
An official website of the United States government
The .gov means it’s official. Federal government websites often end in .gov or .mil. Before sharing sensitive information, make sure you’re on a federal government site.
The site is secure. The https:// ensures that you are connecting to the official website and that any information you provide is encrypted and transmitted securely.
- Publications
- Account settings
- My Bibliography
- Collections
- Citation manager

Save citation to file
Email citation, add to collections.
- Create a new collection
- Add to an existing collection
Add to My Bibliography
Your saved search, create a file for external citation management software, your rss feed.
- Search in PubMed
- Search in NLM Catalog
- Add to Search
Genetic disease amongst the Plain community
Affiliation.
- 1 Central Pennsylvania Clinic, Belleville, Pennsylvania, USA.
- PMID: 39254670
- DOI: 10.1097/MOP.0000000000001392
Purpose of review: The purpose of this review is to highlight recent genetic studies of the Amish and Mennonite (Plain) communities. For decades, the study of genetically isolated populations has improved our understanding and management of genetic diseases that affect all populations.
Recent findings: Major themes of current genetic research of the Amish and Mennonites include new causative gene and new candidate gene discovery, phenotype expansion of previously identified genetic disease, and a target for AAV9-mediated gene therapy. Additionally, several genome-wide association studies (GWAS) examining complex traits such as dementia, cardiometabolic disease, and age-related macular degeneration have been conducted. Finally, clinically relevant studies of attitudes of the Plain community towards genetic testing and telemedicine, as well as reviews of and management suggestions for the Amish variants of propionic acidemia and APOB-associated familial hypercholesterolemia have been recently published.
Summary: Recent genetic studies of the Plain community continue to highlight the value of studying isolated populations to propel genetic disease discovery and treatment. Additionally, population-specific polygenic risk scores are needed for underrepresented and minority populations, to avoid exacerbating disparities in medical genetics care. Finally, it is important for clinicians to develop management guidelines for variants common in this rapidly growing population, such as propionic acidemia.
Copyright © 2024 Wolters Kluwer Health, Inc. All rights reserved.
PubMed Disclaimer
- Hostetler JA. Models for understanding Amish Society. In: Amish Society. 4th ed. Baltimore and London: The Johns Hopkins University Press; 1993. pp. 4–5.
- Hostetler JA. The Birth of Amish Society. In: Amish Society. 4th ed. Baltimore and London: The Johns Hopkins University Press; 1993. pp. 25–33.
- Hostetler JA. To America. In: Amish Society. 4th ed. Baltimore and London: The Johns Hopkins University Press; 1993. pp. 50, 66–67.
- Strauss KA, Puffenberger EG, Morton DH. One community's effort to control genetic disease. Am J Public Health 2012; 102:1300–1306.
- Strauss KA, Puffenberger EG. Genetics, medicine, and the plain people. Annu Rev Genomics Hum Genet 2009; 10:513–536.
LinkOut - more resources
Full text sources.
- Wolters Kluwer
Research Materials
- NCI CPTC Antibody Characterization Program
Miscellaneous
- NCI CPTAC Assay Portal
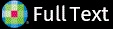
- Citation Manager
NCBI Literature Resources
MeSH PMC Bookshelf Disclaimer
The PubMed wordmark and PubMed logo are registered trademarks of the U.S. Department of Health and Human Services (HHS). Unauthorized use of these marks is strictly prohibited.

- Schools & departments

Dyslexia and ADHD share genetic links, study shows
Scientists have shed new light on the genetic basis of dyslexia, showing how it overlaps with that of attention deficit hyperactivity disorder (ADHD).

Dyslexia and ADHD often occur together in people and they share many genes in common – links which make them distinct from developmental and mental health diagnoses such as autism, bipolar disorder and schizophrenia, a study shows.
Genetic links
This study, led by the University of Edinburgh, is the first to explore the genetic links to dyslexia – believed to affect 10 per cent of the population – in the context of neurodevelopmental and psychiatric traits.
The findings could help in tailoring targeted educational, employment and wellbeing support systems for people with dyslexia or ADHD, experts say.
The findings aid understanding of the biology behind dyslexia – a difficulty with reading and spelling – and ADHD, a condition associated with difficulty concentrating, hyperactivity and impulsivity.
Public datasets
Researchers at the University of Edinburgh analysed large public anonymised datasets of genetic data on 10 neurodevelopmental and psychiatric conditions from the Psychiatric Genomics Consortium, along with dyslexia genetic statistics from an analysis of around one million people in collaboration with 23andMe, a genomics and biotechnology company.
They used a statistical tool to find clusters of genetically similar traits for dyslexia and 10 neurodevelopmental and psychiatric traits including ADHD, anorexia nervosa and Tourette syndrome.
Genetic regions
They conducted more detailed analyses to identify specific genetic regions that overlap between dyslexia and ADHD.
Among the 10 psychiatric traits included in the study, five genetically linked clusters known as latent genomic factors were identified.
ADHD was more strongly related to an attention and learning difficulties factor than with factors related to neurodevelopmental traits like autism and Tourette syndrome.
Follow-up analyses of the attention and learning difficulties factor identified 49 genetic regions and 174 genes shared between dyslexia and ADHD, of which 40 regions and 121 genes have not been previously identified.
This is the first time that genetic links to dyslexia have been studied in the context of psychiatric traits. In the future, other learning difficulties such as dyscalculia or dyspraxia should be included to allow for a more nuanced understanding of the relationships between them. Austėja Čiulkinytė A Translational Neuroscience PhD student at the University of Edinburgh, who led the study
By studying many related behaviours together we are able boost the statistical power for gene discovery. Professor Michelle Luciano School of Philosophy, Psychology and Language Sciences
The study is published in the Journal Molecular Psychiatry.
This study is in collaboration with 23andMe and supported by Wellcome, the Biotechnology and Biological Sciences Research Council and the Max Planck Society.
Link to study
School of Philosophy, Psychology and Language Sciences
Image credit -Izusek via Getty Images
- Introduction to Genomics
- Educational Resources
- Policy Issues in Genomics
- The Human Genome Project
- Funding Opportunities
- Funded Programs & Projects
- Division and Program Directors
- Scientific Program Analysts
- Contacts by Research Area
- News & Events
- Research Areas
- Research Investigators
- Research Projects
- Clinical Research
- Data Tools & Resources
- Genomics & Medicine
- Family Health History
- For Patients & Families
- For Health Professionals
- Jobs at NHGRI
- Training at NHGRI
- Funding for Research Training
- Professional Development Programs
- NHGRI Culture
- Social Media
- Broadcast Media
- Image Gallery
- Press Resources
- Organization
- NHGRI Director
- Mission and Vision
- Policies and Guidance
- Institute Advisors
- Strategic Vision
- Leadership Initiatives
- Diversity, Equity, and Inclusion
- Partner with NHGRI
- Staff Search
Genetic Disorders
Many human diseases have a genetic component. Some of these conditions are under investigation by researchers at or associated with the National Human Genome Research Institute (NHGRI).
A genetic disorder is a disease caused in whole or in part by a change in the DNA sequence away from the normal sequence. Genetic disorders can be caused by a mutation in one gene (monogenic disorder), by mutations in multiple genes (multifactorial inheritance disorder), by a combination of gene mutations and environmental factors, or by damage to chromosomes (changes in the number or structure of entire chromosomes, the structures that carry genes). As we unlock the secrets of the human genome (the complete set of human genes), we are learning that nearly all diseases have a genetic component. Some diseases are caused by mutations that are inherited from the parents and are present in an individual at birth, like sickle cell disease. Other diseases are caused by acquired mutations in a gene or group of genes that occur during a person's life. Such mutations are not inherited from a parent, but occur either randomly or due to some environmental exposure (such as cigarette smoke). These include many cancers, as well as some forms of neurofibromatosis.
List of Genetic Disorders
This list of genetic, orphan and rare diseases is provided for informational purposes only and is by no means comprehensive.
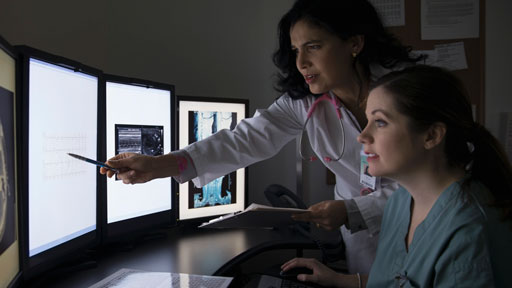
Featured Content
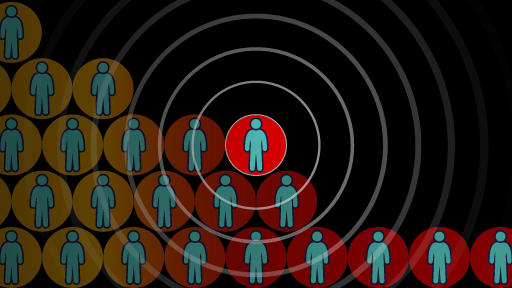
Last updated: May 18, 2018

A .gov website belongs to an official government organization in the United States.
A lock ( ) or https:// means you've safely connected to the .gov website. Share sensitive information only on official, secure websites.
- Genetics Basics
Related Topics:
- View All Home
- Family Health History
- About Cascade Testing
- Hereditary Breast and Ovarian Cancer
- Hereditary Colorectal (Colon) Cancer
- Heart Disease, Family Health History, and Familial Hypercholesterolemia
- Public Health Genomics and Precision Health Knowledge Base
Genetic Disorders
What to know.
Genetic disorders are health problems that happen because of some type of abnormality in a person's genetic material. There are several types of genetic disorders. Some disorders are caused by a genetic change (mutation) in a single gene; some are caused by an abnormality in one of the chromosomes; and some are complex, involving numerous genes and influences from environmental factors.
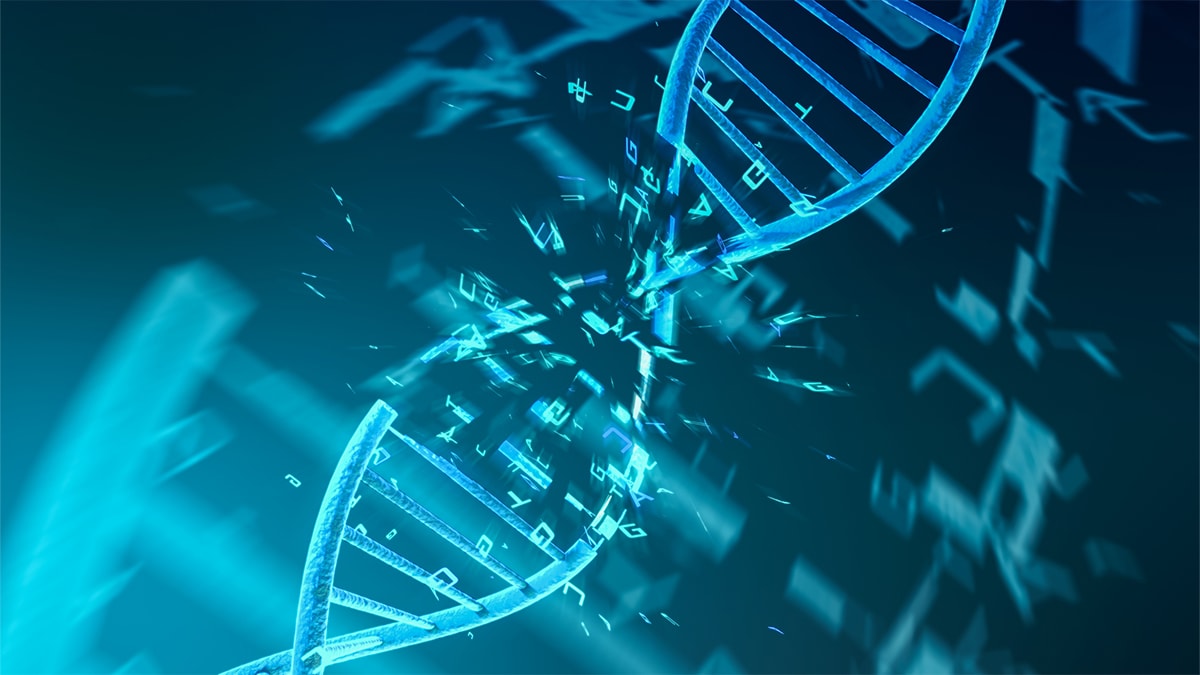
Genetic disorders are health problems that happen because of some type of abnormality in a person's genetic material. There are several types of genetic disorders. In some cases, a genetic change in a single gene can cause someone to have a disease or condition. In other cases, the gene does not have a genetic change, but a person has more or fewer copies of the gene than most people, and this causes a disease or condition. Some diseases or conditions occur when a person does not have the same number of chromosomes as most people or has part of a chromosome that is missing, extra, or not in the right place.
Most genetic disorders happen due to the combination of many genetic changes acting together with a person's behaviors and environment. These are sometimes called complex conditions.
A detailed description of the basic concepts of genetics can be found here .
Single gene disorders
DNA contains the instructions for making your body work. DNA is made up of two strands that wind around each other. Each DNA strand includes chemicals called nitrogen bases—T (thymine), A (adenine), C (cytosine), and G (guanine)—that make up the DNA code. Genes are specific sections of DNA that have instructions for making proteins. Proteins make up most of the parts of your body and make your body work the right way.
Some diseases and conditions happen when a person has a genetic change (sometimes called a mutation) in one of their genes. These types of diseases are called single gene disorders. Sometimes, what happens is that one of the DNA bases is changed. For example, part of a gene that usually has the sequence TAC is changed to the sequence TTC. This can change the way the gene works, for example, by changing the protein that is made. In other cases, one or more of the bases in the DNA sequence are missing altogether, or there are extra bases.
Genetic changes can be passed down to a child from their parents. When this happens, the disease or condition is called hereditary or inherited. Or the changes can happen for the first time in the process of making the sperm or egg or early in development, so the child will have the genetic change but the parents will not.
DNA, genes, and chromosomes
Single gene disorders that affect a gene on one of the 22 autosomal chromosome pairs are called autosomal disorders. Disorders that affect the sex chromosome are called X-linked disorders. Disorders are further described according to whether the affected genetic change is dominant or recessive.
For some diseases and conditions, everyone who inherits the genetic change will have the disease or condition, but how serious it is can vary from person to person. In other cases, people who have the genetic change will be more likely to develop the disease or condition, but some of them will never develop it.
Autosomal dominant
With autosomal dominant diseases or conditions, a person only needs a genetic change in one copy of the gene to have the disease. If one parent has an autosomal dominant disease or condition, each child has a 50% (1 in 2) chance of inheriting the genetic change that causes the condition.
Examples of autosomal dominant conditions include hereditary breast and ovarian cancer caused by genetic changes (mutations) to the BRCA1 and BRCA2 genes ; Lynch syndrome ; and familial hypercholesterolemia .
Autosomal recessive
With autosomal recessive diseases or conditions, a person needs a genetic change in both copies of the gene to have the disease or condition. While a person with a genetic change in only one copy of the gene will not have the disease or condition, they can still pass the genetic change down to their children. These parents are sometimes called "carriers" of the disease because they "carry" the genetic change that causes the disease or condition but do not have the disease themselves.
A parent who is a carrier of a disease has a 50% (1 in 2) chance of passing the gene with the genetic change on to each of their children. If both parents are carriers of the disease, each child has a 25% (1 in 4) chance of inheriting two genes with the genetic change and thus of having the disease. Carrier screening looks for autosomal recessive genetic changes in parents to see if they could have a child with the disease or condition.
Examples of autosomal recessive disorders are sickle cell disease and cystic fibrosis .
Females have two X chromosomes, and males have one X chromosome and one Y chromosome. Each daughter gets an X from her mother and an X from her father. Each son gets an X from his mother and a Y from his father.
Some diseases or conditions happen when a gene on the X chromosome has a genetic change. Because males only have one copy of all the genes on the X chromosome, they are much more likely to be affected by X-linked genetic disorders than females. A female with a genetic change on only one of her two X chromosomes may not have the disease or condition at all. However, in some cases, females with the genetic change on one of their X chromosomes can have the disease or condition, but it is often a milder form of the disease than usually occurs in males.
Because males inherit an X chromosome from their mother, a female with a genetic change on one copy of the gene has a 50% (1 in 2) chance of passing the genetic change on to each of her sons. Her sons could have the disease or condition even though she does not.
Examples of X-linked conditions include fragile X syndrome , Duchenne muscular dystrophy , and hereditary hemophilia .
Chromosomal abnormalities
Different number of chromosomes.
People usually have 23 pairs of chromosomes. But sometimes a person is born with a different number. Having an extra chromosome is called trisomy. Missing a chromosome is called monosomy.
For example, people with Down syndrome have an extra copy of chromosome 21. This extra copy changes the body's and brain's normal development and causes intellectual and physical problems for the person. Some disorders are caused by having a different number of sex chromosomes. For example, people with Turner syndrome usually have only one sex chromosome, an X. Women with Turner syndrome can have problems with growth and heart defects.
Changes in chromosomes
Sometimes chromosomes are incomplete or shaped differently than usual. Missing a small part of a chromosome is called a deletion. A translocation is when part of one chromosome has moved to another chromosome. An inversion is when part of a chromosome has been flipped over.
For example, people with Williams syndrome are missing a small part of chromosome 7. This deletion can result in intellectual disability and a distinctive facial appearance and personality.
Complex conditions
Complex disorders are caused by genetic changes in many different genes working together with environmental factors. Environmental factors include exposures and behaviors such as air pollution, smoking, alcohol use, the amount of exercise a person gets, or the foods they eat. Having a family health history of a complex condition can make you more likely to have that condition yourself. However, genetic testing would not be recommended because there is not a single genetic change causing the condition that could be found by genetic testing.
Most chronic diseases, such as most cases of heart disease , cancer , diabetes , osteoporosis , and asthma , are complex disorders. So are most cases of developmental disabilities, such as autism spectrum disorder and attention deficit / hyperactivity disorder (ADHD) , and mental health conditions, such as depression and schizophrenia .
The vast amount of genetic information available has allowed researchers to develop methods to study which types of genetic changes are found more often in people with a given disease or condition. This allows researchers to estimate a person's risk for a particular disorder based on which genetic changes they have. This estimate is known as the polygenic risk score.
Some important issues need to be considered before polygenic risk scores can be routinely used in health care and public health. Studies are looking at how useful polygenic risk scores are in real-life clinical practice. Information on how each gene change affects disease risk comes from population-level genetic studies. Addressing diversity in development of polygenic risk scores is important, because polygenic risk scores developed from studies in one population (for example, people of Northern European ancestry) might not work as well for other populations (for example, people of West African ancestry). Also, how each gene change affects the polygenic risk score varies from study to study.
Once polygenic risk scores are ready to be used routinely in clinical practice, public health efforts will be needed to address issues such as access, insurance coverage, and sharing of results across health systems.
Genomics and Your Health
Learn more about genomics and its importance for your health
BridgeBio to stop development of therapy for genetic disorder
- Medium Text
- BridgeBio Pharma Inc Follow
Sign up here.
Reporting by Puyaan Singh in Bengaluru; Editing by Shinjini Ganguli
Our Standards: The Thomson Reuters Trust Principles. , opens new tab

US household rent burden unchanged last year, varied by race, Census says
The median cost of housing for both U.S. renters and homeowners rose last year and while the share of income renters put toward housing was unchanged overall the degree to which households were cost-burdened varied by race, the Census Bureau said on Thursday.
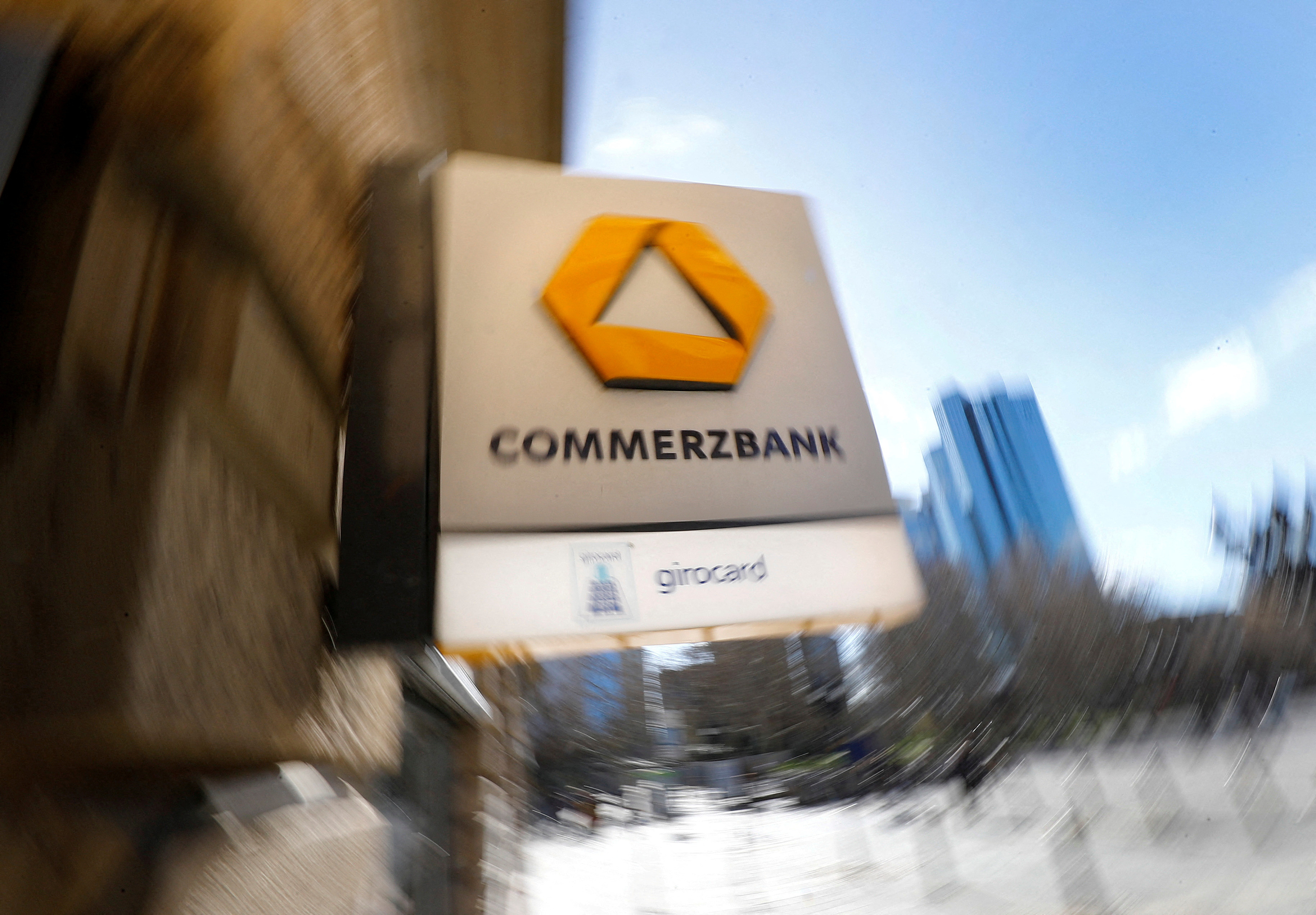

An official website of the United States government
The .gov means it’s official. Federal government websites often end in .gov or .mil. Before sharing sensitive information, make sure you’re on a federal government site.
The site is secure. The https:// ensures that you are connecting to the official website and that any information you provide is encrypted and transmitted securely.
- Publications
- Account settings
Preview improvements coming to the PMC website in October 2024. Learn More or Try it out now .
- Advanced Search
- Journal List
- Dialogues Clin Neurosci
- v.20(3); 2018 Sep
Language: English | Spanish | French
The role of genetics and genomics in clinical psychiatry
El papel de la genética y de la genómica en la psiquiatría clínica, rôles de la génétique et de la génomique en psychiatrie clinique, margret r. hoehe.
Max-Planck Institute for Molecular Genetics, Berlin, Germany
Deborah J. Morris-Rosendahl
Clinical Genetics and Genomics, Royal Brompton and Harefield NHS Foundation Trust, London, UK, NHLI, Imperial College London, UK
The enormous successes in the genetics and genomics of many diseases have provided the basis for the advancement of precision medicine. Thus, the detection of genetic variants associated with neuropsychiatric disorders, as well as treatment outcome, has raised growing expectations that these findings could soon be translated into the clinic to improve diagnosis, the prediction of disease risk and individual response to drug therapy. In this article, we will provide an introduction to the search for genes involved in psychiatric illness and summarize the present findings in major psychiatric disorders. We will review the genetic variants in genes encoding drug metabolizing enzymes and specific drug targets which were found to be associated with variable drug response and severe side effects. We will evaluate the clinical translatability of these findings, whether there is currently any role for genetic testing and in this context, make valuable sources of information available to the clinician seeking guidance and advice in this rapidly developing field of psychiatric genetics.
Los enormes éxitos en la genética y la genómica de muchas enfermedades han proporcionado la base para el avance de la medicina de precisión. Por lo tanto, la detección de variantes genéticas asociadas con trastornos neuropsiquiátricos, así como el resultado del tratamiento, han aumentado las expectativas que estos hallazgos pronto podrían traducirse en la clínica para mejorar el diagnóstico, la predicción de riesgo de enfermar y la respuesta individual a la terapia farmacológica. En este artículo, se presentará una introducción sobre la búsqueda de genes implicados en enfermedades psiquiátricas y se resumirán los hallazgos actuales en los principales trastornos psiquiátricos. Se revisarán las variantes genéticas para genes que codifican enzimas metabolizadoras de fármacos y los blancos farmacológicos específicos que se encontraron asociados con una respuesta farmacológica variable y efectos secundarios graves. Se evaluará la traducción clínica de estos hallazgos, la posibilidad actual de algún papel para las pruebas genéticas y, en ese contexto, se pondrá a disposición del clínico que busca guía y consejo, valiosas fuentes de información para este campo de rápido desarrollo que es la psiquiatría genética.
Les énormes succès obtenus en génétique et génomique dans de nombreuses maladies ont fourni la base des avancées en médecine de précision. La détection de variants génétiques, associés à des troubles neuro-psychiatriques comme à des résultats thérapeutiques, a donc soulevé une attente grandissante de voir traduire ces résultats en pratique clinique pour améliorer le diagnostic, la prédiction du risque d'avoir la maladie et la réponse individuelle au traitement médicamenteux. Nous introduisons dans cet article la recherche de gènes impliqués en pathologie psychiatrique et nous résumons les résultats actuels pour les principaux troubles psychiatriques. Nous analysons les variants génétiques des gènes codant pour les enzymes métabolisant les médicaments et les cibles médicamenteuses spécifiques ayant montré une association avec une réponse variable au médicament et des effets indésirables sévères. Nous évaluons la traduction de ces résultats en pratique clinique, la possibilité actuelle d'un rôle du dépistage génétique et, dans ce contexte, la mise à disposition du médecin en recherche d'aide et de conseils, de sources valables d'information dans ce domaine en rapide développement qu'est la psychiatrie génétique.
The search for genes underlying psychiatric illness
For decades, researchers have intensively sought to identify the underlying molecular causes of psychiatric illness. Understanding the biology of the disease, they believed, would enable valid clinical diagnosis and risk prediction, as well as a better treatment of the individual. So from the 1960s, the biological hypotheses for psychiatric diseases were focused primarily on the catecholamine and indoleamine neurotransmitter systems, which were tested by use of indirect strategies, such as neuroendocrinological challenges, as “windows to the brain.” From the mid-1980s, family, twin, and adoption studies have provided consistent evidence for aggregate genetic effects for psychiatric disorders, demonstrating the substantial role of genetic factors in the etiology of mental illness. 1 The heritability estimates for most psychiatric disorders were found to be high, between 0.4 and 0.8. 2 These results motivated efforts to search for molecular genetic variants predisposing to psychiatric disease. The first-generation molecular genetic studies were, however, largely unsuccessful. Genetic linkage studies of psychiatric disease, pre-assuming existence of single major loci or few large-effect genes, produced mostly negative or irreproducible results. Candidate gene association studies primarily focusing on synthetic, degradative, and receptor components of neurotransmitter systems proved controversial. 1 , 3
The release of a working draft of human genome sequence in 2000 marked the beginning of a new era, with enormous progress in the development of increasingly more efficient sequencing and genotyping technologies allowing the assessment of human genetic variation genome-wide, systematically, and much more completely. Exome- and genome-wide analysis in substantial numbers of individuals became feasible. Genome -wide association studies (GWAS) evolved as a key tool to identify genetic risk variants related to complex disease. This “reverse genetics” approach facilitated the identification of potentially pathogenic variants never previously conceived of, without prior pathophysiological hypothesis. Moreover, statistical methods were developed that allowed assessment of the aggregate effects of genome-wide DNA variation captured by GWAS, 1 for instance by calculating the joint contribution of common variants as a “polygene score.” 4 Finally, progress in psychiatric genetics would have been impossible without the international community combining data sets across multiple GWAS studies to maximize sample size (projecting for instance 100 000 cases for schizophrenia by 2019) and statistical power. 5 So from 2011, replicated common SNPs began to emerge from the GWAS of major psychiatric disorders, beginning with schizophrenia 6 and bipolar disorder. 7 By far the strongest GWAS signal was the association between schizophrenia and genetic markers across the Major Histocompatibility Complex (MHC) locus on chromosome 6. Through very careful molecular dissection of this complex locus, the signal on chromosome 6 was traced to the C4 gene. 8 It has been suggested that increased C4 activity in the brain of people with schizophrenia causes excessive synaptic pruning during postnatal brain development. 8 If this is supported by further work, it is one of very few times that the underlying biological process has been revealed from a GWAS signal.
Mostly facilitated by data from high density genomic arrays used in GWAS, large de novo and rare chromosomal deletions and duplications, so-called copy number variants (CNVs), began to be identified, that substantially increase risk for psychiatric disorders, especially autism spectrum disorder 9 , 10 and schizophrenia 11 , 12 but also other conditions such as attention-deficit hyperactivity disorder (ADHD). 13 Whole-exome sequencing (WES), the high throughput sequencing of all coding exons in the human genome, resulted in first (replicated) discoveries of de novo (gene-disrupting) coding mutations in autism spectrum disorder 14 - 17 and schizophrenia. 18 - 20
Taken together, the emerging architecture of psychiatric disease was found to be highly polygenic, with hundreds or even thousands of common variants of small effect size (with 1.1% to 1.2% absolute risk of illness compared with a -1.0% population risk), accounting collectively for about one third to one half of the heritability between 0.4 and 0.8. 2 Such a polygenic picture is typical for most complex traits. In addition, rare and de novo CNVs with large effect size (odds ratio ~2 to >20) as well as rare and de novo (disrupting) variants can significantly contribute to risk for major psychiatric disorders. The overall contribution of these types of variants is, however, less well understood.
There is increasing evidence for an etiological overlap between major psychiatric disorders, which would in many, though not all, instances have been predicted from their clinical presentation. 2 Major psychiatric disorders have been found to share common genetic variation, 5 , 21 , 22 with the first GWAS meta-analyses implicating neuronal/synaptic, immune and histone pathways. 23 Similarly, an overlap has been observed for rare and de novo CNVs 24 and other coding mutations. 19 , 20 The substantial overlap of genetic risk between the disorders reinforces evidence for comorbidity from earlier genetic epidemiological studies, as exemplified by an increased risk for different psychiatric disorders in relatives of a patient. 5 A recent, elegant study 25 using transcriptomic profiling in the cerebral cortex across autism, schizophrenia, bipolar disorder, depression and alcoholism, revealed patterns of shared and distinct gene-expression perturbations across these disorders. Their data suggested that common polygenic variation underlies a substantial proportion of cross-disorder expression overlap. These results underscore that psychiatric disorders as “clinical-historical constructs” 1 do not correspond to distinct definable pathophysiological entities 1 and question the value of clinical diagnostic stratification and classification.
Translating genetic findings to clinical practice
The enormous successes in genomic medicine, with the dramatic increase in the number of established gene-disease relationships for Mendelian disorders and the distinction of individual molecular tumor profiles in cancer allowing individualized diagnosis and treatment, have motivated efforts to advance precision medicine. These developments have been spurred mainly by the dramatic technological advances of the past 7 years with the implementation of next-generation sequencing (NGS) and all that it has enabled. Whereas genetic testing prior to NGS was performed primarily for very rare, single gene disorders, many of which had recurrent mutations, the advent of NGS has allowed the simultaneous interrogation of many genes and all their variants, using either targeted gene panels, WES, or whole genome sequencing (WGS).The detection of replicated genetic variants associated with neuropsychiatric disorders and treatment outcome has raised growing expectations that these results could be translated into the clinic, to improve individual diagnosis and the prediction of individual risk and treatment response, as well as predict the risk for other family members. Comprehensive genetic tests have become available, and are also commercially provided to doctors and individuals, not least by “direct-to-consumers” (DTC) testing. Thus, it is time to address the potential clinical relevance of genetic testing in psychiatry.
Prerequisites for genetic testing are analytic validity (does the test accurately detect whether a specific genetic variant is present or absent), and clinical validity (is there adequate scientific evidence to support the correlation between the genetic variant and a specific disease phenotype or risks?). Replication is critical for clinical validity. Clinical utility refers to whether the test can “provide information about diagnosis, treatment, management, or prevention of a disease that is likely to improve patient outcomes” (https://ispg.net/genetic-testing-statement/; http://www.cdc.gov/genomics/gtesting/ACCE/index.htm.). The essential prerequisite is knowledge of the genetic causes of the disorder and robust genotype-phenotype correlations, to enable for instance predictive testing for later onset disorders for family members of affected patients.
As outlined above, major adult psychiatric disorders are generally not caused by a single gene or variant, nor do they have a rare Mendelian subform as many other complex disorders do, eg, the adult-onset neurodegenerative disorders such as Alzheimer disease. On the contrary, they are complex, highly polygenic disorders involving numerous genes and variants that have only a modest impact on risk and are neither necessary nor sufficient to cause disease. This makes a clinical interpretation of the present findings at the individual level extremely difficult, if not impossible. Thus, despite tremendous progress in recent years, psychiatric genetics has, with few exceptions, not yet sufficiently advanced to be able to deduce concrete recommendations, or even clinical guidelines, for the use of genetic testing for diagnostic purposes and risk prediction. This applies in particular to major psychiatric disorders which typically begin in adult life, such as depression, bipolar disorder, substance dependence, and schizophrenia (see also https://ispg.net/genetic-testing-statement/; the 'Genetic Testing Statement' of the International Society of Psychiatric Genetics (ISPG) is being periodically updated as research progresses).
There are, however, a few circumstances where genetic testing may be useful in various clinical settings. These pertain to the analysis of variants of strong effect, such as rare or de novo CNVs and disrupting mutations, prevalent in individuals with autism spectrum disorders (ASD), schizophrenia, or other psychiatric disorders, especially when accompanied by intellectual disability. ASD not only has shared phenotypic overlap with many syndromic forms, such as Down syndrome, Prader-Willi/Angelman syndrome and Fragile X-linked intellectual disability (about 4% to 5% of ASD), 26 but is also one of the disorders for which rare variants have been demonstrated to have strong effect. The potential detection of such rare variants has made it amenable to genetic testing in one form or another. Microdeletion 22qll.l syndrome is typically caused by a recurrent 3 MB deletion of 40 genes, including TBX1. Twenty to 50% of patients with this deletion develop ASD, 27 but the deletion is also found in approximately 1% of people with schizophrenia and also in patients with bipolar disorder and idiopathic Parkinson disease. 28 , 29 Current microarrays detect an ASD-associated CNV in 7% to 10 % of cases. 30 There are now more than 50 ASD-associated CNVs and at least 61 ASD-risk genes, 18 of which have recently been identified in a comprehensive study using WGS of trios. 31 Of the 61 ASD-associated genes, 36 (59%) are associated with known syndromes/ phenotypes in OMIM (Online Mendelian Inheritance in Man, www.omim.org), with CHD8, SHANK2, and NLGN3 associated only with ASD. Many of the identified ASD-risk genes converge into shared biological pathways and networks, including synaptic and neuronal adhesion (SHANK3, SCN2A, GRIN2B, SYNGAP1, ANK2), axonal guidance, transcriptional regulation (eg, NF1, PTEN and SYNGAP1) and chromatin remodeling (eg, MECP2, MBD5, CHD8, ADNP, ARID1B and TBR1)? 1 , 32 Sixteen genes contain subdomains that could be targeted by pharmaceutical interventions and specific drug-gene interactions are known for seven genes. 31 For example, individuals with pathogenic variants in SCN2A are potential candidates for drug trials involving allosteric modulators of GABA receptors. 33
Multiple, rare CNVs have been associated with schizophrenia, all of which encompass many genes and are also common to other psychiatric and neurological disorders. 34 Approximately 2.5% of schizophrenia patients will carry one of the associated CNVs, and many more genes may be associated through more powerful sequencing studies in the near future. 35 The use of patient-parent trios to identify potentially harmful “de novo” variants has been applied to schizophrenia in a number of studies. 18 - 20 , 36 Each of these studies demonstrated an excess of damaging de novo variants in schizophrenia, particularly in glutamatergic postsynaptic proteins and proteins whose messenger RNAs are targets of the Fragile X-linked mental retardation protein, FMRP. A subsequent, combined whole-exome sequencing case-control analysis in 4264 patients, 9343 controls and 1077 trios from previous studies revealed a significant excess of very rare, gene-disrupting variants in the SETD1A gene in patients (0.19%). This was the first statistically significant association between schizophrenia and a single candidate gene, 37 although pathogenic SETD1A variants are also found in patients with more severe developmental and physical abnormalities. SETDIA is involved in histone methylation, substantiating the report that common risk variants for psychiatric disorders may aggregate on histone methylation pathways. 23
Although individually rare, the net effects of CNVs across psychiatric disorders are substantial. Specifically, the net effects of the more frequent CNVs on a broad range of psychiatric and intellectual disability- syndromes have already been sufficiently well-assessed by Malhotra et al 38 and Gershon and Alliey-Rodriguez. 39 A recent review of CNVs in schizophrenia in over 41 000 subjects by Marshall et al 34 largely confirmed previous reports of CNV associations in schizophrenia, adding suggestive evidence for six novel CNVs and providing analyses of the genes involved and of the net effects of these CNVs on schizophrenia. Although the majority of adult patients would not be expected to carry a large CNV and such CNVs mostly lack diagnostic specificity-, the identification of an inherited or de novo CNV in a known high-risk region for one of the major psychiatric disorders in such patients, may help diagnose a rare condition that has important medical and psychiatric implications for the patient and their family. Patients who carry such CNVs may find it easier to accept their diagnosis and adhere to treatment when presented with an objective “laboratory test.” 39 Siblings and offspring could be offered genetic testing and might be reassured if they do not carry the same CNV as their mentally ill relative; 39 (https://ispg.net/genetic-testing-statement/). The identification of de novo CNVs could be useful in the management of severe psychiatric disorders, especially those that present atypically or in the context of intellectual disability or certain medical syndromes (https://ispg.net/genetic-testing-statement/) .
The analysis of genes involved in variable drug response
The pharmacological treatment of psychiatric disorders has been severely hampered by a large inter-individual variation in drug response and/or severe side effects, often leading to painful, frustrating and inefficient trial-and-error-based changes of treatment regimens. This variation is to a large extent due to genetic factors, with an estimated heritability h 2 of ~0.6 - 0.8. 40 Thus, numerous studies attempted to detect gene variants associated with individually different drug responsiveness or serious side effects. Their motivation was to identify pharmacogenetic biomarkers for drug efficacy and safety, which would allow prediction of an individual's response to drug therapy and facilitate individually tailored treatment. These studies focused primarily on the analysis of candidate genes including (i) genes involved in drug metabolism (pharmacokinetics); (ii) genes encoding the specific target molecules mediating drug action (pharmacodynamics); and (iii) genes mediating severe side effects. Typically, a few up to hundreds of SNPs within these genes were genotyped in cases and controls. Furthermore, GWAS were applied to scan the genome for variants predisposing to differential drug response “hypothesis-free,” allowing detection of yet unknown genes or biological mechanisms. In view of the immense literature, we will prioritize those results which proved to be most consistent and therefore merit further consideration for potential translation in the clinic. We will focus on the pharmacogenomics of antidepressants and antipsychotics. The results essentially refer to drug-gene relationships.
Two genes of central importance in the metabolism of antidepressants and antipsychotics are those encoding cytochrome P450 (CYP) monooxygenase system enzymes, CYP2D6 and CYP2C19. 41 , 42 Variants in these genes can cause different pharmacokinetic phenotypes in individuals treated with the same dose of a drug: “ultrarapid metabolizers” (UM), characterized by significantly- reduced drug concentrations, hence decreased drug effect or non-response; “extensive metabolizers” (EM) representing the “normal” phenotype; “intermediate metabolizers” (IM), characterized by drug concentrations that are higher compared to EM; and “poor metabolizers” (PM) having the highest drug concentrations at all, resulting potentially in drug-related toxicity due to overdosing. 41 Thus, UM and PM appear to represent the clinically most relevant phenotypes/genotypes. In effect, comprehensive systematic literature reviews have substantiated evidence for lower plasma levels and an increased risk for non-response to tricyclic antidepressant treatment in UM as well as an increased risk for severe side effects in PM. 43 - 45 The same applied to antidepressant treatment with selective serotonin reuptake inhibitors (SSRI). 43 , 46 Regarding treatment with antipsychotics, the studies show a significantly increased risk for tardive dyskinesias in particular for CYP2D6-PM, while CYP2D6-UM overall does not appear to have a significant influence on antipsychotic drug response. Furthermore, a potential influence of CYP1A2 and CYP3A4 variants, other pharmacokinetic candidates of importance, on antipsychotic response has remained inconclusive. 40 , 42 , 43 , 45 Importantly, the altered activity CYP2D6 variants have been reported to exhibit substantial population differences in comprehensive global surveys. 47 - 49 Based on the first global data, 48 Europeans showed the highest fraction of CYP2D6-PM (8%) and ~3% CYP2D6-UM, while for instance 40% of the population were CYP2D6-UM in North Africa. Thus, knowledge of ethnic background is of critical clinical relevance for the development of personalized pharmacological treatment strategies. The classification of pharmacokinetic phenotypes described above is subject to constant efforts towards further standardization. Although well-established, it does not yet represent the entirety- of genetic variation, or allelic combinations. A meta-analysis of population scale sequencing projects integrating whole-genome and exome NGS data from 56 945 individuals of five major populations, demonstrated that the previous pharmacokinetic phenotype predictions from genotype data may have underestimated the prevalence of CYP2D6-PM and -IM subjects substantially. 49 Between 25.3% and 70.3% of analyzed CYP alleles contained variant combinations with no or reduced functional activity. This trend was further substantiated in a comprehensive literature review. 47 Another gene of potential importance for the pharmacokinetics of many antidepressants and some antipsychotics encodes the ATP Binding Cassette (ABC) Subfamily B Member 1 (ABCB1); this ABC transporter gene is expressed at blood-brain barrier (BBB) sites. Its membrane-associated gene product, P Glycoprotein, also known as Multidrug-Resistance Protein 1, transports various substances across the BBB out of the brain. Meta-analyses have shown associations of two (out of several) SNPs with antidepressant response. 50 , 51 Overall, however, the role of genetic variation in ABCB1 in variable antidepressant response has remained controversial and will require further examination.
Concerning the analysis of pharmacodynamic candidate genes involved in antidepressant response, a large number of studies have addressed the gene encoding the serotonin transporter (SCL6A4), a direct target for most prescribed antidepressants. The functional insertion-deletion polymorphism located in the promoter region, 5-HTTLPR, possibly was the most studied variant in relation to antidepressant response at all. Significant associations between this polymorphism and antidepressant response and remission rates were described in major meta-analyses. 52 , 53 Particularly-, a higher probability of response and remission to SSRI treatment was observed in Caucasian carriers of the long (“1”) allele, although its influence on SSRI efficacy was of modest effect. 52 Inversely, Caucasian patients with the short (“s”) allele were found to have difficulties to achieve remission and showed a reduced response to SSRI 52 , 53 as well as an increased risk for side effects. 54 Overall, however, the results are still inconsistent, precluding the use of 5-HTTLPR as a predictor of antidepressant response at present. 42 Condensing other candidate gene data of note, a comprehensive meta-analysis by 51 has suggested a significant association of variants in the serotonin 2A receptor gene (HTR2A) with antidepressant response; the same was true for variants in the gene encoding the FK506-binding protein 5 (FKBPS), which is involved in the regulation of stress hormones. Furthermore, this meta-analysis substantiated evidence that heterozygous carriers of the rs6265 polymorphism (Val66Met) in the brain-derived neurotrophic factor gene (BDNF) respond best to SSRI, particularly Asian patients. 51 Numerous other plausible candidate genes have been investigated, with controversial results and modest effect sizes overall. 42
Concerning pharmacodynamic candidate genes involved in antipsychotic treatment response, the most consistent results have been obtained for genes of the dopaminergic and serotonergic systems. 40 , 42 Thus, an insertion deletion (Ins/Del) polymorphism of the dopamine D2 receptor gene (DRD2) was found significantly associated with antipsychotic drug response, Del allele carriers exhibiting a poorer response rate than patients with the Ins/Ins genotype. 55 Moreover, a Ser9Gly polymorphism of the dopamine D3 receptor gene (DRD3) showed a consistent, though not significant trend for the Ser-allele and reduced clozapine response. 56 Also, two polymorphisms in the IITR2A gene (His452Tyr and T102C) were found associated with clozapine response. 57 Another receptor gene of the serotonergic system (HTR2C) contained a C759T polymorphism, the C-allele of which conferred a significantly increased risk for antipsychotic-induced weight gain, one of the most consistent associations observed in antipsychotics pharmacogenetics. 58 , 59 Strong candidates known to be involved in the genetics of obesity, the melanocortin 4 receptor (MC4R) and leptin genes, were also suggested to be prominent risk factors predisposing to this serious adverse effect of antipsychotics. 58 , 59 Finally, several polymorphisms of the HLA-system, specifically of HLA-B38, DR4 and DQw3 60 and HLA-DQB1 and HLA-B 61 were found associated with clozapine-induced agranulocytosis, another serious side effect of antipsychotics. For a detailed summary of the genetics of common antipsychotic-induced adverse effects see also MacNeil and Müller. 62 Numerous studies were performed with candidate genes potentially involved in lithium response, which all were inconclusive, in part also due to its unresolved underlying biology. 42
Translating pharmacogenomics to clinical practice
Pharmacogenomic studies aimed to improve individual psychiatric drug treatment through pre-emptive genotyping, which would allow adjustment of dosages to reduce the risk of overdosing and serious side effects, or a change of drug. In sum, the scientific evidence to support the clinical validity of pharmacogenetic testing is still insufficient for most gene-drug pairs. Moreover, the clinical utility of specific gene-drug pairs has not yet been clearly demonstrated in adequately powered, double-blind clinical trials, which need to be conducted to clarify whether patients benefit substantially from genotype-guided treatment compared to “treatment as usual.” Also other factors that influence treatment response such as co-medication, age, gender, disease symptoms/comorbidity, smoking and diet and, importantly, ethnic background, need to be taken into account and studied further. Despite these limitations, CYP2D6 and CYP2C19 testing has already been recommended for clinical use, 63 and guidelines for using and generating genetic information have been outlined. 64 First implementation studies using CYP2D6 and CYP2C19 genotype information in clinical practice indicated that pharmacogenetic testing was very well accepted by both physicians and patients, could particularly be beneficial for non-extensive metabolizing patients, and hold great potential for optimization of drug treatment in psychiatry. 45 , 65 Recently, the Individualized Medicine: Pharmacogenetics Assessment and Clinical Treatment (IMPACT) study was launched to demonstrate the feasibility- and utility of pharmacogenetic testing on a large scale and facilitate implementation of this testing in routine health care practice. 66
The implementation of pharmacogenomics in the clinic is supported by the establishment of comprehensive resources such as the Pharmacogenomic Knowledge Base (PharmGKB) (https://www.pharmgkb.org), and international expert groups that enable objective and transparent assessment of existing pharmacogenetic studies to derive clinical recommendations, such as the Clinical Pharmacogenomics Implementation Consortium (CPIC). Accordingly, CPIC performs a systematic review/evaluation of the comprehensive literature curated in PharmGKB to develop peer-reviewed gene-drug guidelines that are published and updated periodically (for further information on pharmacogenomics resources see Pouget et al 40 and Müller et al). 42 Thus, CPIC guidelines for CYP2D6 and CYP2C19 genotype-directed dosing of tricyclic antidepressants as well as SSRIs 44 , 46 have been published. These guidelines provide concrete information for the interpretation of genetic tests, that is, a list of existing genotypes with their “likely (pharmacokinetic) phenotypes” assigned and corresponding dosing recommendations or alternative therapeutic recommendations (suggesting selection of a drug not primarily metabolized by CYP2D6). The expert groups' recommendations are further translated by national or cross-national regulatory agencies. Thus, the US Food and Drug Administration (FDA) and other agencies distinguish for instance four categories, “required,” “recommended,” “actionable,” and “informative,” this classification of gene-drug pairs often varying between agencies and countries.
In sum, there is very good consensus concerning the pharmacogenetic testing of CYP2D6, which is “recommended” for therapy with tricyclic antidepressants with particular reference to the increased risk for serious side effects in patients with PM-status. Also the testing of CYP2C19 is considered “particularly clinically relevant.” Beyond avoiding harm, testing both CYPs is considered to improve therapy through selection of alternative drugs and provide useful information for many other diseases. Agencies such as the FDA have begun to include pharmacogenomics information in drug labeling and recommend genetic testing for now 25 psychiatric drugs. 42 As emphasized in the Genetic Testing Statement released by the ISPG, clinicians are encouraged to consider such recommendations in their treatment decisions and to “stay current on changes to drug labeling and adverse event reports” (https://ispg. net/genetic-testing-statement/). The FDA and other agencies “require” genetic testing in patients of Asian ancestry before carbamazepine treatment; carriers of the major histocompatibility allele HLA-B*15:02 are at highly increased risk to develop Stevens-Johnson syndrome (SJS), a potentially lethal skin disease. The only other “required” genetic test concerns children and adult patients who receive pimozide, an antipsychotic, to prevent side effects in CYP2D6-PM.
Conclusions and outlook
Psychiatric genetics has generated very promising results in terms of risk variants associated with major psychiatric disorders and treatment outcome. Despite these successes, psychiatry still lags behind other fields in medicine in terms of translation of existing knowledge into diagnostic genetic tests that could facilitate early diagnosis and accurate classification of disorders. The nature of genotype-phenotype-relationships has remained largely elusive, and the “fundamental biology” of psychiatric disorders has yet to be revealed. 1 , 5 Significant progress can be expected from several lines of technological advancement/development. For example, there is reason to be excited about the prospect of WGS being increasingly implemented as the assay of choice for both gene discovery and diagnostic testing in highly heterogeneous disorders. Advantages of WGS include its comprehensiveness, with the analysis of coding and non-coding sequence, the improved coverage of sequences, and in fact, of whole genes that were previously not easily sequenced, as well as the detection of all types of genetic variation. This also promises to increase diagnostic yield. Moreover, it will allow establishment of a catalogue of non-coding variation, which is assumed to contribute substantially to the development of psychiatric disorders. One could envisage a comprehensive, genome -wide panel assay, where one assesses all known variants with proven associations to psychiatric disorders in an individual patient. Since these disorders, as well as individual drug response, are complex traits which can be influenced by multiple genes, further progress can be expected through assessment of gene-gene interactions, gene networks and the application of systems approaches. 67 Complex traits are also significantly influenced by environmental factors. Thus, the analysis of the epigenome as the interface between genome and environment is expected to contribute key insights into the development of psychiatric disorders. 68 , 69 True genome-wide assessments of epigenetic marks, such as DNA methylation, or chromatin modifications have become possible, mainly also through progress in second-generation DNA sequencing methods. 69 Furthermore, the inaccessibility of the human brain can now be overcome by stem cell approaches, which make it possible to study (pluripotent stem cell-derived) neurons from patients “in a dish.” 70 The generation of CNS organoids as model systems may open new avenues towards precision drug treatment. Beyond technological advancements, a reconsideration/rethinking of previous research concepts could critically move the field forward. As outlined by Kapur et al, 71 to achieve clinical utility of diagnostic genetic testing may require a new approach. Rather than comparing prototypic patients to healthy controls, the field should focus on “identifying biologically homogeneous subtypes that cut across phenotypic diagnosis.” Validating such biomarker/genetically-defined subtypes will require longitudinal studies of individual patients, providing the “natural basis for a 'stratified' psychiatry that will improve clinical outcomes across conventional diagnostic boundaries,” ultimately more compatible with the major goal of precision medicine 71 —and the findings obtained to date.
Selected abbreviations and acronyms
CNV | Copy number variant |
OMIM | Online Inheritance in Man |
SNP | Single nucleotide polymorphism |
SNV | Single nucleotide variant |
WES | Whole exome sequencing |
WGS | Whole genome sequencing |

Update on Antisocial Personality Disorder
- Published: 04 September 2024
- Volume 26 , pages 543–549, ( 2024 )
Cite this article
- Donald W. Black 1
13 Accesses
1 Altmetric
Explore all metrics
Purpose of Review
Antisocial personality disorder (ASPD) is a characterized by lifelong or recurrent behavioral problems that begin in childhood or early adolescence. This communication provides an overview on ASPD including findings from recent reviews and new research.
Recent Findings
With regard to DSM-5’s Section III Alternative Model of Personality Disorder criteria for ASPD, advocates point to the broader symptom coverage and harmonization with ICD-11; yet critics point to the lack of evidence for improved outcomes. A new report shows that antisocial individuals age faster than non-antisocial peers. ASPD has high heritability and newer molecular studies have found intriguing linkages to genes associated with crucial brain regions. A mentalization-based therapy model has been developed and early work shows promise.
ASPD is common, widespread, and disruptive to individuals, families, and society. Chronic and lifelong, ASPD typically lessens in severity with advancing age. Assessment rests on the individual’s history because there are no diagnostic tests. ASPD likely results from an interplay of genetic and environmental factors. Brain imaging studies have linked cortical dysfunction to antisocial behavior in crucial brain regions. Medication is sometimes targeted at the individual’s aggression and irritability, but a more rational approach is to target co-occurring disorders. Cognitive-behavioral therapy and mentalization-based therapy models have been developed and are being studied.
This is a preview of subscription content, log in via an institution to check access.
Access this article
Subscribe and save.
- Get 10 units per month
- Download Article/Chapter or eBook
- 1 Unit = 1 Article or 1 Chapter
- Cancel anytime
Price excludes VAT (USA) Tax calculation will be finalised during checkout.
Instant access to the full article PDF.
Rent this article via DeepDyve
Institutional subscriptions
Similar content being viewed by others
Antisocial Personality Disorder
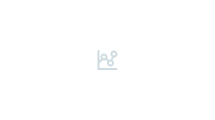
The Treatment of Antisocial Personality Disorder

Psychopathy
Data availability.
No datasets were generated or analysed during the current study.
North C, Yutzy S. Goodwin & Guze’s psychiatric diagnosis. 6th ed. New York: Oxford University Press; 2010.
Google Scholar
Black DW. Bad boys, bad men: Confronting antisocial personality disorder (sociopathy). 3rd ed. New York: Oxford University Press; 2022.
Association AP. Diagnostic and statistical manual of mental disorders. 3rd ed. Washington, D.C.: American Psychiatric Association; 1980.
Robins LN. Deviant children grown up. Baltimore: Williams and Wilkins; 1966.
Association AP. Diagnostic and statistical manual of mental disorders. 5th ed. Washington, D.C.: American Psychiatric Association; 2013.
Book Google Scholar
Association AP. Diagnostic and statistical manual of mental disorders. 5th ed. text revision. Washington, DC: American Psychiatric Association; 2022.
Goldstein RB. Epidemiology of antisocial personality disorder. In: Black DW, Kolla NJ, editors. Textbook of antisocial personality disorder. Washington, D.C.: American Psychiatric Publishing; 2022. p. 29–56.
Widiger TA, Hines A. The diagnostic and statistical manual of mental disorders fifth edition alternative model of personality disorder. Person Disord Theory Res Treat. 2022;13:347–55.
Article Google Scholar
Zimmerman M. Should the demonstration of improved patient outcome be necessary to overhaul diagnostic approaches? Comment on Bach and Tracy. Person Disord Theory Res Treat. 2022;13:387–91.
Black DW, Blum N. Clinical symptoms and assessment of antisocial personality disorder. In: Black DW, Kolla NJ, editors. Textbook of antisocial personality disorder. Washington, D.C.: American Psychiatric Publishing; 2022. p. 83–96.
Tellegen A, Ben-Porath YS, McNulty JL, Arbisi PA, Graham JR, Kaemmer B. The MMPI-2 restructured clinical scales: Development, validation, and interpretation. Minneapolis: University of Minnesota Press; 2003.
Hare RD, Neumann CS. The PCL-R assessment of psychopathy: Development, structural properties, and new developments. In: Patrick CJ, editor. Handbook of psychopathy. New York: Guilford; 2006. p. 58–90.
McGonigal PT, Zimmerman M, Scalora MJ. Psychiatric and medical comorbidity of antisocial personality disorder. In: Black DW, Kolla NJ, editors. Textbook of antisocial personality disorder. Washington, D.C.: American Psychiatric Publishing; 2022. p. 57–82.
Compton WM, Conway KP, Stinson FS, Colliver JD, Grant B. Prevalence, correlates, and comorbidity of DSM-IV antisocial personality syndromes and alcohol and specific drug use disorders in the United States: Results from the National Epidemiologic Survey on Alcohol and Related Conditions. J Clin Psychiatry. 2005;66:677–85.
Article PubMed Google Scholar
Ullrich S, Coid J. Antisocial personality disorder: co-morbid Axis I mental disorders and health service use among a national household population. Person Ment Health. 2009;3:151–64.
Black DW, Goldstein RB. Natural history and course of antisocial personality disorder. In: Black DW, Kolla NJ, editors. Textbook of antisocial personality disorder. Washington, D.C.: American Psychiatric Publishing; 2022. p. 99–111.
Black DW, Baumgard CH, Bell SE. A 16- to 45-year follow-up of 71 men with antisocial personality disorder. Compr Psychiatry. 1995;36:130–40.
Article PubMed CAS Google Scholar
Krasnova A, Eaton WW, Samuels JF. Antisocial personality and risks of cause-specific mortality: results from the Epidemiologic Catchment Area study with 27 years of follow up. Soc Psychiatry Psychatr Epidemiol. 2019;54:617–25.
Langevin S, Caspi A, Barnes JC, Brennan G, Poulton R, Purdy SC, et al. Life-course persistent antisocial behavior and accelerated biological aging in a longitudinal birth cohort. Int J Environ Res Pub Health. 2022;19:14402.
Article CAS Google Scholar
Rosenström T, Ystrom E, Torvik AK, Czajkowski NO, Gillespie NA, et al. Genetic and environmental structure of DSM-IV criteria for antisocial personality disorder: A twin study. Behav Genet. 2017;47:265–77.
Article PubMed PubMed Central Google Scholar
Slutske WS, Davis CN. Family, twin, and adoption studies in antisocial personality disorder and antisocial behavior. In: Black DW, Kolla NJ, editors. Textbook of antisocial personality disorder. Washington, D.C.: American Psychiatric Publishing; 2022. p. 113–35.
Brennan PA, Mednick SA. Genetic perspectives on crime. Acta Psychiatr Scand. 1993;370:19–26.
Cadoret RJ, O’Gorman TW, Troughton E, Heywood E. Alcoholism and antisocial personality: Interrelationships, genetic and environmental factors. Arch Gen Psychiatry. 1985;42:161–7.
Slutske WS. The genetics of antisocial behavior. Curr Psychiatry Rep. 2001;3:158–62.
Kolla NJ, Bortolato M. The role of monoamine oxidase A in the neurobiology of aggression, antisocial, and violent behavior: A tale of mice and men. Prog Neurobiol. 2020;194:101875.
Article PubMed PubMed Central CAS Google Scholar
Caspi A, McClay J, Moffitt TE, Mill J, Martin J, Craig IW, et al. Role of genotype in the cycle of violence in maltreated children. Sci. 2002;297:851–4.
Tielbeek JJ, Medland SE, Benyamin B, Byrne EM, Heath AC, Madden PAF, et al. Unraveling the genetic etiology of adult antisocial behavior: a genome-wide association study. PLoS ONE. 2012;7:e45086.
Rautianen MR, Paunio T, Repo-Tiihonen E, Virkkunen M, Ollila HM, Sulkava S, et al. Genome-wide association study of antisocial personality disorder. Transl Psychiatry. 2016;6:e883.
Tiekbeek JJ, Uffelmann E, Williams BS, Colodno-Conde L, Gagnon E, Mallard TT, Levitt BE, et al. Uncovering the genetic architecture of broad antisocial behavior through genome-wide association study meta-analysis. Mol Psychiatry. 2022;11:4453–63.
Hare RD. Twenty years of experience with the Cleckley psychopath. In: Reid WJ, Dorr D, Walker JI, Bonner JW, editors. Unmasking the psychopath—Antisocial personality and related syndromes. New York: WW Norton; 1986. p. 3–27.
Raine A. Biosocial studies of antisocial and violent behavior in children and adults: a review. J Abnorm Child Psychol. 2002;11:311–26.
Raine A, Venables PH, Williams M. Relationship between central and autonomic measures of arousal at age 15 years and criminality at age 24 years. Arch Gen Psychiatry. 1990;47:1003–7.
Moffitt T. The neuropsychology of conduct disorder. Devel Psychopathol. 1993;5:135–51.
Wakschlag LS, Lahey BB, Loeber R, Green SM, Gordon RA, Leventhal BL. Maternal smoking during pregnancy and risk of conduct disorder in boys. Arch Gen Psychiatry. 1997;54:670–6.
Neugebauer R, Hoek HW, Susser E. Prenatal exposure to wartime famine and development of antisocial personality disorder in early adulthood. JAMA. 1999;282:455–62.
Lam J, Ruocco AC. Biological risk factors for antisocial personality disorder. In: Black DW, Kolla NJ, editors. Textbook of antisocial personality disorder. Washington, D.C.: American Psychiatric Publishing; 2022. p. 161–83.
Virkkunen M, Nuutila A, Goodwin FK, Linoila M. Cerebrospinal fluid monoamine metabolite levels in male arsonists. Arch Gen Psychiatry. 1987;44:241–7.
Åsberg M, Träskman L, Thoren P. 5-HIAA in the cerebrospinal fluid: a biochemical suicide predictor? Arch Gen Psychiatry. 1976;33:1193–7.
Kolla NJ, Matthews B, Wilson AA, Houle S, Bagby RM, Links P, et al. Lower monoamine oxidase-A total distribution volume in impulsive and violent offenders with antisocial personality disorder and high psychopathic traits: An [ 11 C] harmine positron emission tomography study. Neuropsychopharmacol. 2015;40:2596–603.
Tartar RE, Kirisci L, Gavaler JS, Reynolds M, Kirillova G, Clark DB, et al. Prospective study of the association between abandoned dwellings and testosterone level on the development of behaviors leading to cannabis use disorder in boys. Biol Psychiatry. 2009;65:116–21.
Dellazizzo L, Dumais A. Central and peripheral biomarkers of antisocial personality disorder. In: Black DW, Kolla NJ, editors. Textbook of antisocial personality disorder. Washington, DC: American Psychiatric Publishing; 2022. p. 215–41.
Dolan MC. What imaging tells us about violence in anti-social men. Crim Behav Ment Health. 2010;20:199–214.
Yang Y, Glenn AL, Raine A. Brain abnormalities in antisocial individuals: implications for the law. Behav Sci Law. 2008;26:65–83.
Raine R, Buchsbaum MS, LaCasse L. Brain abnormalities in murderers indicated by positron emission tomography. Biol Psychiatry. 1997;42:495–508.
Raine A, Lencz T, Bihrle S, LaCasse L, Colletti P. Reduced prefrontal grey matter volume and reduced autonomic activity and antisocial personality disorder. Arch Gen Psychiatry. 2000;57:119–27.
Raine A. Antisocial personality disorder as a neurodevelopmental disorder. Ann Rev Clin Psychol. 2018;14:259–89.
Kiehl KA, Smith AM, Hare RD, Mendrek A, Forster BB, Brink J, et al. Limbic abnormalities in affective processing by criminal psychopaths as revealed by functional magnetic resonance imaging. Biol Psychiatry. 2001;50:677–84.
Kiehl KA, Smith AM, Mendrek A, Forster BB, Hare RD, Liddle PF. Temporal lobe abnormalities in semantic processing by criminal psychopaths as revealed by functional magnetic resonance imaging. Psychiatry Res. 2004;130:27–42.
Yang Y, Raine A, Narr KL, Colletti P, Toga AW. Localization of deformations within the amygdala of individuals with psychopathy. Arch Gen Psychiatry. 2009;66:986–94.
Paris J, Black DW. Social theories of causation. In: Black DW, Kolla NJ, editors. Textbook of antisocial personality disorder. Washington, D.C.: American Psychiatric Publishing; 2022. p. 151–60.
Krastins A, Francis AJP, Field AM, Carr SN. Childhood predictors of adulthood antisocial personality disorder symptomatology. Austral Psychol. 2014;49:142–50.
National Institute for Clinical Excellence (NICE): Clinical Guideline 77: Antisocial personality disorder. Jan 2009. www.guidance.nice.uk/CG77 .
Khalifa N, Duggan C, Stoffers J, Huband N, Völlm BA, Ferriter M, et al. Pharmacological interventions for antisocial personality disorder. Cochrane Database of Systematic Reviews. 2010;(8):CD007667.
Moscovici M, Jones RM. Pharmacological treatment of antisocial personality disorder. In: Black DW, Kolla NJ, editors. Textbook of antisocial personality disorder. Washington, D.C.: American Psychiatric Publishing; 2022. p. 375–96.
Hirose S. Effective treatment of aggression and impulsivity in antisocial personality disorder with risperidone. Psychiatr Clin Neurosci. 2001;55:161.
Walker C, Thomas J, Allen TS. Treating impulsivity, irritability, and aggression of antisocial personality disorder with quetiapine. Int J Offender Ther Comp Criminol. 2003;20:1–11.
Cowdry RW, Gardner DL. Pharmacotherapy of borderline personality disorder: alprazolam, carbamazepine, trifluoperazine, and tranylcypromine. Arch Gen Psychiatry. 1988;45:111–9.
Davidson KM, Tyrer P, Tata P, Cooke D, Gumley A, Ford I, et al. Cognitive behaviour therapy for violent men with antisocial personality disorder in the community: an exploratory randomized controlled trial. Psychol Med. 2009;39:569–77.
Davidson KM, Halford J, Kirkwood L, Newton-Howes G. CBT for violent men with antisocial personality disorder: Reflections on the experience of carrying out therapy in MASCOT, a pilot randomized controlled trial. Personal Ment Health. 2010;4:86–95.
Bateman A. Mentalizing and group psychotherapy: A novel treatment for antisocial personality disorder. Am J Psychother. 2021;75:32–7.
Bateman A, Fonagy P. Randomized controlled trial of outpatient mentalization-based treatment versus structured clinical management for borderline personality disorder. Am J Psychiatry. 2009;166:1355–64.
Langfeldt M, Morken KTE. Antisocial personality disorder and therapeutic pessimism – how can mentalization-based treatment contribute to an increase in therapeutic optimism among healthcare professionals? Front Psychol. 2024;21(1):13204505.
Download references
Author information
Authors and affiliations.
Department of Psychiatry, University of Iowa Roy J. and Lucille A. Carver College of Medicine, and Iowa City Veterans Administration Health Care, 2-126B Medical Education Building, Iowa City, IA, 52240, USA
Donald W. Black
You can also search for this author in PubMed Google Scholar
Contributions
The author conceived and wrote the manuscript.
Corresponding author
Correspondence to Donald W. Black .
Ethics declarations
Conflicts of interest.
The authors declare no competing interests.
The review does not purport to represent the views of the Department of Veterans Affairs.
Additional information
Publisher's note.
Springer Nature remains neutral with regard to jurisdictional claims in published maps and institutional affiliations.
Rights and permissions
Reprints and permissions
About this article
Black, D.W. Update on Antisocial Personality Disorder. Curr Psychiatry Rep 26 , 543–549 (2024). https://doi.org/10.1007/s11920-024-01528-x
Download citation
Accepted : 16 August 2024
Published : 04 September 2024
Issue Date : October 2024
DOI : https://doi.org/10.1007/s11920-024-01528-x
Share this article
Anyone you share the following link with will be able to read this content:
Sorry, a shareable link is not currently available for this article.
Provided by the Springer Nature SharedIt content-sharing initiative
- Antisocial personality disorder
- Personality disorders
- Alternative model for personality disorders (AMPD)
- Genome wide studies
- Mentalization-based therapy
Advertisement
- Find a journal
- Publish with us
- Track your research
Thank you for visiting nature.com. You are using a browser version with limited support for CSS. To obtain the best experience, we recommend you use a more up to date browser (or turn off compatibility mode in Internet Explorer). In the meantime, to ensure continued support, we are displaying the site without styles and JavaScript.
- View all journals
- Explore content
- About the journal
- Publish with us
- Sign up for alerts
- Expert Review
- Published: 30 August 2024
Integrative genetic analysis: cornerstone of precision psychiatry
- Jacob Vorstman ORCID: orcid.org/0000-0002-1677-3126 1 , 2 , 3 ,
- Jonathan Sebat 4 ,
- Vincent-Raphaël Bourque 5 , 6 &
- Sébastien Jacquemont ORCID: orcid.org/0000-0001-6838-8767 5 , 7
Molecular Psychiatry ( 2024 ) Cite this article
2 Altmetric
Metrics details
- Autism spectrum disorders
The role of genetic testing in the domain of neurodevelopmental and psychiatric disorders (NPDs) is gradually changing from providing etiological explanation for the presence of NPD phenotypes to also identifying young individuals at high risk of developing NPDs before their clinical manifestation. In clinical practice, the latter implies a shift towards the availability of individual genetic information predicting a certain liability to develop an NPD (e.g., autism, intellectual disability, psychosis etc.). The shift from mostly a posteriori explanation to increasingly a priori risk prediction is the by-product of the systematic implementation of whole exome or genome sequencing as part of routine diagnostic work-ups during the neonatal and prenatal periods. This rapid uptake of genetic testing early in development has far-reaching consequences for psychiatry: Whereas until recently individuals would come to medical attention because of signs of abnormal developmental and/or behavioral symptoms, increasingly, individuals are presented based on genetic liability for NPD outcomes before NPD symptoms emerge. This novel clinical scenario, while challenging, also creates opportunities for research on prevention interventions and precision medicine approaches. Here, we review why optimization of individual risk prediction is a key prerequisite for precision medicine in the sphere of NPDs, as well as the technological and statistical methods required to achieve this ambition.
This is a preview of subscription content, access via your institution
Access options
Subscribe to this journal
Receive 12 print issues and online access
$259.00 per year
only $21.58 per issue
Buy this article
- Purchase on SpringerLink
- Instant access to full article PDF
Prices may be subject to local taxes which are calculated during checkout

Similar content being viewed by others
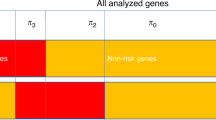
mTADA is a framework for identifying risk genes from de novo mutations in multiple traits
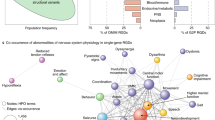
A framework for the investigation of rare genetic disorders in neuropsychiatry
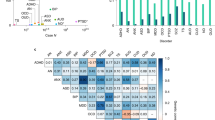
Ten challenges for clinical translation in psychiatric genetics
Carmignac V, Nambot S, Lehalle D, Callier P, Moortgat S, Benoit V, et al. Further delineation of the female phenotype with KDM5C disease causing variants: 19 new individuals and review of the literature. Clin Genet. 2020;98:43–55.
Article CAS PubMed Google Scholar
Vorstman JAS, Parr JR, Moreno-De-Luca D, Anney RJL, Nurnberger JI Jr, Hallmayer JF. Autism genetics: opportunities and challenges for clinical translation. Nat Rev Genet. 2017;18:362–76.
Trubetskoy V, Pardinas AF, Qi T, Panagiotaropoulou G, Awasthi S, Bigdeli TB, et al. Mapping genomic loci implicates genes and synaptic biology in schizophrenia. Nature. 2022;604:502–8.
Article CAS PubMed PubMed Central Google Scholar
Plomin R, von Stumm S. The new genetics of intelligence. Nat Rev Genet. 2018;19:148–59.
Yengo L, Vedantam S, Marouli E, Sidorenko J, Bartell E, Sakaue S, et al. A saturated map of common genetic variants associated with human height. Nature. 2022;610:704–12.
Brainstorm C, Anttila V, Bulik-Sullivan B, Finucane HK, Walters RK, Bras J, et al. Analysis of shared heritability in common disorders of the brain. Science. 2018;360:eaap8757.
Callahan KP, Radack J, Wojcik MH, Jenkins SM, Nye RT, Skraban C, et al. Hospital-level variation in genetic testing in children’s hospitals neonatal intensive care units from 2016 to 2021. Genet Med. 2023;25:100357.
Baribeau DA, Hoang N, Selvanayagam T, Stavropoulos DJ, Costain G, Scherer SW, et al. Developmental implications of genetic testing for physical indications. Eur J Hum Genet EJHG. 2022;30:1297–1300.
Article PubMed Google Scholar
Summers J, Baribeau D, Perlman P, Hoang N, Cui S, Krakowski A, et al. An integrated clinical approach to children at genetic risk for neurodevelopmental and psychiatric conditions: interdisciplinary collaboration and research infrastructure. J Neurodev Disord. 2024;16:37.
Article PubMed PubMed Central Google Scholar
McDonald-McGinn DM, Sullivan KE, Marino B, Philip N, Swillen A, Vorstman JA, et al. 22q11.2 deletion syndrome. Nat Rev Dis Primers. 2015;1:15071.
Schneider M, Debbane M, Bassett AS, Chow EW, Fung WL, van den Bree M, et al. Psychiatric disorders from childhood to adulthood in 22q11.2 deletion syndrome: results from the international consortium on brain and behavior in 22q11.2 deletion syndrome. Am J Psychiatry. 2014;171:627–39.
Huguet G, Schramm C, Douard E, Jiang L, Labbe A, Tihy F, et al. Measuring and estimating the effect sizes of copy number variants on general intelligence in community-based samples. JAMA Psychiatry. 2018;75:447–57.
Moreno-De-Luca A, Myers SM, Challman TD, Moreno-De-Luca D, Evans DW, Ledbetter DH. Developmental brain dysfunction: revival and expansion of old concepts based on new genetic evidence. Lancet Neurol. 2013;12:406–14.
Vorstman JA, Ophoff RA. Genetic causes of developmental disorders. Curr Opin Neurol. 2013;26:128–36.
Fiksinski AM, Hoftman GD, Vorstman JAS, Bearden CE. A genetics-first approach to understanding autism and schizophrenia spectrum disorders: the 22q11.2 deletion syndrome. Mol Psychiatry. 2023;28:341–53.
Fiksinski AM, Schneider M, Zinkstok J, Baribeau D, Chawner S, Vorstman JAS. Neurodevelopmental trajectories and psychiatric morbidity: lessons learned from the 22q11.2 deletion syndrome. Curr Psychiatry Rep. 2021;23:13.
Lee PH, Feng YA, Smoller JW. Pleiotropy and cross-disorder genetics among psychiatric disorders. Biol Psychiatry. 2021;89:20–31.
Moreau CA, Kumar K, Harvey A, Huguet G, Urchs SGW, Schultz LM, et al. Brain functional connectivity mirrors genetic pleiotropy in psychiatric conditions. Brain. 2023;146:1686–96.
Sommer IE, Bearden CE, van Dellen E, Breetvelt EJ, Duijff SN, Maijer K, et al. Early interventions in risk groups for schizophrenia: what are we waiting for? NPJ Schizophr. 2016;2:16003.
Watanabe K, Stringer S, Frei O, Umicevic Mirkov M, de Leeuw C, Polderman TJC, et al. A global overview of pleiotropy and genetic architecture in complex traits. Nat Genet. 2019;51:1339–48.
Negi SK, Guda C. Global gene expression profiling of healthy human brain and its application in studying neurological disorders. Sci Rep. 2017;7:897.
Jacquemont S, Huguet G, Klein M, Chawner S, Donald KA, van den Bree MBM, et al. Genes to mental health (G2MH): a framework to map the combined effects of rare and common variants on dimensions of cognition and psychopathology. Am J Psychiatry. 2022;179:189–203.
Chung WK, Berg JS, Botkin JR, Brenner SE, Brosco JP, Brothers KB, et al. Newborn screening for neurodevelopmental diseases: are we there yet? Am J Med Genet C Semin Med Genet. 2022;190:222–30.
Baribeau D, Vorstman J, Anagnostou E. Novel treatments in autism spectrum disorder. Curr Opin Psychiatry. 2022;35:101–10.
Lin BD, Pinzon-Espinosa J, Blouzard E, van der Horst MZ, Okhuijsen-Pfeifer C, van Eijk KR, et al. Associations between polygenic risk score loading, psychosis liability, and clozapine use among individuals with schizophrenia. JAMA Psychiatry. 2023;80:181–5.
Rolland T, Cliquet F, Anney RJL, Moreau C, Traut N, Mathieu A, et al. Phenotypic effects of genetic variants associated with autism. Nat Med. 2023;29:1671–80.
Chung WK, Roberts TP, Sherr EH, Snyder LG, Spiro JE. 16p11.2 deletion syndrome. Curr Opin Genet Dev. 2021;68:49–56.
Mollon J, Almasy L, Jacquemont S, Glahn DC. The contribution of copy number variants to psychiatric symptoms and cognitive ability. Mol Psychiatry. 2023;28:1480–93.
Mulle JG, Gambello MJ, Sanchez Russo R, Murphy MM, Burrell TL, Klaiman C et al. 3q29 Recurrent Deletion. In: Adam MP, Mirzaa GM, Pagon RA, Wallace SE, Bean LJH, Gripp KW et al. (eds). GeneReviews((R)): Seattle (WA), 1993.
McCarthy SE, Makarov V, Kirov G, Addington AM, McClellan J, Yoon S, et al. Microduplications of 16p11.2 are associated with schizophrenia. Nat Genet. 2009;41:1223–7.
Richards C, Jones C, Groves L, Moss J, Oliver C. Prevalence of autism spectrum disorder phenomenology in genetic disorders: a systematic review and meta-analysis. Lancet Psychiatry. 2015;2:909–16.
Grove J, Ripke S, Als TD, Mattheisen M, Walters RK, Won H, et al. Identification of common genetic risk variants for autism spectrum disorder. Nat. Genet. 2019;51:431–44.
Demontis D, Walters RK, Martin J, Mattheisen M, Als TD, Agerbo E, et al. Discovery of the first genome-wide significant risk loci for attention deficit/hyperactivity disorder. Nat Genet. 2019;51:63–75.
Sanders SJ, Murtha MT, Gupta AR, Murdoch JD, Raubeson MJ, Willsey AJ, et al. De novo mutations revealed by whole-exome sequencing are strongly associated with autism. Nature. 2012;485:237–41.
Singh T, Poterba T, Curtis D, Akil H, Al Eissa M, Barchas JD, et al. Rare coding variants in ten genes confer substantial risk for schizophrenia. Nature. 2022;604:509–16.
Al-Mubarak BR, Omar A, Baz B, Al-Abdulaziz B, Magrashi AI, Al-Yemni E, et al. Whole exome sequencing in ADHD trios from single and multi-incident families implicates new candidate genes and highlights polygenic transmission. Eur J Hum Genet EJHG. 2020;28:1098–110.
Malhotra D, Sebat J. CNVs: harbingers of a rare variant revolution in psychiatric genetics. Cell. 2012;148:1223–41.
Szatmari P, Paterson AD, Zwaigenbaum L, Roberts W, Brian J, Liu XQ, et al. Mapping autism risk loci using genetic linkage and chromosomal rearrangements. NatGenet. 2007;39:319–28.
CAS Google Scholar
Jiang YH, Yuen RK, Jin X, Wang M, Chen N, Wu X, et al. Detection of clinically relevant genetic variants in autism spectrum disorder by whole-genome sequencing. Am J Hum Genet. 2013;93:249–63.
Trost B, Engchuan W, Nguyen CM, Thiruvahindrapuram B, Dolzhenko E, Backstrom I, et al. Genome-wide detection of tandem DNA repeats that are expanded in autism. Nature. 2020;586:80–86.
Demontis D, Walters GB, Athanasiadis G, Walters R, Therrien K, Nielsen TT, et al. Genome-wide analyses of ADHD identify 27 risk loci, refine the genetic architecture and implicate several cognitive domains. Nat Genet. 2023;55:198–208.
Trost, Thiruvahindrapuram B, Chan AJS B, Engchuan W, Higginbotham EJ, Howe JL, et al. Genomic architecture of autism from comprehensive whole-genome sequence annotation. Cell. 2022;185:4409–27 e4418.
Antaki D, Guevara J, Maihofer AX, Klein M, Gujral M, Grove J, et al. A phenotypic spectrum of autism is attributable to the combined effects of rare variants, polygenic risk and sex. Nat Genet. 2022;54:1284–92.
Bergen SE, Ploner A, Howrigan D, Group CNVA, the Schizophrenia Working Group of the Psychiatric Genomics C, O’Donovan MC, et al. Joint contributions of rare copy number variants and common SNPs to risk for schizophrenia. Am J Psychiatry. 2019;176:29–35.
Weiner DJ, Wigdor EM, Ripke S, Walters RK, Kosmicki JA, Grove J, et al. Polygenic transmission disequilibrium confirms that common and rare variation act additively to create risk for autism spectrum disorders. Nat Genet. 2017;49:978–85.
Tansey KE, Rees E, Linden DE, Ripke S, Chambert KD, Moran JL, et al. Common alleles contribute to schizophrenia in CNV carriers. Mol Psychiatry. 2016;21:1085–9.
Calle Sanchez X, Helenius D, Bybjerg-Grauholm J, Pedersen C, Hougaard DM, Borglum AD, et al. Comparing copy number variations in a Danish case cohort of individuals with psychiatric disorders. JAMA Psychiatry. 2022;79:59–69.
Wright CF, Eberhardt RY, Constantinou P, Hurles ME, FitzPatrick DR, Firth HV, et al. Evaluating variants classified as pathogenic in ClinVar in the DDD study. Genet Med. 2021;23:571–5.
Girirajan S, Rosenfeld JA, Cooper GM, Antonacci F, Siswara P, Itsara A, et al. A recurrent 16p12.1 microdeletion supports a two-hit model for severe developmental delay. Nat Genet. 2010;42:203–9.
Williams HJ, Monks S, Murphy KC, Kirov G, O’Donovan MC, Owen MJ. Schizophrenia two-hit hypothesis in velo-cardio facial syndrome. Am J Med Genet B Neuropsychiatr Genet. 2013;162B:177–82.
Pizzo L, Jensen M, Polyak A, Rosenfeld JA, Mannik K, Krishnan A, et al. Rare variants in the genetic background modulate cognitive and developmental phenotypes in individuals carrying disease-associated variants. Genet Med. 2019;21:816–25.
Hill M, Hammond J, Lewis C, Mellis R, Clement E, Chitty LS. Delivering genome sequencing for rapid genetic diagnosis in critically ill children: parent and professional views, experiences and challenges. Eur J Hum Genet EJHG. 2020;28:1529–40.
Dimmock DP, Clark MM, Gaughran M, Cakici JA, Caylor SA, Clarke C, et al. An RCT of rapid genomic sequencing among seriously Ill infants results in high clinical utility, changes in management, and low perceived harm. Am J Hum Genet. 2020;107:942–52.
https://www.genomicsengland.co.uk/initiatives/newborns/ethics
Spiekerkoetter U, Bick D, Scott R, Hopkins H, Krones T, Gross ES, et al. Genomic newborn screening: are we entering a new era of screening? J Inherit Metab Dis. 2023;46:778–95.
Scocchia A, Wigby KM, Masser-Frye D, Del Campo M, Galarreta CI, Thorpe E, et al. Clinical whole genome sequencing as a first-tier test at a resource-limited dysmorphology clinic in Mexico. NPJ Genom Med. 2019;4:5.
Lionel AC, Costain G, Monfared N, Walker S, Reuter MS, Hosseini SM, et al. Improved diagnostic yield compared with targeted gene sequencing panels suggests a role for whole-genome sequencing as a first-tier genetic test. Genet Med. 2018;20:435–43.
Stavropoulos DJ, Merico D, Jobling R, Bowdin S, Monfared N, Thiruvahindrapuram B, et al. Whole genome sequencing expands diagnostic utility and improves clinical management in pediatric medicine. NPJ Genom Med. 2016;1:15012.
Gymrek M. A genomic view of short tandem repeats. Curr Opin Genet Dev. 2017;44:9–16.
Brandler WM, Antaki D, Gujral M, Kleiber ML, Whitney J, Maile MS, et al. Paternally inherited cis-regulatory structural variants are associated with autism. Science. 2018;360:327–31.
Brandler WM, Antaki D, Gujral M, Noor A, Rosanio G, Chapman TR, et al. Frequency and complexity of de novo structural mutation in autism. Am J Hum Genet. 2016;98:667–79.
Battle SL, Puiu D, Group TOmW, Verlouw J, Broer L, Boerwinkle E, et al. A bioinformatics pipeline for estimating mitochondrial DNA copy number and heteroplasmy levels from whole genome sequencing data. NAR Genom Bioinform. 2022;4:lqac034.
An JY, Lin K, Zhu L, Werling DM, Dong S, Brand H, et al. Genome-wide de novo risk score implicates promoter variation in autism spectrum disorder. Science. 2018;362:eaat6576.
Polygenic Risk Score Task Force of the International Common Disease A. Responsible use of polygenic risk scores in the clinic: potential benefits, risks and gaps. Nat Med. 2021;27:1876–84.
Article Google Scholar
Inouye M, Abraham G, Nelson CP, Wood AM, Sweeting MJ, Dudbridge F, et al. Genomic risk prediction of coronary artery disease in 480,000 adults: implications for primary prevention. J Am Coll Cardiol. 2018;72:1883–93.
Elliott J, Bodinier B, Bond TA, Chadeau-Hyam M, Evangelou E, Moons KGM, et al. Predictive accuracy of a polygenic risk score-enhanced prediction model vs a clinical risk score for coronary artery disease. JAMA. 2020;323:636–45.
Abraham G, Havulinna AS, Bhalala OG, Byars SG, De Livera AM, Yetukuri L, et al. Genomic prediction of coronary heart disease. Eur Heart J. 2016;37:3267–78.
Riveros-Mckay F, Weale ME, Moore R, Selzam S, Krapohl E, Sivley RM, et al. Integrated polygenic tool substantially enhances coronary artery disease prediction. Circ Genom Precis Med. 2021;14:e003304.
O’Sullivan JW, Raghavan S, Marquez-Luna C, Luzum JA, Damrauer SM, Ashley EA, et al. Polygenic risk scores for cardiovascular disease: a scientific statement from the American Heart Association. Circulation. 2022;146:e93–e118.
Kullo IJ, Jouni H, Austin EE, Brown SA, Kruisselbrink TM, Isseh IN, et al. Incorporating a genetic risk score into coronary heart disease risk estimates: effect on low-density lipoprotein cholesterol levels (the MI-GENES Clinical Trial). Circulation. 2016;133:1181–8.
Kuchenbaecker KB, McGuffog L, Barrowdale D, Lee A, Soucy P, Dennis J, et al. Evaluation of polygenic risk scores for breast and ovarian cancer risk prediction in BRCA1 and BRCA2 mutation carriers. J Natl Cancer Inst. 2017;109:djw302.
Mars N, Widen E, Kerminen S, Meretoja T, Pirinen M, Della Briotta Parolo P, et al. The role of polygenic risk and susceptibility genes in breast cancer over the course of life. Nat Commun. 2020;11:6383.
Huntley C, Torr B, Sud A, Rowlands CF, Way R, Snape K, et al. Utility of polygenic risk scores in UK cancer screening: a modelling analysis. Lancet Oncol. 2023;24:658–68.
Salazar de Pablo G, Radua J, Pereira J, Bonoldi I, Arienti V, Besana F, et al. Probability of transition to psychosis in individuals at clinical high risk: an updated meta-analysis. JAMA Psychiatry. 2021;78:970–8.
Koutsouleris N, Dwyer DB, Degenhardt F, Maj C, Urquijo-Castro MF, Sanfelici R, et al. Multimodal machine learning workflows for prediction of psychosis in patients with clinical high-risk syndromes and recent-onset depression. JAMA Psychiatry. 2021;78:195–209.
Perkins DO, Olde Loohuis L, Barbee J, Ford J, Jeffries CD, Addington J, et al. Polygenic risk score contribution to psychosis prediction in a target population of persons at clinical high risk. Am J Psychiatry. 2020;177:155–63.
Davies RW, Fiksinski AM, Breetvelt EJ, Williams NM, Hooper SR, Monfeuga T, et al. Using common genetic variation to examine phenotypic expression and risk prediction in 22q11.2 deletion syndrome. Nat Med. 2020;26:1912–8.
Torkamani A, Wineinger NE, Topol EJ. The personal and clinical utility of polygenic risk scores. Nat Rev Genet. 2018;19:581–90.
Pedersen CB, Mors O, Bertelsen A, Waltoft BL, Agerbo E, McGrath JJ, et al. A comprehensive nationwide study of the incidence rate and lifetime risk for treated mental disorders. JAMA Psychiatry. 2014;71:573–81.
Whitehouse AJO, Varcin KJ, Pillar S, Billingham W, Alvares GA, Barbaro J, et al. Effect of preemptive intervention on developmental outcomes among infants showing early signs of autism: a randomized clinical trial of outcomes to diagnosis. JAMA Pediatr. 2021;175:e213298.
Arango C, Diaz-Caneja CM, McGorry PD, Rapoport J, Sommer IE, Vorstman JA, et al. Preventive strategies for mental health. Lancet Psychiatry. 2018;5:591–604.
Riggs ER, Andersen EF, Cherry AM, Kantarci S, Kearney H, Patel A et al. Technical standards for the interpretation and reporting of constitutional copy-number variants: a joint consensus recommendation of the American College of Medical Genetics and Genomics (ACMG) and the Clinical Genome Resource (ClinGen). Genet Med. 2020;22:245–57.
Rammos A, Kirov G, Hubbard L, Walters JTR, Holmans P, Owen MJ, et al. Family-based analysis of the contribution of rare and common genetic variants to school performance in schizophrenia. Mol Psychiatry. 2023;28:2081–7.
van den Broek JJ, Schechter CB, van Ravesteyn NT, Janssens A, Wolfson MC, Trentham-Dietz A, et al. Personalizing breast cancer screening based on polygenic risk and family history. J Natl Cancer Inst. 2021;113:434–42.
Schaaf CP, Betancur C, Yuen RKC, Parr JR, Skuse DH, Gallagher L, et al. A framework for an evidence-based gene list relevant to autism spectrum disorder. Nat Rev Genet. 2020;21:367–76.
Douard E, Zeribi A, Schramm C, Tamer P, Loum MA, Nowak S, et al. Effect sizes of deletions and duplications on autism risk across the genome. Am J Psychiatry. 2021;178:87–98.
Download references
Acknowledgements
We thank Dr Robert W Davies at the Department of Statistics, University of Oxford, Oxford, UK, for writing the code underpinning Fig. 2b . This work was supported by the SickKids Psychiatry Associates Chair in Developmental (JV) and by NIH grants U01MH119741-01 (JV) and U01MH119746 (JS). These funding sources did not influence content of this manuscript.
Author information
Authors and affiliations.
Department of Psychiatry, The Hospital for Sick Children, Toronto, ON, Canada
Jacob Vorstman
Department of Psychiatry, Temerty Faculty of Medicine, University of Toronto, Toronto, ON, Canada
Program in Genetics and Genome Biology, Research Institute, The Hospital for Sick Children, Toronto, ON, Canada
Department of Psychiatry, Department of Cellular & Molecular Medicine, Beyster Center of Psychiatric Genomics, University of California San Diego, San Diego, CA, USA
Jonathan Sebat
Centre de Recherche du Centre Hospitalier Universitaire Sainte-Justine, Montréal, QC, Canada
Vincent-Raphaël Bourque & Sébastien Jacquemont
Department of Psychiatry, McGill University, Montréal, QC, Canada
Vincent-Raphaël Bourque
Département de Pédiatrie, Université de Montréal, Montréal, QC, Canada
Sébastien Jacquemont
You can also search for this author in PubMed Google Scholar
Contributions
JV, JS, VRB and SJ contributed to the conception of the topic of this expert review as well as to the writing of the different iterations of this work. JV, JS, VRB and SJ approved the final manuscript.
Corresponding author
Correspondence to Jacob Vorstman .
Ethics declarations
Competing interests.
JV has served as a consultant for Nobias Therapeutics Inc. and has received speaker fees for Henry Stewart Talks Ltd.
Additional information
Publisher’s note Springer Nature remains neutral with regard to jurisdictional claims in published maps and institutional affiliations.
Rights and permissions
Springer Nature or its licensor (e.g. a society or other partner) holds exclusive rights to this article under a publishing agreement with the author(s) or other rightsholder(s); author self-archiving of the accepted manuscript version of this article is solely governed by the terms of such publishing agreement and applicable law.
Reprints and permissions
About this article
Cite this article.
Vorstman, J., Sebat, J., Bourque, VR. et al. Integrative genetic analysis: cornerstone of precision psychiatry. Mol Psychiatry (2024). https://doi.org/10.1038/s41380-024-02706-2
Download citation
Received : 20 October 2023
Revised : 13 August 2024
Accepted : 19 August 2024
Published : 30 August 2024
DOI : https://doi.org/10.1038/s41380-024-02706-2
Share this article
Anyone you share the following link with will be able to read this content:
Sorry, a shareable link is not currently available for this article.
Provided by the Springer Nature SharedIt content-sharing initiative
Quick links
- Explore articles by subject
- Guide to authors
- Editorial policies


COMMENTS
The term "rare disease" describes a group of diseases whose prevalence is so low that they are considered an unviable market for therapeutics in the absence of appropriate incentives and support and too rare to be fully investigated and appropriately managed by professionals (Orphanet, 2020, Waxman, 1983).Among this group, there is a subgroup, the rare genetic diseases target of this ...
Genetics plays a role, to a greater or lesser extent, in all diseases. ... Essays Biochem. 2018 Dec 2;62(5):643-723. doi: 10.1042/EBC20170053. ... contribute to disease processes. This review explores the genetic basis of human disease, including single gene disorders, chromosomal imbalances, epigenetics, cancer and complex disorders, and ...
It has previously been estimated that around 42-48% of patients with a severe developmental disorder (DD) have a pathogenic de novo mutation (DNM) in a protein-coding gene 1, 2. However, most of ...
A primary goal of human genetics is to identify DNA sequence variants that influence biomedical traits, particularly those related to the onset and progression of human disease. Over the past 25 ...
The start of 2022 is an inflection point in the development of diagnostics and treatments for rare genetic diseases in prenatal, pediatric, and adult individuals-the theme of this special issue. Here I briefly review recent developments in two pivotal aspects of genetic disease diagnostics and treatments: education and equitable implementation.
Beyond disease prediction and biomarker discovery, biomarker-based predictions of individuals with disease could also augment case-control genetic discovery analyses.
the genetic basis of human disease, including single gene disorders, chromosomal imbal-. ances, epigenetics, cancer and complex disorders, and considers how our understanding. and technological ...
homogeneous. However, genomic efforts to understand the impact of mouse genetic diversity on phenotypic outcomes will help us to assess the impact of the genetic heterogeneity inherent to the study of human genetic diseases (Srivastava et al., 2017). Second, human mutations can be exceedingly rare, thus preventing a large casuistic for study.
Abstract. Autism spectrum disorder (autism) is a heterogeneous group of neurodevelopmental conditions characterized by early childhood-onset impairments in communication and social interaction alongside restricted and repetitive behaviors and interests. This review summarizes recent developments in human genetics research in autism ...
A mutation is a change in the nucleotide sequence of a. short region of a genome [1] ( Figure 1 ). Mutation, (a. term coined by Hugo de Yeries in 1900, a rediscover. of Mendels principle s) is ...
Recent genetic studies of the Plain community continue to highlight the value of studying isolated populations to propel genetic disease discovery and treatment. Additionally, population-specific polygenic risk scores are needed for underrepresented and minority populations, to avoid exacerbating di …
Explore the latest full-text research PDFs, articles, conference papers, preprints and more on GENETIC DISORDERS. Find methods information, sources, references or conduct a literature review on ...
New genes, pathways and therapeutic targets in autoinflammatory diseases. Studies in 2023 have described eight new monogenic autoinflammatory diseases and their accompanying disease-causing ...
Results . The United States contributed the highest number of publications, followed by China. Notably, the journal Blood emerged as the leading authority in β-thalassemia research. Analysis of research hotspots revealed that the pathogenesis of β-thalassemia is primarily linked to iron overload, anemia, gene mutations, and ineffective erythropoiesis.
Follow-up analyses of the attention and learning difficulties factor identified 49 genetic regions and 174 genes shared between dyslexia and ADHD, of which 40 regions and 121 genes have not been previously identified. This is the first time that genetic links to dyslexia have been studied in the context of psychiatric traits.
A high chance of genetic disorder is associated with the age of the father begins between 20 and 70 years. By analyzing the patient's age, the genetic disorder diseases occur within the 15 years. This analysis shows that age is an important factor that can be used to study genetic disorders.
Overview. A genetic disorder is a disease caused in whole or in part by a change in the DNA sequence away from the normal sequence. Genetic disorders can be caused by a mutation in one gene (monogenic disorder), by mutations in multiple genes (multifactorial inheritance disorder), by a combination of gene mutations and environmental factors, or ...
What to know. Genetic disorders are health problems that happen because of some type of abnormality in a person's genetic material. There are several types of genetic disorders. Some disorders are caused by a genetic change (mutation) in a single gene; some are caused by an abnormality in one of the chromosomes; and some are complex, involving ...
Autism spectrum disorders (ASDs) have been increasing in prevalence. ASD is a complex human genetic disorder with high heredity and involves interactions between genes and the environment. A ...
Abstract. Congenital defects are main causes of new born and child anomalies and morality, which cause single or multiple defects in one or many organs of the child. Worldwide each year about 3 % ...
Genetic testing is used to inform people whether they have a disorder, or if there is a chance they might pass a disorder on to their children. Approaches to identifying genetic disorders In the UK, if a person is concerned they might have, or might develop, a genetic disorder based on their family history, they can: Go to their GP, who might ...
DISSECTING THE GENETIC RISK OF MENTAL ILLNESS. The nature vs. nurture debate on the causes of mental illness is now understood to be a false dichotomy38, 39.Variation in risk of mental illness is neither solely due to variation in DNA or environmental factors, but both nature and nurture unequivocally contribute in closely intertwined processes.. For millennia, it has been observed that mental ...
An X-linked genetic disease is a disease inherited through a genetic defect on the X chromosome.In human cells, there is a pair of non-matching sex chromosomes, labelled X and Y. Females carry two X chromosomes, whereas males have one X and one Y chromosome.A disease or trait determined by a gene on the X chromosome demonstrates X-linked inheritance, which can be divided into dominant and ...
Human genetic studies of common variants have provided substantial insight into the biological mechanisms that govern ovarian ageing1. Here we report analyses of rare protein-coding variants in ...
Sept 10 (Reuters) - BridgeBio Pharma (BBIO.O), opens new tab said on Tuesday it will stop development of its experimental therapy for a genetic disease that affects the adrenaline-producing gland ...
Genetic disorders are of different types i.e. single-gene. disorders, chromosomal disorders, complex disorder s. This paper intends to be as an introductory paper for the project "Human genetic ...
The enormous successes in the genetics and genomics of many diseases have provided the basis for the advancement of precision medicine. Thus, the detection of genetic variants associated with neuropsychiatric disorders, as well as treatment outcome, has raised growing expectations that these findings could soon be translated into the clinic to improve diagnosis, the prediction of disease risk ...
The genetics of drug-related movement disorders, an umbrella review of meta-analyses. Mol Psychiatry. 2020;25:2237-50. Article PubMed Google Scholar
Purpose of Review Antisocial personality disorder (ASPD) is a characterized by lifelong or recurrent behavioral problems that begin in childhood or early adolescence. This communication provides an overview on ASPD including findings from recent reviews and new research. Recent Findings With regard to DSM-5's Section III Alternative Model of Personality Disorder criteria for ASPD, advocates ...
The role of genetic testing in the domain of neurodevelopmental and psychiatric disorders (NPDs) is gradually changing from providing etiological explanation for the presence of NPD phenotypes to ...