance to bacterial blight in thermosensitive genetic male sterile rice ( Oryza sativa L.) for the development of two-line hybrids. Euphytica 164(3):627–636. https://doi.org/10.1007/s10681-008-9653-1
Pielke R, Linnér B-O (2019) From Green Revolution to Green Evolution: a critique of the political myth of averted famine. Minerva 57(3):265–291. https://doi.org/10.1007/s11024-019-09372-7
Pingali PL (2012) Green Revolution: impacts, limits, and the path ahead. Proc Natl Acad Sci USA 109(31):12302–12308. https://doi.org/10.1073/pnas.0912953109
Pingali P, Roger P (eds.) (1995) Impact of pesticides on farmer health and the rice environment. Springer
Poungchompu S, Tsuneo K, Poungchompu P (2012) Aspects of the aging farming population and food security in agriculture for Thailand and Japan. Int J Environ Rural Dev 03(01):1–6
Price JC, Leviston Z (2014) Predicting pro-environmental agricultural practices: the social, psychological and contextual influences on land management. J Rural Stud 34:65–78. https://doi.org/10.1016/j.jrurstud.2013.10.001
Prom-u-thai C, Rerkasem B (2020) Rice quality improvement. A review. Agron Sustain Dev 40(4):28. https://doi.org/10.1007/s13593-020-00633-4
Propper CR, Hardy LJ, Howard BD, Flor RJB, Singleton GR (2020) Role of farmer knowledge in agro-ecosystem science: Rice farming and amphibians in the Philippines. Hum–Wildl Interact 14(2) https://www.scopus.com/inward/record.uri?eid=2-s2.0-85091838772&partnerID=40&md5=4ad2c5129ee2e5bfbe34d6f1505327bc
Putra AS, Tong G, Pribadi DO (2020) Food security challenges in rapidly urbanizing developing countries: insight from Indonesia. Sustainability (Switzerland) 12(22):1–18. https://doi.org/10.3390/su12229550
Reaño RL, de Padua VAN, Halog AB (2021) Energy efficiency and life cycle assessment with system dynamics of electricity production from rice straw using a combined gasification and internal combustion engine. Energies 14(16) https://doi.org/10.3390/en14164942
Reed DB (2008) America’s aging farmers: tenacious, productive, and underresearched. J Agromed 13(2):69–70. https://doi.org/10.1080/10599240802293942
Resosudarmo BP (2012) Implementing a national environmental policy: Understanding the ‘success’ of the 1989–1999 integrated pest management programme in Indonesia: the 1989–1999 integrated pest management programme. Singap J Trop Geogr 33(3):365–380. https://doi.org/10.1111/sjtg.12006
Rigg J, Phongsiri M, Promphakping B, Salamanca A, Sripun M (2020) Who will tend the farm? Interrogating the ageing Asian farmer. J Peasant Stud 47(2):306–325. https://doi.org/10.1080/03066150.2019.1572605
Rigg J, Salamanca A, Phongsiri M, Sripun M (2018) More farmers, less farming? Understanding the truncated agrarian transition in Thailand. World Dev 107:327–337. https://doi.org/10.1016/j.worlddev.2018.03.008
Rochman KL, Misno, Mubarok Z, Bunyamin, Bahrudin (2021) Ngahuma (Planting rice in the fields) and tilled land limitation of the baduy tribe in Indonesia. Geoj Tour Geosites 34(1):63–68. https://doi.org/10.30892/gtg.34109-620
Rogers M, Barr N, O’Callaghan Z, Brumby S, Warburton J (2013) Healthy ageing: farming into the twilight. Rural Soc 22(3):251–262. https://doi.org/10.5172/rsj.2013.22.3.251
Romanillos RD, Dizon JT, Quimbo MAT, Cruz PCS, Miranda RB (2016) Community development strategies and other factors affecting rice productivity in inland valleys in Quezon province, Luzon, Philippines. Asia Life Sci 25(2):603–620
Rustiadi E, Pravitasari AE, Setiawan Y, Mulya SP, Pribadi DO, Tsutsumida N (2021) Impact of continuous Jakarta megacity urban expansion on the formation of the Jakarta-Bandung conurbation over the rice farm regions. Cities 111 https://doi.org/10.1016/j.cities.2020.103000
Ruzol C, Lomente LL, Pulhin J (2020) Mapping access and use of weather and climate information to aid farm decisions in the Philippines. Philipp Agric Sci 103(Special issue):25–39
Ruzol C, Lomente LL, Pulhin J (2021) Cultural consensus knowledge of rice farmers for climate risk management in the Philippines. Clim Risk Manag 32 https://doi.org/10.1016/j.crm.2021.100298
Salman D, Kasim K, Ahmad A, Sirimorok N (2021) Combination of bonding, bridging and linking social capital in a livelihood system: nomadic duck herders amid the covid-19 pandemic in South Sulawesi, Indonesia. Forest Soc 5(1):136–158. https://doi.org/10.24259/fs.v5i1.11813
Salsinha YCF, Indradewa D, Purwestri YA, Rachmawati D (2020) Selection of drought-tolerant local rice cultivars from east Nusa Tenggara, Indonesia during vegetative stage Biodiversitas 21(1):170–178. https://doi.org/10.13057/biodiv/d210122
Salsinha YCF, Maryani, Indradewa D, Purwestri YA, Rachmawati D (2021) Morphological and anatomical characteristics of Indonesian rice roots from East Nusa Tenggara contribute to drought tolerance. Asian J Agric Biol 2021(1):1–11. https://doi.org/10.35495/ajab.2020.05.304
Sambrook J, Fritsch EF, Maniatis T (1989) Molecular cloning: a laboratory manual, 2nd edn. Cold Spring Harbor laboratory press.
San Juan DM (2021) A review of rice tariffication in the time of COVID-19: rationale and road to rice self-sufficiency in the Philippines. Asia-Pacif Soc Sci Rev 21(4):50–71
Sander BO, Schneider P, Romasanta R, Samoy-Pascual K, Sibayan EB, Asis CA, Wassmann R (2020) Potential of alternate wetting and drying irrigation practices for the mitigation of GHG emissions from rice fields: two cases in central Luzon (Philippines) Agriculture (Switzerland) 10(8):1–19. https://doi.org/10.3390/agriculture10080350
Sanjatmiko P (2021) Multispecies ethnography: reciprocal interaction between residents and the environment in Segara Anakan, Indonesia. South East Asia Res 29(3):384–400. https://doi.org/10.1080/0967828X.2021.1978313
Santiago JO, Buot Jr IE (2018) Conceptualizing the socio-ecological resilience of the Chaya rice terraces, a socio-ecological production landscape in Mayoyao, Ifugao, Luzon Island, Philippines. J Mar Island Cult 7(1):107–126. https://doi.org/10.21463/jmic.2018.07.1.07
Satriawan E, Shrestha R (2018) Mistargeting and regressive take up of the Indonesian Rice Subsidy Program. Asian Econ J 32(4):387–415. https://doi.org/10.1111/asej.12164
Schaarschmidt S, Lawas LMF, Glaubitz U, Li X, Erban A, Kopka J, Krishna Jagadish SV, Hincha DK, Zuther E (2020) Season affects yield and metabolic profiles of rice ( Oryza sativa ) under high night temperature stress in the field. Int J Mol Sci 21(9) https://doi.org/10.3390/ijms21093187
Sembiring H, Subekti NA, Erythrina, Nugraha D, Priatmojo B, Stuart AM (2020) Yield gap management under seawater intrusion areas of Indonesia to improve rice productivity and resilience to climate change. Agriculture (Switzerland) 10(1). https://doi.org/10.3390/agriculture10010001
Sen S, Chakraborty R, Kalita P (2020) Rice—not just a staple food: a comprehensive review on its phytochemicals and therapeutic potential. Trends Food Sci Technol 97:265–285. https://doi.org/10.1016/j.tifs.2020.01.022
Setiawan BI, Irmansyah A, Arif C, Watanabe T, Mizoguchi M, Kato H (2013) Effects of groundwater level on CH4 and N2O emissions under SRI paddy management in Indonesia. Taiwan Water Conserv 61(4):135–146
Shabandeh M (2021) Total global rice consumption 2021. Statista https://www.statista.com/statistics/255977/total-global-rice-consumption/
Sheldon TL, Sankaran C (2017) The impact of Indonesian forest fires on Singaporean pollution and health. Am Econ Rev 107(5):526–529. https://doi.org/10.1257/aer.p20171134
Shepherd C, McWilliam A (2011) Ethnography, agency, and materiality: anthropological perspectives on rice development in east Timor. East Asian Sci Technol Soc 5(2):189–215. https://doi.org/10.1215/18752160-1262876
Singh V, Zhou S, Ganie Z, Valverde B, Avila L, Marchesan E, Merotto A, Zorrilla G, Burgos N, Norsworthy J, Bagavathiannan M (2017) Rice production in the Americas. In Chauhan BS, Jabran K, Mahajan G (eds.) Rice production worldwide. Springer International Publishing, pp. 137–168
Singleton G (2011) Outwit rats with smart, green solutions. Appropr Technol 38(1):66–67
Singleton GR, Belmain S, Brown PR, Aplin K, Htwe NM (2010) Impacts of rodent outbreaks on food security in Asia. Wildl Res 37(5):355–359. https://doi.org/10.1071/WR10084
Singleton GR, Sudarmaji, Jacob J, Krebs CJ (2005) Integrated management to reduce rodent damage to lowland rice crops in Indonesia. Agric Ecosyst Environ 107(1):75–82. https://doi.org/10.1016/j.agee.2004.09.010
Sovacool BK (2014) What are we doing here? Analyzing fifteen years of energy scholarship and proposing a social science research agenda. Energy Res Soc Sci 1:1–29. https://doi.org/10.1016/j.erss.2014.02.003
Statista (2021) Topic: natural disasters in the Philippines. Statista https://www.statista.com/topics/5845/natural-disasters-in-the-philippines-at-a-glance/
Stern PC (2000) Psychology and the science of human-environment interactions. Am Psychol 55(5):523–530. https://doi.org/10.1037/0003-066X.55.5.523
Stuart AM, Prescott CV, Singleton GR, Joshi RC (2011) Knowledge, attitudes and practices of farmers on rodent pests and their management in the lowlands of the Sierra Madre Biodiversity Corridor, Philippines. Crop Prot 30(2):147–154. https://doi.org/10.1016/j.cropro.2010.10.002
Stuecker MF, Tigchelaar M, Kantar MB (2018) Climate variability impacts on rice production in the Philippines. PLoS ONE 13(8). https://doi.org/10.1371/journal.pone.0201426
Sumarminingsih E (2021) Modeling rainfall using spatial vector autoregressive. Int J Agric Stat Sci 17(1):21–30
Syahrawati M, Martono E, Putra NS, Purwanto BH (2018) Effects of fertilizer, irrigation level and spider presence on abundance of herbivore and carnivore in rice cultivation in Yogyakarta. Asian J Agric Biol6(3):385–395
Szabo S, Apipoonanon C, Pramanik M, Leeson K, Singh DR (2021) Perceptions of an ageing agricultural workforce and farmers’ productivity strategies: evidence from Prachinburi Province, Thailand. Outlook Agric 50(3):294–304. https://doi.org/10.1177/00307270211025053
Tan-Soo J-S, Pattanayak SK (2019) Seeking natural capital projects: forest fires, haze, and early-life exposure in Indonesia. Proc Natl Acad Sci USA 116(12):5239–5245. https://doi.org/10.1073/pnas.1802876116
Tekken V, Spangenberg JH, Burkhard B, Escalada M, Stoll-Kleemann S, Truong DT, Settele J (2017) “Things are different now”: farmer perceptions of cultural ecosystem services of traditional rice landscapes in Vietnam and the Philippines. Ecosyst Serv 25:153–166. https://doi.org/10.1016/j.ecoser.2017.04.010
Templeton DJ, Jamora N (2010) Economic assessment of a change in Pesticide Regulatory Policy in the Philippines. World Dev 38(10):1519–1526. https://doi.org/10.1016/j.worlddev.2010.06.009
Tram LTQ, McPherson M (2016) The ageing and feminization of the agricultural labor force in the lower Mekong Basin [Policy Brief]. Lower Mekong Public Policy Initiative
Trijatmiko KR, Supriyanta, Prasetiyono J, Thomson MJ, Vera Cruz CM, Moeljopawiro S, Pereira A (2014) Meta-analysis of quantitative trait loci for grain yield and component traits under reproductive-stage drought stress in an upland rice population. Mol Breed 34(2):283–295. https://doi.org/10.1007/s11032-013-0012-0
Triwidodo H (2020) Brown planthoppers infestations and insecticides use pattern in Java, Indonesia. Agrivita 42(2):320–330. https://doi.org/10.17503/agrivita.v0i0.2501
Tuong TP, Bouman BAM (2003) Rice production in water-scarce environments. In Kijne JW, Barker R, Molden DJ (eds.) Water productivity in agriculture: limits and opportunities for improvement. CABI Publisher
UNDP Philippines (2010) Indigenous peoples in the Philippines [Fast Facts]. United Nations Development Programme, pp. 1–2
UNDRR (2020) Disaster risk reduction in the Republic of Indonesia: status Report 2020 [Status Report 2020]. United Nations Office for Disaster Risk Reduction (UNDRR), Regional Office for Asia and the Pacific
UNDRR & CRED (2020) The Human Cost of Disasters—an overview of the last 20 years 2000–2019—world. ReliefWeb https://reliefweb.int/report/world/human-cost-disasters-overview-last-20-years-2000-2019
Usman M, Sawaya A, Igarashi M, Gayman JJ, Dixit R (2021) Strained agricultural farming under the stress of youths’ career selection tendencies: a case study from Hokkaido (Japan). Humanit Soc Sci Commun 8(1):19. https://doi.org/10.1057/s41599-020-00688-4
Vu Q, Ramal AF, Villegas JM, Jamoralin A, Bernal CC, Pasang JM, Almazan MLP, Ramp D, Settele J, Horgan FG (2018) Enhancing the parasitism of insect herbivores through diversification of habitat in Philippine rice fields. Paddy Water Environ 16(2):379–390. https://doi.org/10.1007/s10333-018-0662-y
Waite MB (1915) The importance of research as a means of increasing agricultural production. Ann Am Acad Political Soc Sci 59:40–50
Wakabayashi H, Hongo C, Igarashi T, Asaoka Y, Tjahjono B, Permata I (2021) Flooded rice paddy detection using sentinel-1 and planetscope data: a case study of the 2018 spring flood in West Java, Indonesia. IEEE J Sel Top Appl Earth Observ Remote Sens 14:6291–6301. https://doi.org/10.1109/JSTARS.2021.3083610
Waltman L (2016) A review of the literature on citation impact indicators. J Informetr 10(2):365–391. https://doi.org/10.1016/j.joi.2016.02.007
White B, Wijaya H (2021) What kind of labour regime is contract farming? Contracting and sharecropping in Java compared. J Agrar Change https://doi.org/10.1111/joac.12459
Widyantari IN, Jamhari, Waluyati LR, Mulyo JH (2018) Does the tribe affect technical efficiency? Case study of local farmer rice farming in Merauke regency, Papua, Indonesia. Int J Mech Eng Technol 9(11):37–47
Widyantari IN, Jamhari, Waluyati LR, Mulyo JH (2019) Case study of farming from transmigrants and local farmers in the district of Semangga and Tanah Miring, Merauke Regency, Papua. Int J Civil Eng Technol 10(2):761–772
Xu W, Virmani SS, Hernandez JE, Sebastian LS, Redoña ED, Li Z (2002) Genetic diversity in the parental lines and heterosis of the tropical rice hybrids. Euphytica 127(1):139–148. https://doi.org/10.1023/A:1019960625003
Ye J, He C, Liu J, Wang W, Chen S (2017) Left-behind elderly: Shouldering a disproportionate share of production and reproduction in supporting China’s industrial development. J Peasant Stud 44(5):971–999. https://doi.org/10.1080/03066150.2016.1186651
Yoshida T, Anas, Rosniawaty S, Setiamihardja R (2009) Genetic background of Indonesia rice germplasm and its relationship to agronomic characteristics and eating quality. Jpn J Crop Sci 78(3):335–343. https://doi.org/10.1626/jcs.78.335
Yudelman M, Kealy LJM (2000) The graying of farmers. Popul Today 28(4):1–6
Zagata L, Hádková Š, Mikovcová M (2015) Basic outline of the problem of the “ageing population of farmers” in the Czech Republic. Agris On-Line Pap Econ Inform 7(1):89–96. https://doi.org/10.7160/aol.2015.070110
Zagata L, Sutherland L-A (2015) Deconstructing the ‘young farmer problem in Europe’: towards a research agenda. J Rural Stud 38:39–51. https://doi.org/10.1016/j.jrurstud.2015.01.003
Zapico FL, DIzon JT, Borromeo TH, McNally KL, Fernando ES, Hernandez JE (2020) Genetic erosion in traditional rice agro-ecosystems in Southern Philippines: drivers and consequences. Plant Genet Resour: Charact Util 18(1):1–10. https://doi.org/10.1017/S1479262119000406
Zenna N, Senthilkumar K, Sie M (2017) Rice production in Africa. In: Chauhan BS, Jabran K, Mahajan G (eds.) Rice production worldwide. Springer International Publishing, pp. 117–135
Zupic I, Čater T (2015) Bibliometric methods in management and organization. Organ Res Methods 18(3):429–472. https://doi.org/10.1177/1094428114562629
Download references
Acknowledgements
The work described in this paper was substantially supported by a grant from the Research Grants Council of the Hong Kong Special Administrative Region, China (Project No. HKUST 26600521). Partial funding was also made available by the HKUST Institute for Emerging Market Studies with support from EY.
Author information
Authors and affiliations.
The Hong Kong University of Science and Technology, Hong Kong SAR, China
Ginbert P. Cuaton & Laurence L. Delina
You can also search for this author in PubMed Google Scholar
Contributions
GPC made substantial contributions through this paper’s original conceptualization, data curation and processing, formal analysis, methodology, writing the original draft, and reviewing, revising, and finalizing the paper. LLD made substantial contributions to its conceptualization, funding acquisition, supervision, and reviewing and editing of the review paper.
Corresponding author
Correspondence to Ginbert P. Cuaton .
Ethics declarations
Competing interests.
The authors declare no competing interests.
Ethics approval
This article does not contain any studies with human participants performed by any of the authors.
Informed consent
Additional information.
Publisher’s note Springer Nature remains neutral with regard to jurisdictional claims in published maps and institutional affiliations.
Supplementary information
Supplementary info, rights and permissions.
Open Access This article is licensed under a Creative Commons Attribution 4.0 International License, which permits use, sharing, adaptation, distribution and reproduction in any medium or format, as long as you give appropriate credit to the original author(s) and the source, provide a link to the Creative Commons license, and indicate if changes were made. The images or other third party material in this article are included in the article’s Creative Commons license, unless indicated otherwise in a credit line to the material. If material is not included in the article’s Creative Commons license and your intended use is not permitted by statutory regulation or exceeds the permitted use, you will need to obtain permission directly from the copyright holder. To view a copy of this license, visit http://creativecommons.org/licenses/by/4.0/ .
Reprints and permissions
About this article
Cite this article.
Cuaton, G.P., Delina, L.L. Two decades of rice research in Indonesia and the Philippines: A systematic review and research agenda for the social sciences. Humanit Soc Sci Commun 9 , 372 (2022). https://doi.org/10.1057/s41599-022-01394-z
Download citation
Received : 20 February 2022
Accepted : 30 September 2022
Published : 14 October 2022
DOI : https://doi.org/10.1057/s41599-022-01394-z
Share this article
Anyone you share the following link with will be able to read this content:
Sorry, a shareable link is not currently available for this article.
Provided by the Springer Nature SharedIt content-sharing initiative
Quick links
- Explore articles by subject
- Guide to authors
- Editorial policies


An official website of the United States government
The .gov means it’s official. Federal government websites often end in .gov or .mil. Before sharing sensitive information, make sure you’re on a federal government site.
The site is secure. The https:// ensures that you are connecting to the official website and that any information you provide is encrypted and transmitted securely.
- Publications
- Account settings
Preview improvements coming to the PMC website in October 2024. Learn More or Try it out now .
- Advanced Search
- Journal List
- BMC Plant Biol

A genomic perspective on the important genetic mechanisms of upland adaptation of rice
1 CAS-Max Planck Junior Research Group, State Key Laboratory of Genetic Resources and Evolution, Kunming Institute of Zoology, Chinese Academy of Sciences, Kunming 650223, China
2 Food Crops Research Institute, Yunnan Academy of Agricultural Sciences, Kunming 650205, China
3 BGI-Shenzhen, Shenzhen 518083, China
Shilai Zhang
Zhiheng gou.
4 Inner Mongolia Agricultural University, Hohhot 010018, China
5 Center for Epigenetics, Johns Hopkins University School of Medicine Baltimore, MD, Baltimore 21205, USA
Wangqi Huang
Associated data.
Cultivated rice consists of two important ecotypes, upland and irrigated, that have respectively adapted to either dry land or irrigated cultivation. Upland rice, widely adopted in rainfed upland areas in virtue of its little water requirement, contains abundant untapped genetic resources, such as genes for drought adaptation. With water shortage exacerbated and population expanding, the need for breeding crop varieties with drought adaptation becomes more and more urgent. However, a previous oversight in upland rice research reveals little information regarding its genetic mechanisms for upland adaption, greatly hindering progress in harnessing its genetic resources for breeding and cultivation.
In this study, we selected 84 upland and 82 irrigated accessions from all over the world, phenotyped them under both irrigated and dry land environments, and investigated the phylogenetic relations and population structure of the upland ecotype using whole genome variation data. Further comparative analysis yields a list of differentiated genes that may account for the phenotypic and physiological differences between upland and irrigated rice.
Conclusions
This study represents the first genomic investigation in a large sample of upland rice, providing valuable gene list for understanding upland rice adaptation, especially drought-related adaptation, and its subsequent utilization in modern agriculture.
Asian cultivated rice ( Oryza sativa type indica and Oryza sativa . type japonica) has phenotypically divergent ecotypes [ 1 ]. Irrigated and upland rice, respectively adapted to growing in paddy fields and rain-fed dry lands, are two main ecotypes that are generally considered to show apparent phenotypic differentiation. The upland type is more drought tolerant, taller, of lower tillering potential, more resistant to blast, and tends to have longer and thicker roots than its irrigated counterpart [ 2 , 3 ]. Accordingly, upland varieties are widely adopted in upland areas to optimize crop yields because of their more efficient water usage and adaptation to dry land. Though the yield of upland rice is comparatively lower than the irrigated rice, it provides the daily staple food on which nearly one hundred million people depends [ 4 ]. Because we are now facing global population expansion and a growing shortage of water resources, upland rice and especially knowledge about its genetic mechanisms for dry land adaptation become more important as they allow the possibility of transforming or modifying high-yield irrigated varieties of rice to upland ones.
Drought resistance is an important component of rice adaption to most rainfed upland areas because in this ecosystem water could not be well preserved and frequent or sporadic drought events could occur each year [ 5 ]. Previous research proposed three different mechanisms for plant drought resistance: drought escape, dehydration avoidance, and dehydration tolerance [ 6 ]. Through drought escape, timing of plant lifecycle is modulated to escape the time of drought when drought time is predictable in each year. Dehydration avoidance refers to the case that plants constitutively avoid excess loss of water through more developed root systems or a thick layer of cutin [ 6 ]. Dehydration tolerance refers to the inducible mechanisms, like osmotic adjustment, which help plants to prevent damage and survive when excess loss of water does happen [ 6 ]. For the frequent and unpredictable drought stress in upland areas, the dehydration avoidance and dehydration tolerance should be the appropriate options for upland crops. In fact, upland rice seems to depend more on the constitutive dehydration avoidance than the inducible dehydration tolerance in its adaptation [ 7 - 9 ]. By far, some genes have been reported to be related with drought resistance[ 10 ], such as genes in abscisic acid (ABA) synthetic pathway (such as 9- cis -epoxycarotenoid dioxygenase genes) [ 11 , 12 ], transcription factors interacting with the cis-acting dehydration response element (DRE) [ 13 ], genes synthesizing osmoprotectants [ 14 , 15 ], and mitogen-activated protein kinase (MAPK) genes [ 16 ]. However, these studies focused on single gene’s effect, while adaptation to dry land may involve many genes in the genome. Furthermore, the valuable genetic resources within upland rice, which were ignored by most previous studies, are also worthy of enhanced utilization.
Upland and irrigated rice evolve their respective characteristic traits under both natural and artificial selections, which lead to phenotypic adaptation to respective environment and simultaneously genetic differentiation between ecotypes. Some statistical tools for identifying selected regions during population differentiation have been well established. Fixation index ( F ST ) is a commonly used statistics that measure population differentiation based on genetic polymorphism data. F ST is positively associated with allele frequency difference. Cross-population composite likelihood ratio test (XP-CLR), a newly invented approach, incorporates not only genetic differentiation but also allele frequency spectrum information [ 17 ]. By jointly modeling multilocus allele frequency differentiation and frequency spectrum under neutrality and selection, XP-CLR was demonstrated to have high power in detecting selective sweeps during population differentiation. Besides, Nielsen’s CLR, a parametric test also based on composite likelihood ratio, detects selective sweeps using the spatial distribution of site frequency spectrum [ 18 ]. This approach only uses SNP data of one population and thus detects selective sweeps in single population without consideration of population differentiation.
To investigate the genetic mechanisms for upland adaptation and identify genes for its important component, ‘drought resistance’, we use our whole-genome re-sequencing data for a large panel of accessions, including 84 upland accessions and 82 irrigated accessions reported by our previous work [ 19 ] , where these data were used to test that a low-diversity region containing a key enzyme gene of abscisic acid synthesis was due to human selection. Considering the wide distribution of upland rice in the world, we obtained our samples from Asia, Africa and South America, covering most major distribution areas of upland rice. We explored phylogenetic relationship between upland and irrigated ecotypes and population structure of current upland rice population. More importantly, by detecting selective sweep regions using F ST and XP-CLR, we compiled a list of candidate genes that are likely responsible for upland adaptation or that are related to phenotypic differentiation between the upland and irrigated ecotypes, providing valuable insights about crop breeding for dry land.
Phenotypes of the upland accessions differ from those of the irrigated ones
For the 84 globally sampled and representative upland rice accessions (see Additional files 1 and 2 ), we want to confirm whether they possess the reported phenotypes that distinguish them from irrigated rice [ 2 , 3 ]. Therefore we grew the varieties to do phenotyping (see Additional files 3 and 4 ). Our examination focused on four non-yield phenotypes that are reported to be differentiated between upland and irrigated rice: root weight, maximal main root length, tiller number, and plant height. Moreover, we also examined four yield traits, including thousand grain (1000-grain) weight, number of productive panicles, number of filled grain per panicle and number of empty grain per panicle. Under both well-watering and dry land (rainfed upland) environments, the upland accessions consistently showed significantly taller plant architecture, fewer tillers, longer main roots and heavier root weights, which supports the previously reported phenotypic differences (Table 1 , see Additional file 5 for the phenotypic difference). Regarding the yield traits, under both environments upland accessions show larger 1000-grain weight and less productive panicles as compared to irrigated accessions (Table 1 ). The numbers of filled and empty grain per panicle show no significant differences between the two ecotypes under the irrigated condition. However, under the rainfed upland condition, the upland accessions perform much better, i.e. have significantly more filled grain (Student’s t-test, P = 0.016) and less empty grain (Student’s t-test, P = 5.3e-05) per panicle than the irrigated accessions.
Phenotypic differentiation between upland and irrigated rice
*Phenotypic value is indicated by mean value ± standard deviation.
#The difference is positive when the phenotypic value in the upland environment is larger than in the irrigated environment, otherwise the difference is negative.
@ The number of productive panicles were measured at the stage of maturity, while tiller number was assessed before the earing period. Since different accessions have different heading dates, some accessions produce more tillers after we measured the tiller numbers. So the final mean numbers of productive panicles were larger than the mean tiller numbers in the irrigated condition.
∆ In the upland environment, most of the accessions have less than two tillers. When we phenotyped the number of productive panicles, we found almost all the tillers develop productive panicles, i.e. the percentage of earbearing tiller is close to 100% in the rainfed upland condition. So here in the upland environment, tiller numbers and productive panicle numbers share the same values. The tillering ability is weak in our upland condition, and no more tillers were produced at the stage of maturity.
We focus on four non-yield phenotypes in this study, including plant height, tillering number, root weight and maximal main root length, and four yield phenotypes, including thousand grain weight, number of productive panicles, number of filled grain per panicle and number of empty grain per panicle. These phenotypic data were collected under both irrigated and upland environments.
Using software SPSS statistics 17.0, we calculated the pearson correlation coefficients (ρ) between each of the four non-yield traits and each of the yield traits under upland condition (Additional file 6 ). The plant height is significantly associated with the 1000-grain weight (ρ = 0.263, P = 0.002), the number of empty grain per panicle (ρ = −0.226, P = 0.009) and the number of productive panicles (ρ = −0.2, P = 0.022). The tiller number is significantly associated with the number of productive panicles (ρ ≈ 1, P < 0.001) and the 1000-grain weight (ρ = −0.169, P = 0.05). The root weight is significantly associated with the number of filled grain per panicle (ρ = 0.295, P = 0.001). The maximal root length is significantly associated with the thousand grain weight (ρ = 0.443, P < 0.001) and the number of empty grain per panicle (ρ = −0.232, P = 0.008). Therefore all of the four non-yield traits are significantly associated with yield traits under rainfed upland condition. From the ρ values it could be speculated that larger root weight and greater root length of upland rice would contribute to net yield increase under upland condition probably because better developed root systems could contribute to better dehydration avoidance. However, the effects of plant height and tiller number on yield are complex. The larger plant height of upland accessions would possibly cause the reduction of productive panicles, but can increase 1000-grain weight and reduce the number of empty grain. The less tiller numbers of upland accessions can cause reduction of productive panicles, but would increase 1000-grain weight.
When comparing these phenotypes between the two culture conditions, we found that dry land environment can slightly reduce plant height and 1000-grain weight, but will dramatically decrease the tiller number, root weight, number of productive panicles and number of filled grain per panicle of both the upland and the irrigated ecotypes. However, the main root length and the number of empty grain per panicle responded differently to the different environments between the two ecotypes. We observed a minor decrease (−2.5%) of main root length of irrigated rice but a significant increase (+9.9%) of upland rice root length in dry land as compared with that in the irrigated condition (Table 1 , Student’s t-test, P = 0.002, Additional file 7 ), indicating an adaptive feature of upland rice. Specifically, 61 percent of the upland accessions showed increase of root length in the upland condition, while only about 38 percent of the irrigated accessions showed root length increase in the upland condition. Moreover, for the number of empty grain per panicle, we observed a slight and nonsignificant reduction of it for the irrigated accessions in the upland condition (Student’s t-test, P = 0.29, Additional file 7 ) but a dramatic decrease of it for the upland accessions (Student’s t-test, P = 5.9e-07, Additional file 7 ), suggesting another adaptive trait of upland rice. Based on the phenotypic differentiation between the upland and irrigated accessions and the correlations between the non-yield phenotypes and yield phenotypes in the upland condition, we define ‘upland adaptation’ in this study as the characteristic non-yield phenotypes of upland rice that are associated with the yield performance in upland conditions, such as plant height, tiller number, root weight and maximal root length which are directly associated with drought resistance.
SNPs calling and annotation for the 166 accessions
For the 82 irrigated and 84 upland accessions, we obtained 402,052,644 pair-end reads that passed the reads Pass Filter, amounting to around 8 G base pairs (NCBI accession code SRA066116). The re-sequencing data for 84 upland and 82 irrigated accessions was reported in our previous work [ 19 ]. Here we comprehensively analyze these re-sequencing data to determine the genetic components that might have caused the upland adaptation and phenotypic differentiation between the two ecotypes, which has been a long-term open question in rice study.
About 87 percent of these reads could be aligned to the reference genome (IRGSP/RAP build 5 Nipponbare genome) using SOAP-2.20 software [ 20 ]. The average mapping ratios of reads for the type indica and type japonica are 81% and 92%, respectively. On average, 29.54% of the reference genome was covered for each accession (ranging from 18.65% to 46.58%). The mean depth for each accession was about 0.50 × on average (ranging from 0.27 × to 0.96 ×) (see Additional file 8 ). In total, the genome coverage of the upland and irrigated population reached 41 × and 43 ×, respectively. After SNP calling and a series of filtering for quality control (see Methods), 3,571,104 high-quality SNPs were identified (Additional file 9 includes all the SNPs), of which 3,563,681 are non-singleton SNPs. By randomly choosing 56 SNPs and genotyping them via Sanger sequencing, we were able to validate our SNP calling accuracy at 96.4%. The sequencing data, though relatively low in depth, are enough to well satisfy our analyses, as we shall discuss below.
In the following analyses, only 3,029,822 SNPs with genotype data covering more than 10 individuals in both populations were used. We annotated these SNPs by classifying them into different categories according to the genomic context (see Additional file 10 ). The non-synonymous-to-synonymous ratio of SNPs is 1.32, consistent with the previously reported ratio of 1.29 in rice [ 21 ]. SNPs causing radical mutations, such as premature stop codons or frame disruptions, were also identified (Additional file 10 ).
Phylogenetics and population genetics analysis for upland rice
SNP genotypes were used in phylogenetic analysis for the 166 accessions. Additionally, 25 wild accessions from our previous data (10 O. nivara and 15 O. rufipogon , Additional file 11 ) were added to help address the phylogenetics of upland accessions [ 21 ]. We constructed the accessions’ phylogenetic tree using a Neighbor-Joining approach with 100 bootstraps (Figure 1 ). Consistent with some of the previous reports [ 21 ], our results support that Indica and Japonica usually considered as two subspecies are very different groups, as they largely clustered in respective group and both have closely related wild accessions (Figure 1 , also see Additional file 12 ). The results also show that probably the majority of current upland accessions belong to the type japonica (in our sample 76% upland accessions are japonica) (Figure 1 , Additional file 12 ), consistent with the classification of the collection center based on traditional phenotypic characteristics and previous reports that upland rice mainly pertains to Japonica [ 22 ]. The phylogenetic tree showed that all the upland japonica accessions cluster together, suggesting that upland japonica likely have a single origin. However, for the type indica, the scattering pattern of upland indica indicates that upland indica accessions may have been separately bred multiple times using different irrigated rice as the basic genetic materials.

Phylogenetic tree of rice accessions. Green, black and orange branches refer to upland, irrigated and wild accessions respectively. Analysis showed that differentiation between Indica and Japonica has existed within the wild population, since there are strains of wild rice close to both Indica and Japonica respectively, supporting the double domestication model. The tree shows multiple origins for upland rice, though the upland japonicas may bear a single origin. Bootstrap values are indicated in some of the major internal nodes. Some of the leaf nodes are labeled with the sample number of the rice accessions. ‘ru’ refers to ‘ rufipogon ’, and ‘ni’ refers to ‘ nivara ’.
Population structure in upland rice and admixture in upland indica
We use 24,890 SNPs with genotype data covering more than 100 accessions to analyze the population structure within our samples (see Methods). Principle component analysis (PCA) summarizes the population structure for the entire population (Figure 2 a, b). The top four principle components explain about 65% of the genetic variation. The huge differentiation on principle component 1 distinguishes Indica from Japonica. By contrast, genetic differentiations between upland and irrigated ecotypes seem to be much lower. The F ST value for the two ecotypes calculated based on genome-wide SNPs is only 0.06. Even in the type japonica, where the upland accessions approximate monophyly and the ecotype differentiation is clear-cut (Figure 1 , Figure 2 a), the upland and irrigated ecotypes still have a limited genetic differentiation ( F ST = 0.13), much lower than the reported differentiation between type indica and type japonica ( F ST = 0.55) [ 23 ].

Population structure estimation. PCA analysis was conducted with the top four components shown in (a) and (b) . The four components together explain about 65% of the genetic variance. (a) , Principal component 1 distinguishes the type japonica from the type indica. Both types include two ecotypes (upland and irrigated ones). The ecotype differentiation in Japonica is clear-cut. (b) , principal components 3 and 4. (c) , the population structure reported by FRAPPE. Results were shown from K = 2 to K = 5. When K = 2, there is only division of Indica and Japonica. Then wild rice is separated when K = 3. When K = 4, the ecotype differentiation in Japonica emerges, i.e. upland japonica begins to differ from irrigated japonica. Our results show that upland-irrigated ecotype differentiation is genetically lower than the Indica-Japonica differentiation. The red rectangle indicates the six upland accessions, which were previously classified as upland indica accessions but are genetically more close to the upland japonica group. The blue arrows in K = 5 panel indicates the two upland indica, GS215 and GS233, which have a large genomic proportion close to upland japonica. The red arrow indicates upland indica, GS224, which is close to wild rice.
We also investigated the population structure using FRAPPE to estimate the ancestry and population admixture of each accessions [ 24 ]. By increasing K (the number of populations) from 2 to 5, the population structure of upland and irrigated accessions are shown (Figure 2 c). For K = 2, we see a differentiation between type indica and type japonica, and this differentiation also exists for Oryza rufipogon and Oryza nivara in the wild population. When K = 3, the wild rice and the cultivars become separated. When K = 4, the division between irrigated japonica and upland japonica emerge, while the irrigated indica and upland indica still share the same structure, indicating the ecotype differentiation is higher within the type japonica than in the type indica. When K = 5, a new subgroup in upland japonica appears, suggesting some accessions in upland japonica forms a unique subgroup which is differentiated from the others in it.
From the structure analysis, we found that some upland indica accessions, though primarily classified as type indica according to their phenotypes, turn out to be quite complicated in terms of their genomic composition. For example, six accessions (GS190, GS192, GS199, GS200, GS201, GS202) (enclosed in the red rectangular in Figure 2 c), previously classified as upland indica according to their phenotypes (e.g., the seed shape, see Additional file 13 ), turn out to be more close to upland japonica (Figure 2 c), indicating that for the upland ecotype, seed shape phenotype could be misleading in distinguishing type indica from type japonica. Besides the six accessions, GS215 and GS233 also have a large genomic proportion close to upland japonica(indicated with blue arrow in Figure 2 c), which makes them cluster with japonica in the phylogenetic tree (Figure 1 , Additional file 12 ). And upland indica GS224 (Dular), indicated with a red arrow, has a large proportion of wild rice ancestry, and correspondingly it clusters with the wild rice in the phylogenetic tree (Figure 1 , Additional file 12 ). These results suggest that current upland indica group is a admixture, containing accessions of different origins. They are classified as belong to indica probably because some of their traits are more close to type indica than type japonica. Besides, the 19 upland indica accessions which remain in the indica group in the tree also seem to be polyphyletic, indicating they might originate multiple times and their genetic mechanisms for upland adaptation might be heterogenous. On the contrary, upland japonica accessions that form a monophyletic group are more likely to have similar mechanisms for upland adaption. Considering the well-known hybridization between indica and japonica, it’s probable that upland japonica and some upland indica share similar adaptation genes, while the rest upland indica might be different. Therefore, in the following study on the ecotype differentiated genes, we first use a large sample size by comparing the merged upland and irrigated population to identify genes that show strongest signals and might be shared between upland japonica and some upland indica accessions. Then we mainly focus on the type japonica to find the japonica-specific upland adaptation genes. More data and other experiments would be needed to comprehensively address the adaptation mechanism in the complicated indica group.
Ecotype differentiated genomic regions (EDRs) in genomes
EDRs probably contain genes that account for the adaptive differentiation. Using a sliding window approach to screen regions with both the top 5‰ F ST and cross-population composite likelihood ratio test (XP-CLR) scores (Methods), EDRs were identified with selective signals to narrow down the genes underlying the phenotypic differentiation between upland and irrigated rice (Figure 3 ). After merging the neighboring EDRs, we eventually identified 74 EDRs in the genome with a median length of 22.7 kb that were differentiated between the upland and irrigated rice (Additional file 14 ). Within these EDR regions, there are a total of 8980 SNPs (Additional file 15 ). Compared with the whole genome SNPs used in our analysis, the SNPs in EDRs are significantly enriched in genic regions (Chi-square test, P < 2.2e-16) (see Additional file 16 ). Among the EDR SNPs, 2623 have allele frequency differences larger than 60% between ecotypes, which we subsequently labeled “ecotype differentiated SNPs” (EDSs) (see Additional file 17 ).

EDRs scanning. (a) , F ST values were plotted against physical coordinates over the genome; the red horizontal dashed line refers to the top 5‰ threshold. (b) , XP-CLR scores were plotted against genomic coordinates; the green horizontal dashed line refers to the top 5‰ threshold. EDRs were obtained by taking the intersection of the EDRs given by the two approaches. The vertical dashed lines in (a) and (b) indicate the boundaries between two chromosomes; the vertical red arrows indicate the interesting genes shown in detail in (c - f). (c) and (d) respectively show the F ST and XP-CLR values around Os07g0449700 , an interesting gene annotated as a type A response regulator (indicated using a red arrow). (e) and (f ) respectively show the F ST and XP-CLR around Os12g0597700 , which is similar to WRKY2 and Os12g0597000 encoding a SOS3-like calcium-binding protein 1 (indicated with red arrows). All of these interesting genes have strong F ST and XP-CLR values and are biologically related to upland adaptation in rice.
Ecotype differentiated genes (EDGs) in EDRs
There are 154 genes in the EDRs, which we called EDGs (see Additional file 18 ). For these EDGs, 74 genes have EDSs in the gene regions and the other 53 genes have EDSs located within 1 kb upstream or downstream regions. By gene enrichment analysis, we found that the EDGs are enriched in several classes (Table 2 , Additional file 19 ), such as lipase containing GDSL domain class ( P = 7.49e-05), peroxidases ( P = 1.80e-03), glutathione-related classes ( P = 5.44e-08), and auxin signaling pathway ( P = 3.80e-06).
Interesting EDGs, gene families or pathways
For the EDGs of the whole population and japonica specific EDGs, the enriched gene families and interesting genes in them are shown. And their potentially functional effects are indicated.
The GDSL domain-containing lipase has been reported to be involved in response to abiotic stress, such as drought [ 25 - 27 ], indicating that the genetic differentiation of these genes in upland rice may be relevant to their adaption to dry land. Peroxidases and glutathione-related proteins bear antioxidant activity, another crucial factor in plant adaptation to a drought-like environment [ 6 , 7 ]. Furthermore, auxin, the famous growth-promoting hormone, plays an important role in determining plant architecture, and has been proved to control the formation of adventitious roots [ 8 , 28 - 30 ]. Accordingly, differences in auxin signaling between upland and irrigated rice could potentially contribute to their different architectures and root systems. Additionally, we also found several individual EDGs of interest that might also be critical in upland adaptation or the phenotypic differentiation between the two ecotypes. Os07g0449700 , a type-A response regulator, is of special interest (Figure 3 c, d). Type-A response regulators belong to ARR gene family induced by cytokinin and play important roles in cytokinin signaling [ 31 ]. Quite a few pieces of evidence have shown that ARR genes impact root and shoot development and apparent functional overlap exists among type-A ARRs [ 31 - 34 ]. Moreover, the relevance of type-A ARR gene to the root and shoot phenotypes that are differentiated between upland and irrigated rice has also received experimental support; over-expression of a type-A response regulator in transgenic rice has been shown to cause dwarfness and poorly developed root systems [ 32 ]. Hence the ecotype differentiation on this type-A ARR gene, Os07g0449700 , may be able to explain certain differences of root and plant height phenotypes between upland and irrigated rice. Further reciprocal transgenic experiments between upland and irrigated rice will eventually address the contribution of Os07g0449700 in the adaptation of upland rice.
Other EDGs were also implied by direct or indirect evidence to be relevant to upland adaptation. Os12g0597700 (Figure 3 e, f) has a WRKY DNA-binding domain and WRKY genes have been well established to be important elements in drought tolerance [ 35 - 38 ]. Os12g0597000 encodes a SOS3-like calcium-binding protein 1 and its homologs in Arabidopsis are related to responses to water deprivation [ 39 ]. Os11g0474600 encodes a terpenoid synthase, and terpenoid plant hormones have been shown to inhibit rice tillering [ 40 ]. This gene may, therefore, explain the difference in tiller numbers between upland and irrigated rice. Besides, several pentatricopeptide repeat protein-coding genes ( Os01g0646000 , Os06g0184866 , Os06g0185800 ) and two serine/threonine protein kinase genes ( Os04g0616400 , Os04g0616600 ) may also be related to drought response as these classes of genes have been reported to be involved in responses to drought/osmotic stress and root development [ 38 , 41 ]. These results illustrate how these 154 EDGs may represent a valuable candidate gene list for investigating the phenotypic differentiation of the two ecotypes and upland rice adaptation.
EDGs selected in individual populations
The investigated EDGs indicate selection during the differentiation of the two ecotypes. To further pinpoint in which population the selection occurred, we used Nielsen’s CLR test to identify possible selective sweeps in a single population (see Methods). We identified 661 selective sweep regions in the upland population with a median length of 27 kb, and 351 selective sweep regions in the irrigated population with a median length of 41 kb (Additional file 20 ). In the selected regions of these upland and irrigated populations, there are 2542 and 1285 genes, respectively. Upland rice then has twice as many genomic regions/genes selected as irrigated rice, indicating that upland rice varieties probably suffered more selection pressure imposed by adaptation or breeding. By overlapping the 154 EDGs with these potentially selected genes, we found that almost all of these selected EDGs (150/154) could be reconfirmed as selected genes using Nielsen’s CLR approach. Among these, 60 were potentially specifically selected in upland rice, 19 were potentially specifically selected in the irrigated population, while 71 were likely selected in both populations (Figure 4 , Additional file 18 ).

EDGs population assignment. Using CLR, 154 EDGs were assigned to specific populations. In total, 60 genes were selected in upland rice, 19 genes in irrigated rice, and 71 genes were selected as probably being in both populations, while four were not able to be assigned to a specific population.
Japonica-specific and Indica-specific EDGs
Genetic architectures of complex traits in rice are often both rich and complicated since they are usually heterogeneous across the subspecies [ 42 ]. In light of this, we were also interested in the ecotype-differentiated genes within the type indica or type japonica. As Figure 1 depicts, upland indica have multiple origins, and thus may be genetically heterogeneous in terms of upland adaptation. When investigating the type-specific EDRs, we accordingly mainly focused on the type japonica.
We carried out a similar pipeline to screen the EDRs in type japonica (Additional file 21 ) and found 52 EDRs with a median length of 24 kb (see Additional file 22 ). In these EDRs, 169 EDGs were identified (see Additional file 23 ), among which 47 EDGs are overlapped with the previous EDG list from the total population while the remaining 122 are japonica-specific. For these japonica-specific EDGs, the significantly enriched gene families include mitogen-activated protein kinase ( P = 1.37e-14), WRKY genes ( P = 0.03) and thioredoxin coding genes ( P = 0.04) (Table 2 ). Previous studies showed that mitogen-activated protein kinase plays an important role in response to biotic stress and root development [ 11 , 43 ]; one japonica-specific EDG of this class is very similar to blast induced kinase, which may then explain why upland rice are generally more blast tolerant than irrigated rice. Moreover, as mentioned previously, WRKY genes and thioredoxin may be critical for drought response. Intriguingly, among our list we found Os09g0410500 , a homolog to the famous maize-domestication gene, teosinte-branched 1( tb1 ), indicating this gene may also be related to the different tillering abilities displayed by the upland and irrigated types.
For the type indica, because of the admixture of upland indica only 19 upland indica accessions that remain to cluster in the indica group could be used for analysis. But the F ST between upland and irrigated accessions in indica is quite lower (0.06) than that in japonica (0.13), which means that a much larger sample would be needed to detect the ecotype differentiation in indica. However, because of the small population size of upland indica accessions in the world it is difficult to collect enough samples so far. Analysis using only the 19 upland indica accessions yield a list of 56 genes identified by intersecting F ST and XP-CLR top signals (Additional file 24 , Additional file 25 ). Hence, it is not surprising that none of the 56 genes were overlapped with the previous EDG list from the total population, considering the small sample size and possibly heterogeneous adaptation mechanisms in indica. However, we noticed that nine and four of the genes from the indica XP-CLR top signals overlap with the previous EDGs of the merged populations and EDGs of the japonica population, respectively (Additional file 26 , Additional file 27 ), indicating that some genes related to upland ecotype might be shared among some indica accessions and japonica but are more difficult to detect because of the limited and probable heterogenous samples. More experiments and data are needed to comprehensively address the indica-specific upland adaptation genes.
Largely due to the popularity and fecundity of irrigated rice, there has been limited inquiry about the phenotypes that distinguish upland rice. Some work in this area has been done by Chang et al., who investigated the rate of leaf production, tillering production, plant height, root system and resistance to drought for 11 upland varieties as explanatory factors in the different phenotypes [ 3 ]. Likewise, Ono pointed out that Japanese upland varieties are tall and low tillering with broad leaves, long panicles, drought and blast resistant, and have a less efficient utilization of fertilizer [ 44 ]. Despite interesting findings, these studies were based on only a few varieties of upland rice. To our knowledge, the sample size of the phenotypic comparisons between upland and irrigated rice in this study represents a very large sample of upland varieties to date and the sample covers most distribution regions of upland rice over the world, providing solid evidence supporting Chang et. al’s observations [ 3 ]. Our phenotyping also observed some new features, e.g., the phenomenon that dry land culture increases main root length of upland rice but decreases that of irrigated rice indicates a remarkable superiority of upland rice to adapt to an upland environment.
Beyond our thorough phenotypic analysis, this study, to our knowledge, also provides the first genomic perspective as to the phylogenetics and population structure of current upland rice. Consistent with our classification based on phenotypic characteristics and Glaszmann’s report which indicated most upland cultivars belong to the type japonica [ 22 ], phylogenetic analysis revealed that a large proportion of upland accessions belong to the type japonica, which form a monophyly. The population structure inference reveals much less genetic differentiation between the two ecotypes than exists between the type indica-japonica, which could be due to either much more frequent gene flow between ecotypes than that between Indica and Japonica, or recent origin of upland rice.
Though the sequencing depth for each accession used in our study is relatively low, the data set itself is informative enough to address the questions we wanted to address about upland rice adaptation. Firstly, the SNPs identified have a high accuracy (96.4%), even taking into account the low coverage. Secondly, the average sequencing depth for each site in the upland or irrigated population is more than 40×, providing a sufficient sample size for allele frequency estimation. Likewise, only SNP sites with the genotype determined in more than 10 individuals within each population were used for analysis such as calculating allele frequencies, and only SNP sites with no less than 100 individuals’ genotypes were used for estimating population structure. Thirdly, though the genome coverage rate for each accession is not high, for each pair-wise comparison there are enough informative sites for the distance between any two accessions to be precisely computed (see Additional file 28 ). Thus, as some previous studies have shown, we presented an interesting example substantiating the feasibility of genetic analysis with low coverage population genomic data [ 45 , 46 ].
Except for some QTL mapping efforts, very few reports have touched upon the genetic mechanisms that underlie the adaptive phenotypes of upland rice [ 47 , 48 ]. In this study, we identified some interesting candidate genes or gene families that likely contribute to the upland adaptive traits or phenotypic differentiation between the upland and irrigated ecotypes, not only providing exciting new evidence useful in harnessing the genetic adaptations of upland rice but also demonstrating the efficiency of our methodology. Though pending more experimental evidence to validate the precise phenotypic effects of all the identified alleles, a limited number of genes (around 150) provided a highly feasible list for functional investigation as well as for the upland breeding. Crucially, we would like to stress that our approach most likely caught most of the major genomic regions that account for ecotype differentiation and upland rice adaptation including those specific regions in type japonica, but identifying some indica accession specific adaptation genes still need further work and additional data.
In summary, we surveyed the phenotypes across a large sample of upland rice in both dry land and irrigated conditions, and by comparing them to irrigated rice in similar conditions we were able to demonstrate some significant phenotypic differentiations between the two ecotypes. Using high-throughput re-sequencing of a large batch of rice accessions, for the first time, to our knowledge, we clarified a single-origin pattern of upland japonica rice but a novel multiple origin pattern in upland indica accessions. With comprehensive population genetic analysis, we identified the ecotype differentiated regions and important selection genes during the differentiation between the upland and irrigated ecotypes, and more poignantly, provided great insights into upland rice adaption and the phenotypic differentiation between the two ecotypes. These findings have important implications in the spread of upland rice varieties in breeding. For example, the genes that likely account for drought resistance can be used to breed highly drought resistant varieties from the existing high-yield irrigated varieties, and concurrently, the genes likely related to tillering ability can be used to enhance the tillering ability of upland rice, a major factor limiting its yield and subsequently its widespread usage.
Investigating phenotypes of upland accessions
To assess the reported phenotypes of upland and irrigated rice, we grew both varieties. Due to the limited amount of seeds for some accessions, only 131 accessions were phenotyped (Additional file 3 ). Phenotyping was performed both in irrigated and dry land conditions (i.e., preventing submergence in water to simulate the rainfed upland environment). For the irrigated condition, seeds were germinated and seedlings then transplanted to a paddy field with submergence in water for the whole growth and development stage. For the rainfed upland condition, we conducted direct-seeding. To fully simulate the rainfed condition, no irrigation was conducted for the whole growth stage. When the rain came, we released the water to prevent submergence. According to our records, several moderate drought events occurred and caused a few individual plants death in the upland condition. For each accession, we planted 12 individuals in two rows (6 individuals in each row) with the row spacing of 30 centimeters and plant spacing of 20 centimeters. Before the earing period, we counted the tillering numbers. After the plants entered maturity, we surveyed the root weight, main root length, plant height and the yield traits. For each accession, about five individuals were randomly selected and phenotyped (Additional file 4 ). Because in the rainfed upland condition the percentage of earbearing tiller is close to 100%, in this study tiller number and productive panicle number in the upland condition share the same result.
The re-sequencing data
The re-sequencing data for the 84 upland and 82 irrigated accessions was reported by our previous work [ 19 ]. In our previous study, these data were simply used to test that a selected region containing a key enzyme gene of ABA synthesis was not due to demographic reason. They have neither been used to investigate the population structure for upland rice, nor been used to study the population differentiated regions/genes between the two ecotypes. Here we use these re-sequencing data to further exploit the genetic components that underlie the upland adaptation and phenotypic differentiation between the two ecotypes. The 84 upland and 82 irrigated accessions were obtained from the Yunnan Academy of Agricultural Sciences (YAAS), Yunnan, China. The ecotype information was documented by the genetic resources center of YAAS. Both indica and japonica were selected for either the irrigated or upland groups (Additional file 2 ). For the irrigated ecotype, we included 42 indica accessions and 40 japonica accessions. For the upland ecotype, we selected 28 indica and 56 japonica accessions since in our genetic resources center most upland rice accessions belong to the type japonica (Additional file 2 ). The origins of the accessions cover a wide geographic range (Additional file 1 ) including most major regions of upland rice distribution (South Asia, Southeast Asia, South America, West Africa, etc.). All seeds were sown under irrigated conditions. When the seedlings turned to trefoil-stage, genomic DNA was extracted from the leaves using the CTAB method [ 49 ].
Reads mapping
Our previously obtained re-sequencing data of these upland and irrigated accessions were deposited in the NCBI Short Read Archive under the accession number SRA066116 [ 19 ]. The raw reads of the wild rice came from our previous work (NCBI Short Read Archive accession number SRA023116)[ 21 ]. The IRGSP 5.0 Nipponbare genome was downloaded from the RAP-DB database ( http://rapdblegacy.dna.affrc.go.jp/download/latest/IRGSPb5.fa.masked.gz ). We mapped the short reads to the Nipponbare genome using SOAP for each accession separately [ 20 ].
Calling SNPs and SNP annotation
After mapping the reads, we used SOAPsnp -v1.02 to call SNPs for the whole population of the irrigated and upland accessions [ 50 ]. A series of filtering was conducted to ensure the quality of the SNPs. For example, the SNPs with a quality value less than 15 or with a nearby copy number > 1.5 were removed. Also we eliminated SNPs sites with depths less than 6 or larger than 300 because sites with too low a depth are prone to sequencing errors while sites with too high depths are likely located within repeat or duplication regions [ 51 ]. In order to confirm the accuracy of SNP calling, we randomly selected 60 SNPs, amplified the sequences around them with PCR and subjected them to Sanger sequencing. In our experiment, 56 of the 60 pairs of primers worked. Afterward, genomic annotation was used to assign these SNPs into different categories related to transcription and translation, including genic regions (CDS, introns, and UTRs), promoter regions and intergenic regions using our in-house PERL scripts. SNPs that resulted in premature stop codons, disrupted start/stop codons or splice donor/acceptor sites were defined as radical SNPs.
Determining SNP genotypes for each accession
For each accession, we extracted the SNP genotype information from the output of SOAPsnp. Again, we conducted a series of quality control procedures to ensure the reliability of the genotype. The consensus files contained information including the best base genotype and its average quality score, the second best base genotype and its average quality score, and the consensus genotype and its quality scores for each site, which can be used as filters [ 50 ]. We used very stringent criterion to ensure that only genotypes with a quality score > =20 (with error rate no more than 1%) were taken into consideration. More explicitly, if the average quality score of the best base is > =20 while the average quality score of the second best base is < 20, then that genotype is the best base. If both the average quality score of the best and second best base are > =20, then the genotype is heterozygous. If both the average quality score of the best and second best base are < 20 but the quality score of the consensus genotype is > =20, then the consensus genotype is used. On average 67% of the SNP genotypes were missing for each accession due to the relatively low coverage of our re-sequencing data. However, this missing data will likely not undermine our analyses because the phylogenetic analysis is based on pair-wise distances averaged over many sites and the population genetics analyses are based on allele frequencies. As such, the data are sufficient to estimate the pair-wise distances and allele frequencies. Besides the 166 accessions we re-sequenced, the genotypes of the SNPs for the wild accessions were determined using the same method.
Constructing the phylogenetic tree
Incorporating the genotype information of wild rice accessions, we use the SNP genotypes of each accession to calculate the pair-wise distances between any two accessions using PHYLIP [ 52 ]. We used default parameters to calculate the distances, i.e. using F84 model, a transition/transversion ratio of 2.0 and no Gamma distributed rates across sites. We assumed a uniform mutation rate across the genome and thus gave same weight for different SNPs. Bootstrap with 100 replicates was conducted for the whole genome SNP data set. After the genetic distances were calculated for the 100 replicate data sets, phylogenetic trees were constructed using the Neighbor-Joining (NJ) approach based on the genetic distances [ 52 ]. The consensus tree was then built using the built-in ‘consense’ program of PHYLIP with the parameters, ‘strict majority rule’ and ‘trees to be treated as unrooted’.
Population structure inference
We first conducted a PCA analysis using the procedure as reported [ 53 ]. 24890 SNPs with genotype data covering more than 100 accessions was used in the analysis. Moreover, the FRAPPE software [ 24 ], which used a frequentist approach to estimate individual admixture, was used to examine the population structure as well. The maximum iteration of EM to run is set as 10000.
Calculating F ST
The allele frequencies of SNP alleles were determined based on the accessions’ genotypes. SNPs with less than 10 individuals’ genotype information in one population were discarded when calculating the allele frequencies for that particular population. Based on the allele frequencies, we calculated the ‘ F ST ’ using the method described by Nei [ 54 ]. In the whole-genome scan, 20-kb sliding windows were used with 2-kb sliding step. By averaging the F ST values over SNP sites in each window, F ST values for the windows were obtained.
Scanning selective sweep using composite likelihood approaches
Cross-population composite likelihood ratio test (XP-CLR) and Nielsen’s composite likelihood ratio test (CLR) are two approaches for identifying selective sweep signals using SNP data sets [ 17 , 18 ]. XP-CLR incorporates the allele frequency differentiation patterns between two populations and multilocus frequency spectrum to identify selective sweeps occurring in population differentiation. The Nielsen’s CLR method uses the distribution pattern of allele frequency spectrum in a particular population to search for selective sweep signals in that population. In our analysis, we combined XP-CLR and Nielsen’s CLR using the software XP-CLR and SweepFinder, respectively. In the XP-CLR analysis, we used the allele frequencies of both upland and irrigated populations, calculating XP-CLR scores and picking the top 5‰ regions. When calculating the XP-CLR scores, we used a window size of 0.1 cM, a 2-kb grid size and a maximum SNP number of 150 for each window. By overlapping these regions of top XP-CLR scores with those of top 5‰ F ST , we obtained a conservative set of ecotype differentiation regions (EDRs) between upland and irrigated rice and the ecotype differentiated genes (EDGs) within these regions. In Nielsen’s CLR analysis, we used allele frequencies of a single population (upland or irrigated population) and found the top 5% grid points with the highest likelihood ratio (LR) values in the genome. Genes within 10 kb of these grid points were considered as the potential selection targets in either rice population [ 55 ]. Fine-scale grid points (a grid point at every 1000 nucleotides) were used when calculating the LR values.
Gene enrichment analysis for the EDGs
Gene enrichment analysis was done using chi-square test. For a specific category of genes, we calculated the gene number in the category within the whole genome using the rice gene annotation information [ 56 ]. For example, for the gene category “lipase-containing GDSL domain”, we counted the number of lipase genes annotated with this domain in the rice gene annotation database [ 56 ]. The number of genes in this category was also counted in the EDGs. A contingency table was then constructed based on the number of interesting genes in the EDGs, the total gene number of EDGs, the number of interesting genes in the whole genome, and the total gene number in the whole genome. We then conducted the Chi-square test and obtained P -values.
Accession codes
The raw sequence data of the 166 varieties has been deposited into the NCBI Short Read Archive (SRA, http://www.ncbi.nlm.nih.gov/sra/ ) under accession number SRA066116.
Abbreviations
XP-CLR: Cross-population composite likelihood ratio; CLR: Composite likelihood ratio; EDR: Ecotype differentiated region; EDG: Genes in EDR; EDS: Ecotype differentiated SNPs.
Competing interests
The authors declare that they have no competing interests.
Authors’ contributions
JL, WW, FH designed the project. FH and DT provided the rice materials. HL, JL, LM performed the sequencing, JL, ZG, WH and XL analyzed the data. BL did the phenotyping. JL, SZ, JZ, WH and BL cultivated rice. JL and WW wrote the manuscript. All authors read and approved the final manuscript.
Supplementary Material
Geographic distribution of accessions. Blue dots show the origins of these accessions, while the dot sizes roughly correspond to the numbers of the accessions from certain areas.
Basic information of the 166 sequenced accessions.
Basic information of the Phenotyped accessions.
The phenotypes of the accessions under rainfed upland condition (a) and irrigated condition (b).
Phenotype comparison between five upland and five irrigated accessions. The left five accessions are irrigated rice (in order from left to right, Hongyou 4, Diantun 502, Yunhui 290, Hexi 42, Taizhong 65). The right five accessions are upland rice (in order from left to right IRAT104, CNA4140, Sanlicun, Arias Halus, Dourado). This image indicates that the upland type generally have higher architecture, better developed roots, and fewer tillers.
The correlation between each of the four traits (root weight, maxiaml root length, tiller number and plant height) and the yeild trais (1000-grain weight, number of filled grains per panicle, number of empty grains per panicle and number of productive panicles) under the upland condition.
The maximal main root length (a) and the number of empty grain (b) under irrigated and upland conditions and statistical test.
Mean depth and coverage rate for each accession.
The SNPs for the whole population.
SNP annotation for the 166 accessions. a, using genome annotation information we classify the SNPs into intergenic SNPs and SNPs in gene region, which are further classified as SNPs in intron or UTR regions, non-synonymous SNPs and synonymous SNPs. b, SNPs causing radical mutations including disrupting stop/start codons, causing premature stop codons or disupting splicing sites.
Wild rice information.
Phylogenetics analysis of the upland accessions. Green, black and orange branches respectively refer to upland, irrigated and wild accessions. The leaf nodes are labeled with the sample number of the accessions. This tree indicates that upland rice seems to have originated multiple times for the type Indica, while upland japonicas probably derived from a single origin.
Five of the six erroneously classified accessions. (a), typical indica seed; (b), typical japonica seed; (c) The accessions, GS190(TOS2300), GS192(WAB56-125), GS199(CNA4140), GS200 (GUARANI), GS201(Dourado), GS202(TGR78), were previously classified as upland indica according to their phenotypes (i.e. seed shape, etc.). According to our whole genome phylogenetics analysis, they are more close to upland japonica. GS200 was not photographed due to seeds being unavailable.
EDR length distribution. Median length is 22721 bp.
Annotation of SNPs in EDRs. In EDRs, there are 8980 SNPs, of which 2409 are located within gene regions. In the gene regions, 241 and 266 SNPs are synonymous and non-synonymous, respectively.
Distribution pattern of SNPs in EDRs.
Ecotype differentiated SNPs (EDS) between upland and irrigated populations.
The 154 EDGs.
Enrichment analysis for interesting gene categories in EDGs and japonica-specific EDGs.
Length distribution of selective sweep regions in the upland(a) and irrigated(b) population. Median length is 27002 bp(a) and 41001 bp(b) respectively.
EDR scanning for type japonica. (a) F ST values between upland japonica and irrigated japonica were plotted against physical coordinates over the genome; the red horizontal dashed line refers to the top 5‰ threshold. (b), XP-CLR scores were plotted against genomic coordinates; the green horizontal dashed line refers to the top 5‰ threshold. EDRs were obtained by taking the intersection of the EDRs given by the two approaches. The vertical dashed lines in (a) and (b) indicate the boundaries between two chromosomes.
Length distribution of EDRs in type japonica. Median length is 24019 bp.
Detailed information of the 169 Japonica-specific EDGs.
Detailed information of the 56 Indica-specific EDGs.
EDR scanning for type indica. (a) F ST values between upland indica and irrigated indica were plotted against physical coordinates over the genome; the red horizontal dashed line refers to the top 5‰ threshold. (b), XP-CLR scores were plotted against genomic coordinates; the green horizontal dashed line refers to the top 5‰ threshold. EDRs were obtained by taking the intersection of the EDRs given by the two approaches. The vertical dashed lines in (a) and (b) indicate the boundaries between two chromosomes.
Nine genes from the indica XP-CLR top signals overlap with the previous EDGs of merged population.
Four genes from the indica XP-CLR top signals overlap with the previous EDGs of the japonica population.
The numbers of informative sites for computing the pair-wise distance between any two accessions.
Acknowledgments
The Southwest ultra-Computer Center of Chinese Academy of Sciences provided the computing resources. We would like to thank Dr. Hua Chen from the Harvard School of Public Health for his constructive suggestions about data analysis. And thank Dr. Hui Xiang for her help editing the manuscript. This work was supported by the Department of Sciences and Technology of Yunnan Province (2011BB015, 2013HA004 and 2013GA004), the Chinese 973 Program (2013CB835200, 2013CB835201).
- Khush GS. Origin, dispersal, cultivation and variation of rice. Plant Mol Biol. 1997; 35 (1–2):25–34. [ PubMed ] [ Google Scholar ]
- Chang TT VS. Varietal diversity and morpho-agronomic characteristics of upland rice. Baiios, Philippines: Major research in upland rice, International Rice Research Institute; 1975. pp. 72–90. [ Google Scholar ]
- Chang TT LC, Tagumpay O. Agronomic and growth characteristics of upland and lowland rice varieties. Baiios, Philippines: Rice breeding, International Rice Research Institute; 1972. pp. 645–661. [ Google Scholar ]
- IRRI. An Overview of Upland Rice Research. Proceedings of the 1982 Bouake. LOS BAÑOS, LAGUNA, PHILIPPINES: Ivory Coast Upland Rice Workshop, International Rice Research Institute(IRRI); 1984. pp. 1–500. [ Google Scholar ]
- Institute IRR. Major Research in Upland Rice. Baiios, Philippines: International Rice Research Institute(IRRI); 1975. [ Google Scholar ]
- Bernier J, Atlin GN, Serraj R, Kumar A, Spaner D. Review: breeding upland rice for drought resistance. 2008.
- Price AH, Steele KA, Moore BJ, Barraclough PB, Clark LJ. A combined RFLP and AFLP linkage map of upland rice (Oryza sativa L.) used to identify QTLs for root-penetration ability. Theor Appl Genet. 2000; 100 (1):49–56. [ Google Scholar ]
- Cairns JE, Audebert A, Mullins CE, Price AH. Mapping quantitative trait loci associated with root growth in upland rice (Oryza sativa L.) exposed to soil water-deficit in fields with contrasting soil properties. Field Crop Res. 2009; 114 (1):108–118. [ Google Scholar ]
- Price AH, Steele KA, Moore BJ, Jones RGW. Upland rice grown in soil-filled chambers and exposed to contrasting water-deficit regimes II. Mapping quantitative trait loci for root morphology and distribution. Field Crop Res. 2002; 76 (1):25–43. [ Google Scholar ]
- Zhu JK. Salt and drought stress signal transduction in plants. Annu Rev Plant Biol. 2002; 53 :247–273. [ PMC free article ] [ PubMed ] [ Google Scholar ]
- Thompson AJ, Jackson AC, Symonds RC, Mulholland BJ, Dadswell AR, Blake PS, Burbidge A, Taylor IB. Ectopic expression of a tomato 9-cis-epoxycarotenoid dioxygenase gene causes over-production of abscisic acid. Plant J. 2000; 23 (3):363–374. [ PubMed ] [ Google Scholar ]
- Iuchi S, Kobayashi M, Taji T, Naramoto M, Seki M, Kato T, Tabata S, Kakubari Y, Yamaguchi-Shinozaki K, Shinozaki K. Regulation of drought tolerance by gene manipulation of 9-cis-epoxycarotenoid dioxygenase, a key enzyme in abscisic acid biosynthesis in Arabidopsis. Plant J. 2001; 27 (4):325–333. [ PubMed ] [ Google Scholar ]
- Kasuga M, Liu Q, Miura S, Yamaguchi-Shinozaki K, Shinozaki K. Improving plant drought, salt, and freezing tolerance by gene transfer of a single stress-inducible transcription factor. Nat Biotechnol. 1999; 17 (3):287–291. [ PubMed ] [ Google Scholar ]
- Garg AK, Kim JK, Owens TG, Ranwala AP, Choi YD, Kochian LV, Wu RJ. Trehalose accumulation in rice plants confers high tolerance levels to different abiotic stresses. Proc Natl Acad Sci U S A. 2002; 99 (25):15898–15903. [ PMC free article ] [ PubMed ] [ Google Scholar ]
- Jang IC, Oh SJ, Seo JS, Choi WB, Song SI, Kim CH, Kim YS, Seo HS, Choi YD, Nahm BH, Kim JK. Expression of a bifunctional fusion of the Escherichia coli genes for trehalose-6-phosphate synthase and trehalose-6-phosphate phosphatase in transgenic rice plants increases trehalose accumulation and abiotic stress tolerance without stunting growth. Plant Physiol. 2003; 131 (2):516–524. [ PMC free article ] [ PubMed ] [ Google Scholar ]
- Xiong L, Yang Y. Disease resistance and abiotic stress tolerance in rice are inversely modulated by an abscisic acid-inducible mitogen-activated protein kinase. Plant Cell. 2003; 15 (3):745–759. [ PMC free article ] [ PubMed ] [ Google Scholar ]
- Chen H, Patterson N, Reich D. Population differentiation as a test for selective sweeps. Genome Res. 2010; 20 (3):393–402. [ PMC free article ] [ PubMed ] [ Google Scholar ]
- Nielsen R, Williamson S, Kim Y, Hubisz MJ, Clark AG, Bustamante C. Genomic scans for selective sweeps using SNP data. Genome Res. 2005; 15 (11):1566–1575. [ PMC free article ] [ PubMed ] [ Google Scholar ]
- Lyu J, Zhang S, Dong Y, He W, Zhang J, Deng X, Zhang Y, Li X, Li B, Huang W, Wan W, Yu Y, Li Q, Li J, Liu X, Wang B, Tao D, Zhang G, Wang J, Xu X, Hu F, Wang W. Analysis of elite variety tag SNPs reveals an important allele in upland rice. Nat Commun. 2013; 4 :2138. [ PMC free article ] [ PubMed ] [ Google Scholar ]
- Li R, Li Y, Kristiansen K, Wang J. SOAP: short oligonucleotide alignment program. Bioinformatics. 2008; 24 (5):713–714. [ PubMed ] [ Google Scholar ]
- Xu X, Liu X, Ge S, Jensen JD, Hu F, Li X, Dong Y, Gutenkunst RN, Fang L, Huang L, Li J, He W, Zhang G, Zheng X, Zhang F, Li Y, Yu C, Kristiansen K, Zhang X, Wang J, Wright M, McCouch S, Nielsen R, Wang W. Resequencing 50 accessions of cultivated and wild rice yields markers for identifying agronomically important genes. Nat Biotechnol. 2012; 30 (1):105–111. [ PubMed ] [ Google Scholar ]
- Glaszmann JC. Isozymes and classification of Asian rice varieties. Theor Appl Genet. 1987; 74 (1):21–30. [ PubMed ] [ Google Scholar ]
- Huang X, Kurata N, Wei X, Wang ZX, Wang A, Zhao Q, Zhao Y, Liu K, Lu H, Li W, Guo Y, Lu Y, Zhou C, Fan D, Weng Q, Zhu C, Huang T, Zhang L, Wang Y, Feng L, Furuumi H, Kubo T, Miyabayashi T, Yuan X, Xu Q, Dong G, Zhan Q, Li C, Fujiyama A, Toyoda A. et al. A map of rice genome variation reveals the origin of cultivated rice. Nature. 2012; 490 (7421):497–501. [ PMC free article ] [ PubMed ] [ Google Scholar ]
- Tang H, Peng J, Wang P, Risch NJ. Estimation of individual admixture: analytical and study design considerations. Genet Epidemiol. 2005; 28 (4):289–301. [ PubMed ] [ Google Scholar ]
- Hu HH, You J, Fang YJ, Zhu XY, Qi ZY, Xiong LZ. Characterization of transcription factor gene SNAC2 conferring cold and salt tolerance in rice. Plant Mol Biol. 2008; 67 (1–2):169–181. [ PubMed ] [ Google Scholar ]
- Hong JK, Choi HW, Hwang IS, Kim DS, Kim NH, du Choi S, Kim YJ, Hwang BK. Function of a novel GDSL-type pepper lipase gene, CaGLIP1, in disease susceptibility and abiotic stress tolerance. Planta. 2008; 227 (3):539–558. [ PubMed ] [ Google Scholar ]
- Gupta S, Bharalee R, Bhorali P, Bandyopadhyay T, Gohain B, Agarwal N, Ahmed P, Saikia H, Borchetia S, Kalita MC, Handique AK, Das S. Identification of drought tolerant progenies in tea by gene expression analysis. Funct Integr Genomics. 2012; 12 (3):543–563. [ PubMed ] [ Google Scholar ]
- Wu Q, Lin J, Liu JZ, Wang X, Lim W, Oh M, Park J, Rajashekar CB, Whitham SA, Cheng NH, Hirschi KD, Park S. Ectopic expression of Arabidopsis glutaredoxin AtGRXS17 enhances thermotolerance in tomato. Plant Biotechnol J. 2012; 10 (8):945–955. [ PubMed ] [ Google Scholar ]
- Price AH, Steele KA, Gorham J, Bridges JM, Moore BJ, Evans JL, Richardson P, Jones RGW. Upland rice grown in soil-filled chambers and exposed to contrasting water-deficit regimes I. Root distribution, water use and plant water status. Field Crop Res. 2002; 76 (1):11–24. [ Google Scholar ]
- Liu DD, Dong QL, Fang MJ, Chen KQ, Hao YJ. Ectopic expression of an apple apomixis-related gene MhFIE induces co-suppression and results in abnormal vegetative and reproductive development in tomato. J Plant Physiol. 2012; 169 (18):1866–1873. [ PubMed ] [ Google Scholar ]
- To JP, Haberer G, Ferreira FJ, Deruere J, Mason MG, Schaller GE, Alonso JM, Ecker JR, Kieber JJ. Type-A Arabidopsis response regulators are partially redundant negative regulators of cytokinin signaling. Plant Cell. 2004; 16 (3):658–671. [ PMC free article ] [ PubMed ] [ Google Scholar ]
- Hirose N, Makita N, Kojima M, Kamada-Nobusada T, Sakakibara H. Overexpression of a type-A response regulator alters rice morphology and cytokinin metabolism. Plant Cell Physiol. 2007; 48 (3):523–539. [ PubMed ] [ Google Scholar ]
- Argyros RD, Mathews DE, Chiang YH, Palmer CM, Thibault DM, Etheridge N, Argyros DA, Mason MG, Kieber JJ, Schaller GE. Type B response regulators of Arabidopsis play key roles in cytokinin signaling and plant development. Plant Cell. 2008; 20 (8):2102–2116. [ PMC free article ] [ PubMed ] [ Google Scholar ]
- Hill K, Mathews DE, Kim HJ, Street IH, Wildes SL, Chiang YH, Mason MG, Alonso JM, Ecker JR, Kieber JJ, Schaller GE. Functional characterization of type-B response regulators in the Arabidopsis cytokinin response. Plant Physiol. 2013; 162 (1):212–224. [ PMC free article ] [ PubMed ] [ Google Scholar ]
- Ulker B, Somssich IE. WRKY transcription factors: from DNA binding towards biological function. Curr Opin Plant Biol. 2004; 7 (5):491–498. [ PubMed ] [ Google Scholar ]
- Zhang Y, Wang L. The WRKY transcription factor superfamily: its origin in eukaryotes and expansion in plants. BMC Evol Biol. 2005; 5 :1. [ PMC free article ] [ PubMed ] [ Google Scholar ]
- Eulgem T, Rushton PJ, Robatzek S, Somssich IE. The WRKY superfamily of plant transcription factors. Trends Plant Sci. 2000; 5 (5):199–206. [ PubMed ] [ Google Scholar ]
- Rabello AR, Guimaraes CM, Rangel PH, da Silva FR, Seixas D, de Souza E, Brasileiro AC, Spehar CR, Ferreira ME, Mehta A. Identification of drought-responsive genes in roots of upland rice (Oryza sativa L) BMC Genomics. 2008; 9 :485. [ PMC free article ] [ PubMed ] [ Google Scholar ]
- Cheong YH, Pandey GK, Grant JJ, Batistic O, Li L, Kim BG, Lee SC, Kudla J, Luan S. Two calcineurin B-like calcium sensors, interacting with protein kinase CIPK23, regulate leaf transpiration and root potassium uptake in Arabidopsis. Plant J. 2007; 52 (2):223–239. [ PubMed ] [ Google Scholar ]
- Umehara M, Hanada A, Yoshida S, Akiyama K, Arite T, Takeda-Kamiya N, Magome H, Kamiya Y, Shirasu K, Yoneyama K, Kyozuka J, Yamaguchi S. Inhibition of shoot branching by new terpenoid plant hormones. Nature. 2008; 455 (7210):195–200. [ PubMed ] [ Google Scholar ]
- Wang H, Huang Z, Chen Q, Zhang Z, Zhang H, Wu Y, Huang D, Huang R. Ectopic overexpression of tomato JERF3 in tobacco activates downstream gene expression and enhances salt tolerance. Plant Mol Biol. 2004; 55 (2):183–192. [ PubMed ] [ Google Scholar ]
- Zhao K, Tung CW, Eizenga GC, Wright MH, Ali ML, Price AH, Norton GJ, Islam MR, Reynolds A, Mezey J, McClung AM, Bustamante CD, McCouch SR. Genome-wide association mapping reveals a rich genetic architecture of complex traits in Oryza sativa. Nat Commun. 2011; 2 :467. [ PMC free article ] [ PubMed ] [ Google Scholar ]
- Xing T, Wang XJ, Malik K, Miki BL. Ectopic expression of an Arabidopsis calmodulin-like domain protein kinase-enhanced NADPH oxidase activity and oxidative burst in tomato protoplasts. Mol Plant Microbe Interact. 2001; 14 (10):1261–1264. [ PubMed ] [ Google Scholar ]
- Ono S. Upland Rice Breeding in Japan. Japan Agricultural Research Quarterly: Tokyo; 1971. [ Google Scholar ]
- Turner TL, Bourne EC, Von Wettberg EJ, Hu TT, Nuzhdin SV. Population resequencing reveals local adaptation of Arabidopsis lyrata to serpentine soils. Nat Genet. 2010; 42 (3):260–263. [ PubMed ] [ Google Scholar ]
- Huang X, Wei X, Sang T, Zhao Q, Feng Q, Zhao Y, Li C, Zhu C, Lu T, Zhang Z, Li M, Fan D, Guo Y, Wang A, Wang L, Deng L, Li W, Lu Y, Weng Q, Liu K, Huang T, Zhou T, Jing Y, Lin Z, Buckler ES, Qian Q, Zhang QF, Li J, Han B. Genome-wide association studies of 14 agronomic traits in rice landraces. Nat Genet. 2010; 42 (11):961–967. [ PubMed ] [ Google Scholar ]
- Huang N, Courtois B, Khush GS, Lin HX, Wang GL, Wu P, Zheng KL. Association of quantitative trait loci for plant height with major dwarfing genes in rice. Heredity. 1996; 77 :130–137. [ Google Scholar ]
- Mu P, Li ZC, Li CP, Zhang HL, Wu CM, Li C, Wang XK. QTL mapping of the root traits and their correlation analysis with drought resistance using DH lines from paddy and upland rice cross. Chinese Science Bulletin. 2003; 48 (24):2718–2724. [ Google Scholar ]
- Richards E, Reichardt M, Rogers S. Preparation of genomic DNA from plant tissue. Curr Protoc Mol Biol. 2001. Chapter 2:Unit2 3. [ PubMed ]
- Li R, Li Y, Fang X, Yang H, Wang J, Kristiansen K. SNP detection for massively parallel whole-genome resequencing. Genome Res. 2009; 19 (6):1124–1132. [ PMC free article ] [ PubMed ] [ Google Scholar ]
- Lam HM, Xu X, Liu X, Chen W, Yang G, Wong FL, Li MW, He W, Qin N, Wang B, Li J, Jian M, Wang J, Shao G, Sun SS, Zhang G. Resequencing of 31 wild and cultivated soybean genomes identifies patterns of genetic diversity and selection. Nat Genet. 2010; 42 (12):1053–1059. [ PubMed ] [ Google Scholar ]
- Felsenstein J. PHYLIP -- Phylogeny Inference Package (Version 3.2) Cladistics. 1989. pp. 164–166.
- Patterson N, Price AL, Reich D. Population structure and eigenanalysis. PLoS Genet. 2006; 2 (12):e190. [ PMC free article ] [ PubMed ] [ Google Scholar ]
- Nei M. Molecular Evolutionary Genetics. New York: Columbia University Press; 1987. [ Google Scholar ]
- Williamson SH, Hubisz MJ, Clark AG, Payseur BA, Bustamante CD, Nielsen R. Localizing recent adaptive evolution in the human genome. PLoS Genet. 2007; 3 (6):e90. [ PMC free article ] [ PubMed ] [ Google Scholar ]
- Tanaka T, Antonio BA, Kikuchi S, Matsumoto T, Nagamura Y, Numa H, Sakai H, Wu J, Itoh T, Sasaki T, Aono R, Fujii Y, Habara T, Harada E, Kanno M, Kawahara Y, Kawashima H, Kubooka H, Matsuya A, Nakaoka H, Saichi N, Sanbonmatsu R, Sato Y, Shinso Y, Suzuki M, Takeda J, Tanino M, Todokoro F, Yamaquchi K. Rice Annotation Project et al. The Rice Annotation Project Database (RAP-DB): 2008 update. Nucleic Acids Res. 2008; 36 (Database issue):D1028–D1033. [ PMC free article ] [ PubMed ] [ Google Scholar ]

Progress in upland rice research
149 citations
91 citations
88 citations
67 citations
58 citations
Related Papers (5)
Ask Copilot
Related topics

IMAGES
COMMENTS
Results reveal that lowland regional rice producers are on average, significantly more technically efficient (91.7%) than their upland counterparts (84.2%). Additionally, mean TGRs associated with lowland rice farmers are higher (92.1%) than their corresponding upland producers (84.7%).
Abstract. Enhancing rice yield in upland rice systems through genetic improvement remains a major challenge in the tropics. This review aims to provide the trends on upland rice cultivation over the last 30 years and recent distribution of upland rice in the tropics, and to report progress in studies on genetic improvement for enhancing productivity in Africa, Asia, and Latin America.
In this study, we identified 25 typical upland rice accessions and assembled a high-quality genome of one of the typical upland rice varieties, IRAT109, comprising 384 Mb with a contig N50 of 19.6 Mb. Phylogenetic analysis revealed upland and lowland rice have distinct ecotype differentiation within the japonica subgroup. Comparative genomic ...
Research Paper. Improvement of Upland Rice Variety by Pyramiding Drought Tolerance QTL with Two Major Blast Resistance Genes for Sustainable Rice Production ... (Pi54 and Pi1) into the genetic background of upland rice variety Varalu. Two best improved Varalu lines, MSM-36 and MSM-60 possessing high yield under drought stress and blast ...
Parallel domestication has been widely acknowledged but its genetic basis remains largely unclear. As an important rice ecotype, upland rice was assumedly domesticated multiple times in two rice subspecies (Indica and Japonica) and provides a feasible system to explore the genetic basis of parallel domestication.To uncover the genome-wide pattern of genetic differentiation between upland and ...
Cultivated rice consists of two important ecotypes, upland and irrigated, that have respectively adapted to either dry land or irrigated cultivation. Upland rice, widely adopted in rainfed upland areas in virtue of its little water requirement, contains abundant untapped genetic resources, such as genes for drought adaptation. With water shortage exacerbated and population expanding, the need ...
Emergence of upland rice in Laos and Bhutan (Fig. 4) coincides in time and space with widespread establishment of rainfed rice agriculture in mainland Southeast Asia around 4,000 yr bp 14,42 and ...
Grain yield, yield components, biomass accumulation and nitrogen uptake of upland rice were analyzed in the 2004-2005, 2006-2006, and 2006-2007 seasons, and root length density was measured ...
Genome-wide association study (GWAS) was performed for 16 agronomic traits including nitrogen use efficiency (NUE) and yield-related components using a panel of 190 mainly japonica rice varieties and a set of 38 390 single nucleotide polymorphism (SNP) markers. This panel was evaluated under rainfed upland conditions in Madagascar in two consecutive cropping seasons with two contrasted ...
Rice research began at a range of NARIs including those of Madagascar and Nigeria in the 1920s ... A research paper published in this Special Issue showed the usefulness of models ... Growing upland rice under a conservation agriculture practice combining no-till, crop residue mulching and cover crops (e.g., stylo, pigeon pea, crotalaria) seem ...
IRRI's upland rice research has made progress in solving some of the dilemmas in the upland rice ecosystem, although many challenges remain. IRRI researchers are gaining a better understanding of the ecosystem's constraints, opportunities, and research approaches. Cultivar improvement, use of farmer participatory methods to reduce erosion, and weed management are areas where research advances ...
The most used keywords found in 2243 rice research papers published between 2001 and 2021 in Indonesia and the Philippines are food ... climate change, agriculture, upland rice, irrigation ...
Comparative Economics of Weed Management Treatments in Upland Rice at Western Mid Hill of Nepal. S. L. Bohara. Economics, Agricultural and Food Sciences. Green Reports. 2021. ISSN: 2582-6239 Abstract: Weeds cause drastic reduction on yield and quality of rice. The weeds problem is more severe in upland rice system.
The level of effectiveness of farmers on Upland FFS, CSB, and Upland Palayamanan was also assessed. The result shows that the conduct of Upland FFS and overall impressions to URDP were agreed to be very effective. Respondents stated that they gained more knowledge of upland rice farming and increase their yield.
D. Maiti N. Toppo Mukesh Nitin Binit Kumar. Environmental Science, Agricultural and Food Sciences. 2017. Upland ecology is predominantly rainfed and drought prone having nutrient-poor, well-drained, acidic soils. Direct-seeded rice (Oryza sativa L.) is the major crop beside maize (Zea mays L.), pulses…. Expand.
Upland ecosystem is prominent in most of the Indian states, covering about 13.5 per cent of the area under rice and contribute to 4 per cent of rice production. More than 90 per cent of upland ...
However, a previous oversight in upland rice research reveals little information regarding its genetic mechanisms for upland adaption, greatly hindering progress in harnessing its genetic resources for breeding and cultivation. Results. In this study, we selected 84 upland and 82 irrigated accessions from all over the world, phenotyped them ...
Agricultural and Food Sciences. 2012. TLDR. Cultivar mixtures are a promising strategy that could contribute to a more sustainable cultivation of rice under upland conditions in the context of subsistence agriculture in Madagascar, where all cropping operations are manual. 42.
Coordination Unit of the Eastern and Central African Rice Research Network (ECARRN), based in Tanzania. WARDA has its headquarters in Cotonou, Benin and regional research stations near Saint- ... Growing upland rice: a production handbook - Africa Rice Center (WARDA) 11 Thinning seedlings • At2-3weeksaftersowing,thin theseedlingsto2 ...
Progress in upland rice research. F.J. Shideler, C.V. Mendoza. 31 Dec 1985 -. About: The article was published on 1986-01-01 and is currently open access. It has received 19 citations till now. The article focuses on the topics: Upland rice.
It was found out that there are three tribes of upland farmers. These are the Blaans, the Tagakaulus and the Tibolis. These three tribes have unique practices that are in accordance with their beliefs, culture, and ecological environment. Hence, these practices are very rich and are worth preserving. The different tribes plant rice only once a ...
Direct seeded rice (DSR) systems have been considered a sustainable strategy for sustainable rice (Oryza sativa L.) production and resilience under adverse climatic conditions. Providing essential nutrition for more than 50% of the global population, there has been a significant decline in rice productivity due to climate change. The results suggest that an adoption of DSR options, without ...
The title of this most welcome publication is too modest; far from being an overview, all aspects of upland rice production have been covered, many in considerable detail. There are 41 papers from 47 individual authors plus all the international centres involved: IRRI, WARDA, IITA, CIAT and IRAT. Four base papers cover production systems and problems in Africa, Asia, India and Latin America ...