An official website of the United States government
Official websites use .gov A .gov website belongs to an official government organization in the United States.
Secure .gov websites use HTTPS A lock ( Lock Locked padlock icon ) or https:// means you've safely connected to the .gov website. Share sensitive information only on official, secure websites.
- Publications
- Account settings
- Advanced Search
- Journal List


Sources and Consequences of Groundwater Contamination
D karunanidhi, t subramani, k srinivasamoorthy.
- Author information
- Article notes
- Copyright and License information
Corresponding author.
Accepted 2020 Dec 17; Issue date 2021.
This article is made available via the PMC Open Access Subset for unrestricted research re-use and secondary analysis in any form or by any means with acknowledgement of the original source. These permissions are granted for the duration of the World Health Organization (WHO) declaration of COVID-19 as a global pandemic.
Groundwater contamination is a global problem that has a significant impact on human health and ecological services. Studies reported in this special issue focus on contaminants in groundwater of geogenic and anthropogenic origin distributed over a wide geographic range, with contributions from researchers studying groundwater contamination in India, China, Pakistan, Turkey, Ethiopia, and Nigeria. Thus, this special issue reports on the latest research conducted in the eastern hemisphere on the sources and scale of groundwater contamination and the consequences for human health and the environment, as well as technologies for removing selected contaminants from groundwater. In this article, the state of the science on groundwater contamination is reviewed, and the papers published in this special issue are summarized in terms of their contributions to the literature. Finally, some key issues for advancing research on groundwater contamination are proposed.
Groundwater is a major source of fresh water for the global population and is used for domestic, agricultural, and industrial uses. Approximately one third of the global population depends on groundwater for drinking water (International Association of Hydrogeologists 2020 ). Groundwater is a particularly important resource in arid and semi-arid regions where surface water and precipitation are limited (Li et al. 2017a ). Securing a safe and renewable supply of groundwater for drinking is one of the crucial drivers of sustainable development for a nation. However, urbanization, agricultural practices, industrial activities, and climate change all pose significant threats to groundwater quality. Contaminants, such as toxic metals, hydrocarbons, trace organic contaminants, pesticides, nanoparticles, microplastics, and other emerging contaminants, are a threat to human health, ecological services, and sustainable socioeconomic development (Li 2020 ; Li and Wu 2019 ).
Over the past three decades, chemical contamination is a common theme reported in groundwater studies. While groundwater contamination is a great challenge to human populations, this subject also presents a great opportunity for researchers to better understand how our subsurface aquifers have evolved and for decision makers to grasp how we can protect both the quality and quantity of these resources. Fresh water aquifers are one of the most important sections of the Critical Zone (CZ), which extends from the top of the vegetation canopy down to the bottom of the aquifer (Lin 2010 ). As part of the global effort to understand the functions, structures, and processes within the CZ, a range of investigations have been performed that contribute to our knowledge of the circulation and evolution of groundwater (Sawyer et al. 2016 ; Goldhaber et al. 2014 ).
Many of the contaminants in groundwater are of geogenic origin as a result of dissolution of the natural mineral deposits within the Earth’s crust (Basu et al. 2014 ; Pandey et al. 2016 ; Subba Rao et al. 2020 ; He et al. 2020a ). However, due to rapid expansion of the global population, urbanization, industrialization, agricultural production, and the economy, we now are faced with the challenge of the negative impacts of contaminants of anthropogenic origin. The countries most affected by these global changes are those that are going through rapid economic development, with many of them located in the eastern hemisphere (Clement and Meunie 2010 ; Hayashi et al. 2013 ; Lam et al. 2015 ). Thus, it is appropriate that this special issue entitled, “The fate and consequences of groundwater contamination” focuses on studies of the unique challenges related to contaminants of both anthropogenic and geogenic origin in groundwater in several countries in the eastern hemisphere, including China, India, Turkey, Bangladesh, Ethiopia, and Nigeria. Figure 1 illustrates the countries where the research was conducted and the classes of chemical contaminants reported in the articles in this special issue.
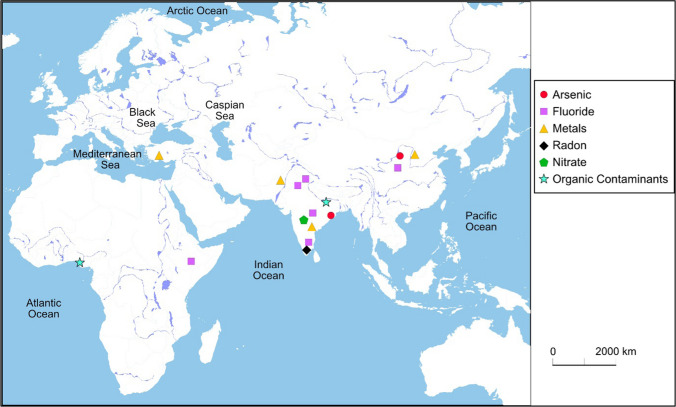
Eastern hemisphere, showing the countries where the groundwater research was conducted and the classes of contaminants studied in the articles published in this special issue
The range of topics included in articles in this special issue includes: (1) Latest methods for detecting and tracking the movement of groundwater contaminants; (2) Novel techniques for assessing risks to human populations consuming contaminated groundwater; (3) Effects of groundwater contamination on the abiotic environment, such as soil, sediments, and surface water; and (4) Case studies and remedial actions to control groundwater contamination from natural and anthropogenic sources. The co-editors of this special issue anticipate that these articles will facilitate an understanding of the origins and extent of groundwater contamination and its consequences and will provide examples of approaches that can be taken for remediation of groundwater contamination and protection of groundwater quality.
Major Contaminants
Groundwater contamination is defined as the addition of undesirable substances to groundwater caused by human activities (Government of Canada 2017 ). This can be caused by chemicals, road salt, bacteria, viruses, medications, fertilizers, and fuel. However, groundwater contamination differs from contamination of surface water in that it is invisible and recovery of the resource is difficult at the current level of technology (MacDonald and Kavanaugh 1994 ). Contaminants in groundwater are usually colorless and odorless. In addition, the negative impacts of contaminated groundwater on human health are chronic and are very difficult to detect (Chakraborti et al. 2015 ). Once contaminated, remediation is challenging and costly, because groundwater is located in subsurface geological strata and residence times are long (Wang et al. 2020 ; Su et al. 2020 ). The natural purification processes for contaminated groundwater can take decades or even hundreds of years, even if the source of contamination is cut off (Tatti et al. 2019 ).
The numbers of classes of contaminants detected in groundwater are increasing rapidly, but they can be broadly classified into three major types: chemical contaminants, biological contaminants, and radioactive contaminants. These contaminants can come from natural and anthropogenic sources (Elumalai et al. 2020 ). The natural sources of groundwater contamination include seawater, brackish water, surface waters with poor quality, and mineral deposits. These natural sources may become serious sources of contamination if human activities upset the natural environmental balance, such as depletion of aquifers leading to saltwater intrusion, acid mine drainage as a result of exploitation of mineral resources, and leaching of hazardous chemicals as a result of excessive irrigation (Su et al. 2020 ; Wu et al. 2015 ; Li et al. 2016 , 2018 ).
Nitrogen contaminants, such as nitrate, nitrite, and ammonia nitrogen, are prevalent inorganic contaminants. Nitrate is predominantly from anthropogenic sources, including agriculture (i.e., fertilizers, manure) and domestic wastewater (Hansen et al. 2017 ; He and Wu 2019 ; He et al. 2019 ; Karunanidhi et al. 2019 ; Li et al. 2019a ; Serio et al. 2018 ; Zhang et al. 2018 ). Groundwater nitrate contamination has been widely reported from regions all over the world. Other common inorganic contaminants found in groundwater include anions and oxyanions, such as F − , SO 4 2− , and Cl − , and major cations, such as Ca 2+ and Mg 2+ . Total dissolved solids (TDS), which refers to the total amount of inorganic and organic ligands in water, also may be elevated in groundwater. These contaminants are usually of natural origin, but human activities also can elevate levels in groundwater (Adimalla and Wu 2019 ).
Toxic metals and metalloids are a risk factor for the health of both human populations and for the natural environment. Chemical elements widely detected in groundwater include metals, such as zinc (Zn), lead (Pb), mercury (Hg), chromium (Cr), and cadmium (Cd), and metalloids, such as selenium (Se) and arsenic (As). Exposures at high concentrations can lead to severe poisoning, although some of these elements are essential micronutrients at lower doses (Hashim et al. 2011 ). For example, exposure to hexavalent chromium (Cr 6+ ) can increase the risk of cancer (He and Li 2020 ). Arsenic is ranked as a Group 1 human carcinogen by the US Environmental Protection Agency (EPA) and the International Agency for Research on Cancer (IARC), and As 3+ can react with sulfhydryl (–SH) groups of proteins and enzymes to upset cellular functions and eventually cause cell death (Abbas et al. 2018 ; Rebelo and Caldas 2016 ). Toxic metals in the environment are persistent and subject to moderate bioaccumulation when they enter the food chain (He and Li 2020 ; Hashim et al. 2011 ).
Organic contaminants have been widely detected in drinking water, and many of these compounds are regarded as human carcinogens or endocrine disrupting chemicals. In groundwater, more than 200 organic contaminants have been detected, and this number is still increasing (Lesser et al. 2018 ; Jurado et al. 2012 ; Lapworth et al. 2012 ; Sorensen et al. 2015 ). Some organic contaminants are biodegradable, while some are persistent. The biodegradable organic contaminants originate mainly from domestic sewage and industrial wastewater. Many of these organic substances are naturally produced from carbohydrates, proteins, fats, and oils and can be transformed into stable inorganic substances by microorganisms. They have no direct toxic effects on living beings but can reduce the dissolved oxygen levels in groundwater. Common organic contaminants include hydrocarbons, halogenated compounds, plasticizers, pesticides, pharmaceuticals, and personal care products and natural estrogens, among others (Lapworth et al. 2015 ; Meffe and Bustamante 2014 ). Many of the halogenated compounds (e.g., chlorinated, brominated, fluorinated) are stable in the environment and can be accumulated and enriched in organisms, causing harmful effects in organisms from higher trophic levels, including humans (Gwenzi and Chaukura 2018 ; Schulze et al. 2019 ). The persistent organic contaminants are mainly compounds used for agriculture, industrial processes, and protection of human health (Lapworth et al. 2015 ). Because these compounds degrade very slowly or even not at all, they may permanently threaten the quality of groundwater for drinking purposes (Schulze et al. 2019 ).
Radioactive contaminants in groundwater can originate from geological deposits of radionuclides but also can originate from anthropogenic sources, such as wastes from nuclear power plants, nuclear weapons testing, and improper disposal of medical radioisotopes (Dahlgaard et al. 2004 ; Lytle et al. 2014 ; Huang et al. 2012 ). Radioactive substances can enter the human body through a variety of routes, including drinking water. However, radioactive contaminants have been rarely detected in groundwater at levels that are a threat to human health.
Biological contaminants include algae and microbial organisms, such as bacteria, viruses, and protozoa. For microbial contaminants, more than 400 kinds of bacteria have been identified in human and animal feces, and more than 100 kinds of viruses have been recognized (Shen and Gao 1995 ). Some of these microbial organisms originate from natural sources, but some include microscopic organisms that co-exist with natural algal species and compete for available resources (Flemming and Wuertz 2019 ; Lam et al. 2018 ). Drinking water contaminated by microbial contaminants can result in many human diseases, including serious diarrheal diseases, such as typhoid and cholera. Currently, the COVID-19 virus has resulted in pandemic affecting every corner of the world. This coronavirus is primarily transmitted from person-to-person through respiratory droplets (Centers for Disease Control and Prevention 2020 ). However, water contaminated by this virus also can threaten human health (Bhowmick et al. 2020 ; Lokhandwala and Gautam 2020 ). Algal contamination is very common in surface waters, such as lakes and reservoirs due to eutrophication, but algae are rarely found at a high biomass in groundwater.
Consequences of Groundwater Contamination
Groundwater contamination can impact human health, environmental quality, and socioeconomic development. For example, many studies have shown that high levels of fluoride, nitrate, metals, and persistent organic pollutants are a health risk for human populations (Wu et al. 2020 ). This is especially critical for infants and children who are more susceptible to the effects of these contaminants than adults (He et al. 2020b ; Wu and Sun 2016 ; Karunanidhi et al. 2020 ; Mthembu et al. 2020 ; Ji et al. 2020 ; Subba Rao et al. 2020 ; Zhou et al. 2020 ). For example, “blue baby syndrome,” also known as infant methemoglobinemia, is caused by excessive nitrate concentrations in the drinking water used to make baby formulas. Human health also can be affected by the groundwater contamination through effects on the food production system. Irrigation with groundwater contaminated by heavy metals and wastewater containing persistent contaminants can result in the accumulation of toxic elements in cereals and vegetables, causing health risks to humans (Jenifer and Jha 2018 ; Yuan et al. 2019 ; Njuguna et al. 2019 ).
Groundwater contamination also can negatively affect the quality of lands and forests. Contaminated groundwater can lead to soil contamination and degradation of land quality. For example, in many agricultural areas in arid regions, high groundwater salinity is one of the major factors influencing soil salinization (Wu et al. 2014 ). The soluble salts and other contaminants, such as toxic metals, can accumulate in the root zone, affecting vegetation growth. Groundwater contaminants also can be transported by surface water-groundwater interactions, leading to deterioration of surface water quality (Teng et al. 2018 ).
Sustainable economic development requires a balance between the rate of renewal of natural resources and human demand (Li et al. 2017b ). Freshwater is probably the most valuable of the natural resources. However, chronic groundwater contamination may reduce the availability of freshwater, breaking the balance between water supply and demand and leading to socioeconomic crises and even wars. Water shortages induced by contamination may become a factor causing conflicts among citizens in the future (Schillinger et al. 2020 ), possibly delaying the socioeconomic development of a nation. Groundwater contamination is not only an environmental issue but also a social issue, demanding collaboration between both natural scientists and social scientists.
Articles in the Special Issue
Nineteen papers are included in this special issue. The topics of these papers cover a range of contamination issues, including the sources of geogenic and anthropogenic contamination, seasonal cycles in contamination, human health risks, and remediation technologies. Figure 2 illustrates a word cloud generated using the words in the titles and abstracts of the articles in this special issue, showing the most frequently used terms. The word cloud shows that the most frequently used technical terms in the articles are water, risk, metals, nitrate, fluoride, polycyclic aromatic hydrocarbons (PAHs), health, limits, and values. These terms reflect the main topics of the articles, which cover the assessment of the concentrations of trace metals, fluoride, nitrate, PAHs, and other organic contaminants in groundwater and the associated risks to the health of human populations. Some more minor terms, such as geogenic, source, removal, statistical, EWQI, and mobility, indicate that some articles focus on evaluating the sources of groundwater contamination, approaches to groundwater quality assessment, and contaminant remediation techniques. The main contributions of each article in this special issue are summarized below.
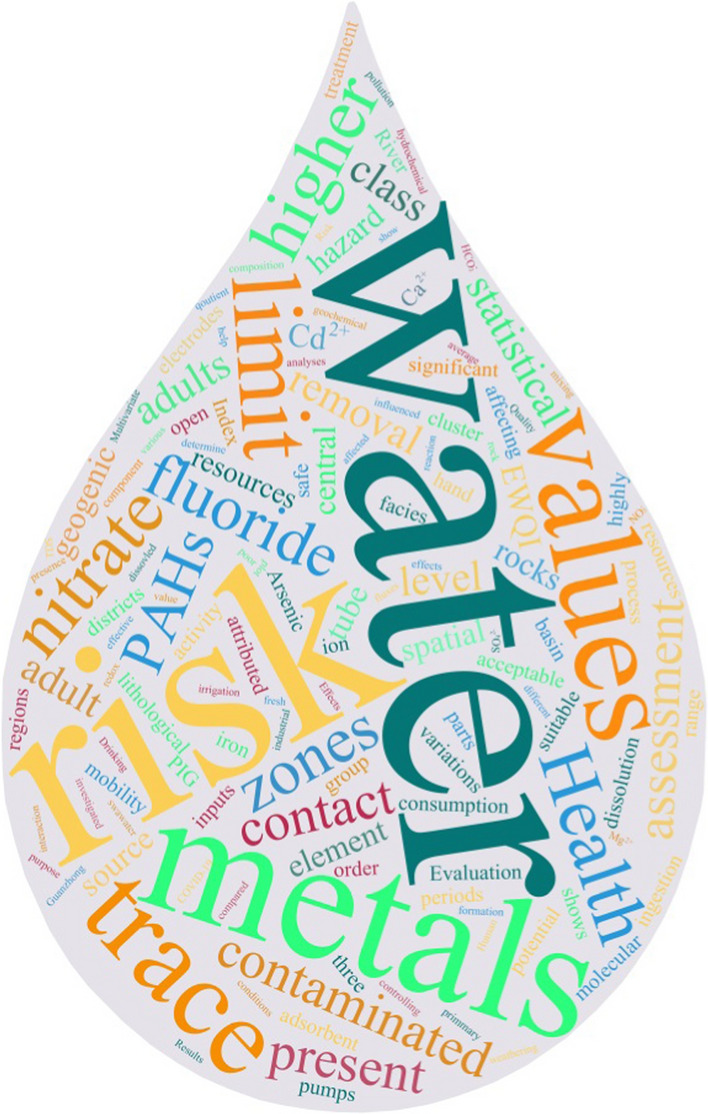
Word cloud generated using the words in the titles and abstracts of articles in this special issue
Toxic metals are persistent contaminants and can be bioaccumulated in human tissues via food chain (He and Li 2020 ). In this special issue, six articles focused on the assessing trace metal pollution in groundwater. Çiner et al. ( 2021 ) used multivariate statistical analysis to identify the sources of trace elements in groundwater, including Al, Cr, Mn, Fe, Co, Ni, Cu, Zn, As, Se, and Ba, and assessed the health risks from arsenic contamination in a region of south-central Turkey. Their research results indicate that the carcinogenic risks from exposure to arsenic to both adults and children were higher than the guideline limit, and the geogenic processes are the main cause of trace element contamination in groundwater in this region. Chandrasekar et al. ( 2021 ) also identified geogenic metal contamination in their article focused on the source, geochemical mobility, and health risks from trace metals in groundwater in a Cretaceous-Tertiary (K/T) contact region of India. However, Raja et al. ( 2021 ) concluded that industrial activities and leaching from municipal dumpsites were the main sources of the metal pollution in the groundwater in the industrialized township (Taluk) of Virudhunagar in India.
In addition to contamination of groundwater, trace elements can be transported via groundwater into surface waters and into oceans. In the article by Prakash et al. ( 2021 ), estimates were made of the submarine groundwater discharge and associated trace element fluxes from an urban estuary region to the marine environment in the Bay of Bengal in India. This study revealed that submarine groundwater discharge is an important factor contributing to the fluxes to the sea of dissolved trace elements.
Finding efficient and cost-effective technologies for removal of trace elements from groundwater is crucial for the sustainable management of water resources. Zhao et al. ( 2021 ) studied Cd removal from water using a novel low-temperature roasting technique associated with alkali to synthesize a high-performance adsorbent from coal fly ash. Dutta et al. ( 2021 ) proposed to use electrocoagulation with iron electrodes as a treatment technology for arsenic removal from groundwater, and a pilot scale filtration unit was used to remove ferric hydroxide flocs produced during the process.
Fluoride is of value in trace amounts for promoting dental health, but this anion is toxic when present in high concentrations in water and food (Adimalla and Li 2019 ; Li et al. 2014 , 2019b ; Marghade et al. 2020 ). In this special issue, two articles specifically address fluoride occurrence, distribution, and health risks. The article by Haji et al. ( 2021 ) describes a study of groundwater quality and human health risks from fluoride contamination in a region within the southern Main Ethiopian Rift. Keesari et al. ( 2021 ) used the empirical cumulative density function to estimate the health risks from consuming fluoride contaminated groundwater in northeastern parts of Rajasthan in India. These authors also produced a fluorosis risk map to aid decision makers in taking necessary remedial measures to improve the groundwater quality.
Organic pollutants, including polycyclic aromatic hydrocarbons (PAHs) and polychlorinated biphenyls (PCBs), are common contaminants of anthropogenic origin in groundwater that could cause serious health problems. In this special issue, two articles focused on these organic pollutants. The article by Ololade et al. ( 2021 ) reported an investigation into PAHs and PCBs in groundwater near selected waste dumpsites located in two southwestern states in Nigeria. They found that the more water-soluble, low molecular weight-PAHs accounted for more than 61% of the total PAHs detected across all locations, but surprisingly the more highly chlorinated hexa-PCBs dominated the congener profiles. In another paper in this issue by Ambade et al. ( 2021 ), the occurrence, distribution, health risk, and composition of 16 priority PAHs were investigated in drinking water from southern Jharkhand in the eastern part of India. These authors found that lower and middle molecular weight PAHs were dominant in groundwater from the study area, but the levels are currently below concentrations that are a carcinogenic risk.
Studies of radioactive elements in groundwater often are neglected, but these radionuclides can be a hazard to human health. Adithya et al. ( 2021 ) conducted a study in Tamil Nadu state in southern India to measure the levels of radon (Rn) in groundwater and quantify the health risks. Their study showed that the Rn is released into groundwater from granitic and gneissic rocks within uranium-enriched lithological zones. However, the Rn levels determined in Bequerels per litre were lower than the guideline limit and the groundwater does not pose health risks to consumers.
In this special issue, Adimalla and Qian ( 2021 ) conducted a study on the spatial distribution and potential health risks from nitrate pollution in groundwater in southern India. The article revealed high nitrate levels in groundwater, at concentrations up to 130 mg/L. Both adults and children were judged to face health risks from consumption of nitrate in drinking water, but children were identified as more susceptible to the effects of groundwater nitrate pollution. The paper by Karunanidhi et al. ( 2021 ) describes the improvements in groundwater quality that occurred in an industrialized region of southeastern India between January and June of 2020. These improvements included reduced nitrate contamination, which may have been due to reduced transport of nitrate into groundwater before the monsoon period, but also could have been due to the decline in industrial and agricultural activity in the region during the lockdown in India that began in March 2020 in response to the first wave of the COVID-19 pandemic. In this study, fluoride concentrations of geogenic origin also were lower in groundwater before the monsoon.
Understanding the seasonal and spatial variations in groundwater quality is essential for the protection of human health and to maintain the crop yields. Subba Rao et al. ( 2021 ) used multiple approaches to identify the seasonal variations in groundwater quality and revealed that the groundwater quality for drinking and irrigation purposes was lower in the post-monsoon period relative to the pre-monsoon period. The deterioration of groundwater quality in the post-monsoon period was attributed to contaminant transport occurring through groundwater recharge but also was influenced by topographical factors and human activities.
Understanding the hydrogeochemical processes affecting groundwater chemistry is the basis for effective management of groundwater resources. Ren et al. ( 2021 ) adopted statistical approaches and multivariate statistical analysis techniques to understand the hydrogeochemical processes affecting groundwater in the central part of the Guanzhong Basin, China. The main contribution of this article is that it could help local decision makers to make water management decisions in the densely populated river basin by providing them with useful groundwater management options.
There are four articles in this special issue that focus specifically on methods to assess groundwater quality and humfluoride and associated arsenicosis and fluoan health risks. Shukla and Saxena ( 2021 ) assessed the groundwater quality and health risk in the rural parts of Raebareli district in northern India. Wang et al. ( 2021 ) identified the hydrochemical characteristics of groundwater and assessed health risk to consumers in a part of the Ordos basin in China. Adimalla ( 2021 ) applied two indices: the entropy weighted water quality index (EWQI), and the pollution index of groundwater (PIG) to assess the suitability of groundwater for drinking purpose in the Telangana state in southeastern India. Khan et al. ( 2021 ) assessed the drinking water quality and potential health impacts by considering physicochemical parameters, as well as bacteriological contamination of groundwater in Bajaur, Pakistan.
Collectively, these articles contribute to the literature on scientific developments in the field of groundwater contamination. The case studies presented in these articles are useful for policy makers and the public to understand the current water quality status in these regions. In particular, these articles provide a window into the groundwater contamination issues that are affecting low- and middle-income countries and countries with emerging economies in the eastern hemisphere. Researchers from Europe, North America, and other high-income countries often do not grasp the extent of groundwater contamination from geogenic and anthropogenic sources in these regions and do not realize that many human populations have no choice but to consume the contaminated drinking water.
The Way Ahead
Groundwater contamination is now a global problem and the resolution of these problems requires close collaboration among researchers in universities and government agencies, industries, and decision makers from all levels of government. To solve the groundwater contamination problems, international collaboration is needed. This is particularly true in countries with developing economies where financial resources and access to advanced technologies are not readily available. Special focus should be given to the following aspects of research and training:
Groundwater contamination issues in different countries should be addressed with a range of measures, techniques, and policies. Although groundwater contamination is a global problem, its nature and influencing factors are different between countries, climatic regions, and geological features. It may not be optimal to adopt remediation approaches that are successful in other countries or regions. For example, nitrate pollution is caused by fertilizer and manure applications in some agricultural regions (Zhang et al. 2018 ) but also may be caused by pollution by industrial and domestic wastewater in other areas, or even by explosives used in mineral exploration (Li et al. 2018 ). It may be necessary to use different approaches to mitigate different types of nitrate pollution. Even in instances where fertilizer application is the common cause of nitrate pollution in a tropical and a temperate region, the remediation approaches could be different, as climate factors and soil characteristics will have a great influence on the mechanisms and extent of contaminant transport.
With the rapid technological development, many novel techniques have been developed to study groundwater contamination, including geophysical and geoinformatics techniques. Geographical information systems (GIS) and remote sensing (Ahmed et al. 2020 ; Al-Abadi et al. 2020 ; Alshayef et al. 2019 ; Kannan et al. 2019 ) have accelerated the development of groundwater science. In the future, artificial intelligence, “big data” analysis, drone surveys, and molecular and stable isotope analysis technologies will be more widely available for applications in groundwater research. Groundwater scientists need to adopt and apply these new technologies for the study of groundwater contamination.
Governments, particularly in countries with developing economies need to invest in and encourage research and training in groundwater science. In many regions, human populations have no alternative but to consume groundwater that is contaminated with chemical or biological agents, potentially causing wide ranging health effects. Investment is needed to determine the extent of this contamination and how to remediate the impacts on human health, or to find alternate sources of drinking water.
Acknowledgements
Editing a successful special issue is not easy. The Guest Editors must ensure that the topic is of importance and of broad interest so that there are an adequate number of contributors willing to submit their manuscripts. They must also make sure that the peer review process is efficient and effective, while maintaining the high quality of the papers. All of these cannot be fulfilled without the support of the Editor in Chief. So, we are extremely grateful for Prof. Chris Metcalfe’s guidance and support for this special issue. We are also sincerely thankful to the reviewers who provided constructive comments that are essential for maintaining the high quality of the special issue. Last but not the least, the authors whose manuscripts were included and those whose manuscripts were rejected are acknowledged for their interest in contributing to the special issue. The special issue was edited in a situation in which the COVID-19 struck in nearly every corner of the world. We are impressed by the dedication of doctors who fought and/or are fighting against the coronavirus. Prof. Peiyue Li is grateful for the financial support granted by the National Natural Science Foundation of China (41761144059 and 42072286), the Fundamental Research Funds for the Central Universities of CHD (300102299301), the Fok Ying Tong Education Foundation (161098), and the Ten Thousand Talents Program (W03070125), which allow him to carry out various investigations. The year 2021 is the 70th anniversary of Chang’an University. Congratulations!
Compliance with Ethical Standards
Conflict of interest.
The authors declare no conflict of interest.
- Abbas G, Murtaza B, Bibi I, Shahid M, Niazi NK, Khan MI, Amjad M, Hussain M, Natasha Arsenic uptake, toxicity, detoxification, and speciation in plants: physiological, biochemical, and molecular aspects. Int J Environ Res Public Health. 2018;15:59. doi: 10.3390/ijerph15010059. [ DOI ] [ PMC free article ] [ PubMed ] [ Google Scholar ]
- Adimalla N (2021) Application of entropy weighted water quality index (EWQI) and pollution index of groundwater (PIG) to assess the groundwater quality for drinking purposes: a case study. Arch Environ Contam Toxicol ( this issue ) [ DOI ] [ PubMed ]
- Adimalla N, Li P. Occurrence, health risks and geochemical mechanisms of fluoride and nitrate in groundwater of the rock-dominant semi-arid region, Telangana State, India. Hum Ecol Risk Assess. 2019;25:81–103. [ Google Scholar ]
- Adimalla N, Qian H (2021) Geospatial distribution and potential noncarcinogenic health risk assessment of nitrate contaminated groundwater in Southern India: a case study. Arch Environ Contam Toxicol ( this issue ). 10.1007/s00244-020-00762-7 [ DOI ] [ PubMed ]
- Adimalla N, Wu J. Groundwater quality and associated health risks in a semi-arid region of south India: implication to sustainable groundwater management. Hum Ecol Risk Assess. 2019;25:191–216. [ Google Scholar ]
- Adithya VS, Chidambaram S, Prasanna MV, Venkatramanan S, Tirumalesh K, Thivya C, Thilagavathi R (2021) Health risk implication and spatial distribution of radon in groundwater along the lithological contact in south India. Arch Environ Contam Toxicol ( this issue ) [ DOI ] [ PubMed ]
- Ahmed A, Nawaz R, Woulds C, Drake F. Influence of hydro-climatic factors on future coastal land susceptibility to erosion in Bangladesh: a geospatial modelling approach. J Geovis Spat Anal. 2020;4:6. doi: 10.1007/s41651-020-00050-x. [ DOI ] [ Google Scholar ]
- Al-Abadi AM, Ghalib HB, Al-Mohammdawi JA. Delineation of groundwater recharge zones in Ali Al-Gharbi District, Southern Iraq using multi-criteria decision-making model and GIS. J Geovis Spat Anal. 2020;4:9. doi: 10.1007/s41651-020-00054-7. [ DOI ] [ Google Scholar ]
- Alshayef MS, Javed A, Mohammed AMB. Appraisal of potential hydrocarbon zones in Masila Oil Field, Yemen. J Geovis Spat Anal. 2019;3:17. doi: 10.1007/s41651-019-0043-0. [ DOI ] [ Google Scholar ]
- Ambade B, Sethi SS, Kumar A, Sankar TK (2021) Health risk assessment, composition and distribution of polycyclic aromatic hydrocarbons (PAHs) in drinking water of southern Jharkhand, East India. Arch Environ Contam Toxicol ( this issue ) [ DOI ] [ PubMed ]
- Basu A, Saha D, Saha R, Ghosh T, Saha B. A review on sources, toxicity, and remediation technologies for removing arsenic from drinking water. Res Chem Intermediation. 2014;40:447–485. [ Google Scholar ]
- Bhowmick GD, Dhar D, Nath D, Ghangrekar MM, Banerjee R, Das S, Chatterjee J. Coronavirus disease 2019 (COVID-19) outbreak: some serious consequences with urban and rural water cycle. NPJ Clean Water. 2020;3:32. doi: 10.1038/s41545-020-0079-1. [ DOI ] [ Google Scholar ]
- Centers for Disease Control and Prevention (2020) Global COVID-19. https://www.cdc.gov/coronavirus/2019-ncov/global-covid-19/index.html . Accessed 18 Oct 2020
- Chakraborti D, Rahman MM, Mukherjee A, Alauddin M, Hassan M, Dutta RN, Pati S, Mukherjee SC, Roy S, Quamruzzman Q, Rahman M, Islam T, Sorif S, Selim Md, Islam MR, Hossain MM. Groundwater arsenic contamination in Bangladesh—21 years of research. J Trace Elem Med Biol. 2015;31:237–248. doi: 10.1016/j.jtemb.2015.01.003. [ DOI ] [ PubMed ] [ Google Scholar ]
- Chandrasekar T, Keesari T, Gopalakrishnan G, Karuppannan S, Senapathi V, Sabarathinam C, Viswanathan PM (2021) Occurrence of heavy metals in groundwater along the lithological interface of K/T boundary, peninsular India: a special focus on source, geochemical mobility and health risk. Arch Environ Contam Toxicol ( this issue ) [ DOI ] [ PubMed ]
- Çiner F, Sunkari ED, Şenbaş BA (2021) Geochemical and multivariate statistical evaluation of trace elements in groundwater of Niğde Municipality, South-Central Turkey: implications for arsenic contamination and human health risks assessment. Arch Environ Contam Toxicol ( this issue ). 10.1007/s00244-020-00759-2 [ DOI ] [ PubMed ]
- Clement M, Meunie A. Is inequality harmful for the environment? An empirical analysis applied to developing and transition countries. Rev Social Econ. 2010;68:223–232. doi: 10.1080/00346760903480590. [ DOI ] [ PubMed ] [ Google Scholar ]
- Dahlgaard H, Eriksson M, Nielsen SP, Joensen HP. Levels and trends of radioactive contaminants in the Greenland environment. Sci Total Environ. 2004;331:53–67. doi: 10.1016/j.scitotenv.2004.03.023. [ DOI ] [ PubMed ] [ Google Scholar ]
- Dutta N, Haldar A, Gupta A (2021) Electrocoagulation for arsenic removal: field trials in West Bengal, India. Arch Environ Contam Toxicol ( this issue ) [ DOI ] [ PubMed ]
- Elumalai V, Nethononda VG, Manivannan V, Rajmohan N, Li P, Elango L. Groundwater quality assessment and application of multivariate statistical analysis in Luvuvhu catchment, Limpopo, South Africa. J Afr Earth Sci. 2020;171:103967. doi: 10.1016/j.jafrearsci.2020.103967. [ DOI ] [ Google Scholar ]
- Flemming H, Wuertz S. Bacteria and archaea on Earth and their abundance in biofilms. Nat Rev Microbiol. 2019;17:247–260. doi: 10.1038/s41579-019-0158-9. [ DOI ] [ PubMed ] [ Google Scholar ]
- Goldhaber MB, Mills CT, Morrison JM, Stricker CA, Mushet DM, LaBaugh JW. Hydrogeochemistry of prairie pothole region wetlands: role of long-term critical zone processes. Chem Geol. 2014;387:170–183. doi: 10.1016/j.chemgeo.2014.08.023. [ DOI ] [ Google Scholar ]
- Government of Canada (2017) Groundwater contamination. https://www.canada.ca/en/environment-climate-change/services/water-overview/pollution-causes-effects/groundwater-contamination.html . Accessed 17 Oct 2020
- Gwenzi W, Chaukura N. Organic contaminants in African aquatic systems: current knowledge, health risks, and future research directions. Sci Total Environ. 2018;619–620:1493–1514. doi: 10.1016/j.scitotenv.2017.11.121. [ DOI ] [ PubMed ] [ Google Scholar ]
- Haji M, Karuppannan S, Qin D, Shube H, Kawo NS (2021) Potential human health risks due to fluoride contaminants of groundwater using multi-techniques approaches in Bilate River Basin of Southern Main Ethiopian Rift, Ethiopia. Arch Environ Contam Toxicol ( this issue ) [ DOI ] [ PubMed ]
- Hansen B, Thorling L, Schullehner J, Termansen M, Dalgaard T. Groundwater nitrate response to sustainable nitrogen management. Sci Rep. 2017;7:8566. doi: 10.1038/s41598-017-07147-2. [ DOI ] [ PMC free article ] [ PubMed ] [ Google Scholar ]
- Hashim MA, Mukhopadhyay S, Sahu JN, Sengupta B. Remediation technologies for heavy metal contaminated groundwater. J Environ Manag. 2011;92:2355–2388. doi: 10.1016/j.jenvman.2011.06.009. [ DOI ] [ PubMed ] [ Google Scholar ]
- Hayashi A, Akimoto K, Tomoda T, Kii M. Global evaluation of the effects of agriculture and water management adaptations on the water-stressed population. Mitig Adapt Strateg Global Change. 2013;18:591–618. [ Google Scholar ]
- He X, Li P. Surface water pollution in the middle Chinese Loess Plateau with special focus on hexavalent chromium (Cr6+): occurrence, sources, and health risks. Expo Health. 2020;12:385–401. [ Google Scholar ]
- He S, Wu J. Hydrogeochemical characteristics, groundwater quality and health risks from hexavalent chromium and nitrate in groundwater of Huanhe Formation in Wuqi County, northwest China. Expo Health. 2019;11:125–137. [ Google Scholar ]
- He X, Wu J, He S. Hydrochemical characteristics and quality evaluation of groundwater in terms of health risks in Luohe aquifer in Wuqi County of the Chinese Loess Plateau, northwest China. Hum Ecol Risk Assess. 2019;25:32–51. [ Google Scholar ]
- He X, Li P, Wu J, Wei M, Ren X, Wang D (2020a) Poor groundwater quality and high potential health risks in the Datong Basin, northern China: research from published data. Environ Geochem Health. 10.1007/s10653-020-00520-7 [ DOI ] [ PubMed ]
- He X, Li P, Ji Y, Wang Y, Su Z, Elumalai V. Groundwater arsenic and fluoride and associated arsenicosis and fluorosis in China: occurrence, distribution, and management. Expo Health. 2020;12:355–368. doi: 10.1007/s12403-020-00347-8. [ DOI ] [ Google Scholar ]
- Huang C, Lin T, Chiao L, Chen H. Characterization of radioactive contaminants and water treatment trials for the Taiwan Research Reactor’s spent fuel pool. J Hazard Mater. 2012;233–234:140–147. doi: 10.1016/j.jhazmat.2012.07.009. [ DOI ] [ PubMed ] [ Google Scholar ]
- International Association of Hydrogeologists (2020) Groundwater—more about the hidden resource. https://iah.org/education/general-public/groundwater-hidden-resource . Accessed 13 Nov 2020
- Jenifer MA, Jha MK. Comprehensive risk assessment of groundwater contamination in a weathered hard-rock aquifer system of India. J Clean Product. 2018;201:853–868. [ Google Scholar ]
- Ji Y, Wu J, Wang Y, Elumalai V, Subramani T. Seasonal variation of drinking water quality and human health risk assessment in Hancheng City of Guanzhong Plain, China. Expo Health. 2020;12:469–485. [ Google Scholar ]
- Jurado A, Vàzquez-Suñé E, Carrera J, de Alda ML, Pujades E, Barceló D (2012) Emerging organic contaminants in groundwater in Spain: a review of sources, recent occurrence and fate in a European context. Sci Total Environ 440:82–94 [ DOI ] [ PubMed ]
- Kannan R, Venkateswaran S, Vijay Prabhu M, Suresh R, Kalaiyarasi A. Demarcation of groundwater possible zones in a hard rock terrain, Nagavathi watershed of Dharmapuri District, Tamil Nadu, India—Geophysical and geoinformatics approach. J Geovis Spat Anal. 2019;3:2. doi: 10.1007/s41651-018-0025-7. [ DOI ] [ Google Scholar ]
- Karunanidhi D, Aravinthasamy P, Subramani T, Wu J, Srinivasamoorthy K. Potential health risk assessment for fluoride and nitrate contamination in hard rock aquifers of Shanmuganadhi River basin, South India. Hum Ecol Risk Assess. 2019;25:250–270. [ Google Scholar ]
- Karunanidhi D, Aravinthasamy P, Deepali M, Subramani T, Bellows BC, Li P. Groundwater quality evolution based on geochemical modeling and aptness testing for ingestion using entropy water quality and total hazard indexes in an urban-industrial area (Tiruppur) of Southern India. Environ Sci Pollut Res. 2020 doi: 10.1007/s11356-020-10724-0. [ DOI ] [ PubMed ] [ Google Scholar ]
- Karunanidhi D, Aravinthasamy P, Deepali M, Subramani T, Shankar K, Kumar M (2021) Groundwater pollution and human health risks in an industrialized region of southern India: impacts of the COVID-19 lockdown and the monsoon seasonal cycles. Arch Environ Contam Toxicol ( this issue ) [ DOI ] [ PMC free article ] [ PubMed ]
- Keesari T, Pant D, Roy A, Sinha UK, Jaryal A, Singh M, Jain SK (2021) Fluoride geochemistry and exposure risk through groundwater sources in northeastern parts of Rajasthan, India. Arch Environ Contam Toxicol ( this issue ) [ DOI ] [ PubMed ]
- Khan MH, Nafees M, Muhammad N, Ullah U, Hussain R, Bilal M (2021) Assessment of drinking water sources for its quality, human health risks and pollution source(s) apportionment-a case study. Arch Environ Contam Toxicol ( this issue ) [ DOI ] [ PubMed ]
- Lam S, Nguyen-Viet H, Tuyet-Hanh TT, Nguyen-Mai H, Harper S. Evidence for public health risks of wastewater and excreta management practices in Southeast Asia: a scoping review. Int J Res Public Health. 2015;12:12855–12863. doi: 10.3390/ijerph121012863. [ DOI ] [ PMC free article ] [ PubMed ] [ Google Scholar ]
- Lam TP, Lee T-M, Chen C-Y, Chang J-S. Strategies to control biological contaminants during microalgal cultivation in open ponds. Bioresour Technol. 2018;252:180–187. doi: 10.1016/j.biortech.2017.12.088. [ DOI ] [ PubMed ] [ Google Scholar ]
- Lapworth DJ, Baranm N, Stuartm ME, Ward RS. Emerging organic contaminants in groundwater: a review of sources, fate and occurrence. Environ Pollut. 2012;163:287–303. doi: 10.1016/j.envpol.2011.12.034. [ DOI ] [ PubMed ] [ Google Scholar ]
- Lapworth DJ, Baran N, Stuart ME, Manamsa K, Talbot J. Persistent and emerging micro-organic contaminants in chalk groundwater of England and France. Environ Pollut. 2015;203:214–225. doi: 10.1016/j.envpol.2015.02.030. [ DOI ] [ PubMed ] [ Google Scholar ]
- Lesser LE, Mora A, Moreau C, Mahlknecht J, Hernández-Antonio A, Ramírez AI, Barrios-Piña H. Survey of 218 organic contaminants in groundwater derived from the world’s largest untreated wastewater irrigation system: Mezquital Valley, Mexico. Chemosphere. 2018;198:510–521. doi: 10.1016/j.chemosphere.2018.01.154. [ DOI ] [ PubMed ] [ Google Scholar ]
- Li P. To make the water safer. Expo Health. 2020;12:337–342. doi: 10.1007/s12403-020-00370-9. [ DOI ] [ PMC free article ] [ PubMed ] [ Google Scholar ]
- Li P, Wu J. Sustainable living with risks: meeting the challenges. Hum Ecol Risk Assess. 2019;25:1–10. [ Google Scholar ]
- Li P, Qian H, Wu J, Chen J, Zhang Y, Zhang H. Occurrence and hydrogeochemistry of fluoride in shallow alluvial aquifer of Weihe River, China. Environ Earth Sci. 2014;71:3133–3145. [ Google Scholar ]
- Li P, Wu J, Qian H. Preliminary assessment of hydraulic connectivity between river water and shallow groundwater and estimation of their transfer rate during dry season in the Shidi River, China. Environ Earth Sci. 2016;75:99. doi: 10.1007/s12665-015-4949-7. [ DOI ] [ Google Scholar ]
- Li P, Tian R, Xue C, Wu J. Progress, opportunities and key fields for groundwater quality research under the impacts of human activities in China with a special focus on western China. Environ Sci Pollut Res. 2017;24:13224–13234. doi: 10.1007/s11356-017-8753-7. [ DOI ] [ PubMed ] [ Google Scholar ]
- Li P, Qian H, Zhou W. Finding harmony between the environment and humanity: an introduction to the thematic issue of the Silk Road. Environ Earth Sci. 2017;76:105. doi: 10.1007/s12665-017-6428-9. [ DOI ] [ Google Scholar ]
- Li P, Wu J, Tian R, He S, He X, Xue C, Zhang K. Geochemistry, hydraulic connectivity and quality appraisal of multilayered groundwater in the Hongdunzi coal mine, northwest China. Mine Water Environ. 2018;37:222–237. [ Google Scholar ]
- Li P, He X, Guo W. Spatial groundwater quality and potential health risks due to nitrate ingestion through drinking water: a case study in Yan’an City on the Loess Plateau of northwest China. Hum Ecol Risk Assess. 2019;25:11–31. [ Google Scholar ]
- Li P, He X, Li Y, Xiang G. Occurrence and health implication of fluoride in groundwater of Loess aquifer in the Chinese Loess Plateau: a case study of Tongchuan, northwest China. Expo Health. 2019;11(2):95–107. doi: 10.1007/s12403-018-0278-x. [ DOI ] [ Google Scholar ]
- Lin H. Earth’s critical zone and hydropedology: concepts, characteristics, and advances. Hydrol Earth Syst Sci. 2010;14:25–45. [ Google Scholar ]
- Lokhandwala S, Gautam P. Indirect impact of COVID-19 on environment: a brief study in Indian context. Environ Res. 2020;188:109807. doi: 10.1016/j.envres.2020.109807. [ DOI ] [ PMC free article ] [ PubMed ] [ Google Scholar ]
- Lytle DA, Sorg T, Wang L, Chen A. The accumulation of radioactive contaminants in drinking water distribution systems. Water Res. 2014;50:396–407. doi: 10.1016/j.watres.2013.10.050. [ DOI ] [ PubMed ] [ Google Scholar ]
- MacDonald JA, Kavanaugh MC. Restoring contaminated groundwater: An achievable goal? Environ Sci Technol. 1994;28(8):362A–368A. doi: 10.1021/es00057a714. [ DOI ] [ PubMed ] [ Google Scholar ]
- Marghade D, Malpe DB, Duraisamy K, Patil PD, Li P. Hydrogeochemical evaluation, suitability, and health risk assessment of groundwater in the watershed of Godavari basin, Maharashtra, Central India. Environ Sci Pollut Res. 2020 doi: 10.1007/s11356-020-10032-7. [ DOI ] [ PubMed ] [ Google Scholar ]
- Meffe R, Bustamante I. Emerging organic contaminants in surface water and groundwater: a first overview of the situation in Italy. Sci Total Environ. 2014;481:280–295. doi: 10.1016/j.scitotenv.2014.02.053. [ DOI ] [ PubMed ] [ Google Scholar ]
- Mthembu PP, Elumalai V, Brindha K, Li P. Hydrogeochemical processes and trace metal contamination in groundwater: impact on human health in the Maputaland coastal aquifer, South Africa. Expo Health. 2020;12:403–426. [ Google Scholar ]
- Njuguna SM, Makokha VA, Yan X, Gituru RW, Wang Q, Wang J. Health risk assessment by consumption of vegetables irrigated with reclaimed wastewater: a case study in Thika (Kenya) J Environ Manag. 2019;231:576–581. doi: 10.1016/j.jenvman.2018.10.088. [ DOI ] [ PubMed ] [ Google Scholar ]
- Ololade IA, Arogunrerin IA, Oladoja NA, Ololade OO, Alabi AB (2021) Concentrations and toxic equivalency of polycyclic aromatic hydrocarbons (PAHs) and polychlorinated biphenyl (PCB) congeners in groundwater around waste dumpsites in South-West Nigeria. Arch Environ Contam Toxicol ( this issue ) [ DOI ] [ PubMed ]
- Pandey HK, Duggal SK, Jamatia A. Fluoride contamination of groundwater and its hydrological evolution in District Sonbhadra (U.P.) India. Proc Nat Acad Sci India Sect A Phys Sci. 2016;86:81–93. [ Google Scholar ]
- Prakash R, Srinivasamoorthy K, Sundarapandian SM, Nanthakumar C, Gopinath S, Saravanan K, Vinnarasi F (2021) Submarine groundwater discharge from an urban estuary to southeastern bay of Bengal, India: revealed by trace element fluxes. Arch Environ Contam Toxicol ( this issue ). 10.1007/s00244-020-00774-3 [ DOI ] [ PubMed ]
- Raja V, Lakshmi RV, Sekar CP, Chidambaram S, Neelakantan MA (2021) Evaluation of human health risk assessment of heavy metals in groundwater of the industrial township of Virudhunagar, Tamilnadu, India. Arch Environ Contam Toxicol ( this issue ) [ DOI ] [ PubMed ]
- Rebelo FM, Caldas ED (2016) Arsenic, lead, mercury and cadmium: toxicity, levels in breast milk and the risks for breastfed infants. Environ Res 51:671–688 [ DOI ] [ PubMed ]
- Ren X, Li P, He X, Su F, Elumalai V (2021) Hydrogeochemical processes affecting groundwater chemistry in the central part of the Guanzhong Basin, China. Environ Contam Toxicol ( this issue ). 10.1007/s00244-020-00772-5 [ DOI ] [ PubMed ]
- Sawyer AH, Michael HA, Schroth AW. From soil to sea: the role of groundwater in coastal critical zone processes. WIREs Water. 2016;3:706–726. [ Google Scholar ]
- Schillinger J, Özerol G, Güven-Griemert Ş, Heldeweg M. Water in war: understanding the impacts of armed conflict on water resources and their management. WIREs Water. 2020;7:e1480. doi: 10.1002/wat2.1480. [ DOI ] [ Google Scholar ]
- Schulze S, Zahn D, Montes R, Rodil R, Quintana JB, Knepper TP, Reemtsma T, Berger U. Occurrence of emerging persistent and mobile organic contaminants in European water samples. Water Res. 2019;153:80–90. doi: 10.1016/j.watres.2019.01.008. [ DOI ] [ PubMed ] [ Google Scholar ]
- Serio F, Miglietta PP, Lamastra L, Ficocelli S, Intini F, De Leo F, De Donno A. Groundwater nitrate contamination and agricultural land use: a grey water footprint perspective in Southern Apulia Region (Italy) Sci Total Environ. 2018;645:1425–1431. doi: 10.1016/j.scitotenv.2018.07.241. [ DOI ] [ PubMed ] [ Google Scholar ]
- Shen J, Gao Y. Groundwater and the environment. Wuhan: China University of Geoscience Press; 1995. [ Google Scholar ]
- Shukla S, Saxena A (2021) Appraisal of groundwater quality with human health risk assessment in parts of Indo-Gangetic alluvial plain, North India. Arch Environ Contam Toxicol ( this issue ). 10.1007/s00244-020-00771-6 [ DOI ] [ PubMed ]
- Sorensen JPR, Lapworth DJ, Nkhuwa DCW, Stuart ME, Gooddy DC, Bell RA, Chirwa M, Kabika J, Liemisa M, Chibesa M, Pedley S. Emerging contaminants in urban groundwater sources in Africa. Water Res. 2015;72:51–63. doi: 10.1016/j.watres.2014.08.002. [ DOI ] [ PubMed ] [ Google Scholar ]
- Su Z, Wu J, He X, Elumalai V. Temporal changes of groundwater quality within the groundwater depression cone and prediction of confined groundwater salinity using Grey Markov model in Yinchuan area of northwest China. Expo Health. 2020;12:447–468. [ Google Scholar ]
- Subba Rao N, Ravindra B, Wu J. Geochemical and health risk evaluation of fluoride rich groundwater in Sattenapalle Region, Guntur district, Andhra Pradesh, India. Hum Ecol Risk Assess. 2020;26:2316–2348. [ Google Scholar ]
- Subba Rao N, Dinakar A, Kumari BK, Karunanidhi D, Kamalesh T (2021) Seasonal and spatial variation of groundwater quality vulnerable zones of Yellareddygudem watershed, Nalgonda district, Telangana State, India. Arch Environ Contam Toxicol ( this issue ). 10.1007/s00244-020-00783-2 [ DOI ] [ PubMed ]
- Tatti F, Papini MP, Torretta V, Mancini G, Boni MR, Viotti P. Experimental and numerical evaluation of groundwater circulation wells as a remediation technology for persistent, low permeability contaminant source zones. J Contam Hydrol. 2019;222:89–100. doi: 10.1016/j.jconhyd.2019.03.001. [ DOI ] [ PubMed ] [ Google Scholar ]
- Teng Y, Hu B, Zheng J, Zhai Y, Zhu C. Water quality responses to the interaction between surface water and groundwater along the Songhua River, NE China. Hydrogeol J. 2018;26:1591–1607. [ Google Scholar ]
- Wang D, Wang L, Yang Q, Yu K, Ma H (2021) Hydrogeochemistry assessment of shallow groundwater and its health threat on human in northwestern Ordos basin, China. Arch Environ Contam Toxicol ( this issue ) [ DOI ] [ PubMed ]
- Wang D, Wu J, Wang Y, Ji Y. Finding high-quality groundwater resources to reduce the hydatidosis incidence in the Shiqu County of Sichuan Province, China: analysis, assessment, and management. Expo Health. 2020;12:307–322. [ Google Scholar ]
- Wu J, Li P, Qian H. Hydrochemical characterization of drinking groundwater with special reference to fluoride in an arid area of China and the control of aquifer leakage on its concentrations. Environ Earth Sci. 2015;73:8575–8588. [ Google Scholar ]
- Wu J, Li P, Qian H, Fang Y (2014) Assessment of soil salinization based on a low-cost method and its influencing factors in a semi-arid agricultural area, northwest China. Environ Earth Sci 71(8):3465–3475. 10.1007/s12665-013-2736-x
- Wu J, Sun Z. Evaluation of shallow groundwater contamination and associated human health risk in an alluvial plain impacted by agricultural and industrial activities, mid-west China. Expo Health. 2016;8:311–329. [ Google Scholar ]
- Wu J, Zhang Y, Zhou H (2020) Groundwater chemistry and groundwater quality index incorporating health risk weighting in Dingbian County, Ordos basin of northwest China. Geochemistry 80(4):125607. 10.1016/j.chemer.2020.125607
- Yuan Y, Xiang M, Liu C, Theng BKG. Chronic impact of an accidental wastewater spill from a smelter, China: a study of health risk of heavy metal(loid)s via vegetable intake. Ecotoxicol Environ Saf. 2019;182:109401. doi: 10.1016/j.ecoenv.2019.109401. [ DOI ] [ PubMed ] [ Google Scholar ]
- Zhang Y, Wu J, Xu B. Human health risk assessment of groundwater nitrogen pollution in Jinghui canal irrigation area of the loess region, northwest China. Environ Earth Sci. 2018;77:273. [ Google Scholar ]
- Zhao H, Song F, Su F, Shen Y, Li P (2021) Removal of cadmium from contaminated groundwater using a novel silicon/aluminum nanomaterial: an experimental study. Arch Environ Contam Toxicol ( this issue ). 10.1007/s00244-020-00784-1 [ DOI ] [ PubMed ]
- Zhou Y, Li P, Chen M, Dong Z, Lu C. Groundwater quality for potable and irrigation uses and associated health risk in southern part of Gu’an County, North China Plain. Environ Geochem Health. 2020 doi: 10.1007/s10653-020-00553-y. [ DOI ] [ PubMed ] [ Google Scholar ]
- View on publisher site
- PDF (917.4 KB)
- Collections
Similar articles
Cited by other articles, links to ncbi databases.
- Download .nbib .nbib
- Format: AMA APA MLA NLM
Add to Collections
Information
- Author Services
Initiatives
You are accessing a machine-readable page. In order to be human-readable, please install an RSS reader.
All articles published by MDPI are made immediately available worldwide under an open access license. No special permission is required to reuse all or part of the article published by MDPI, including figures and tables. For articles published under an open access Creative Common CC BY license, any part of the article may be reused without permission provided that the original article is clearly cited. For more information, please refer to https://www.mdpi.com/openaccess .
Feature papers represent the most advanced research with significant potential for high impact in the field. A Feature Paper should be a substantial original Article that involves several techniques or approaches, provides an outlook for future research directions and describes possible research applications.
Feature papers are submitted upon individual invitation or recommendation by the scientific editors and must receive positive feedback from the reviewers.
Editor’s Choice articles are based on recommendations by the scientific editors of MDPI journals from around the world. Editors select a small number of articles recently published in the journal that they believe will be particularly interesting to readers, or important in the respective research area. The aim is to provide a snapshot of some of the most exciting work published in the various research areas of the journal.
Original Submission Date Received: .
- Active Journals
- Find a Journal
- Journal Proposal
- Proceedings Series
- For Authors
- For Reviewers
- For Editors
- For Librarians
- For Publishers
- For Societies
- For Conference Organizers
- Open Access Policy
- Institutional Open Access Program
- Special Issues Guidelines
- Editorial Process
- Research and Publication Ethics
- Article Processing Charges
- Testimonials
- Preprints.org
- SciProfiles
- Encyclopedia
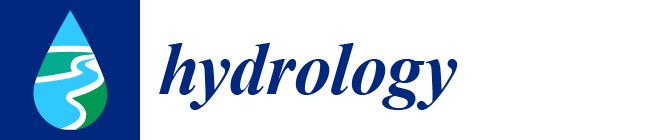
Journal Menu
- Hydrology Home
- Aims & Scope
- Editorial Board
- Topical Advisory Panel
- Instructions for Authors
- Special Issues
- Sections & Collections
- Article Processing Charge
- Indexing & Archiving
- Editor’s Choice Articles
- Most Cited & Viewed
- Journal Statistics
- Journal History
- Journal Awards
- Society Collaborations
- Conferences
- Editorial Office
- 10th Anniversary
Journal Browser
- arrow_forward_ios Forthcoming issue arrow_forward_ios Current issue
- Vol. 11 (2024)
- Vol. 10 (2023)
- Vol. 9 (2022)
- Vol. 8 (2021)
- Vol. 7 (2020)
- Vol. 6 (2019)
- Vol. 5 (2018)
- Vol. 4 (2017)
- Vol. 3 (2016)
- Vol. 2 (2015)
- Vol. 1 (2014)
Find support for a specific problem in the support section of our website.
Please let us know what you think of our products and services.
Visit our dedicated information section to learn more about MDPI.
Groundwater Pollution: Sources, Mechanisms, and Prevention
Special issue editors, special issue information, benefits of publishing in a special issue.
- Published Papers
A special issue of Hydrology (ISSN 2306-5338). This special issue belongs to the section " Surface Waters and Groundwaters ".
Deadline for manuscript submissions: closed (29 February 2024) | Viewed by 27183
Share This Special Issue

Dear Colleagues,
Groundwater resources are vital for ecosystems and for human health and prosperity. Groundwater pollution continues to increase, a fact that further limits the potential of groundwater resources for use. The sources of contaminants can be natural (e.g., salinity or arsenic) or anthropogenic (excess fertilizers, pesticides, industrial chemicals, sewage effluent). Groundwater pollution may emerge from point sources (which can be well identified in space) and nonpoint sources, which are more difficult to identify, measure, and control than the former. To deal with this phenomenon, the sources of pollution and the mechanisms of the fate and transport of pollutants in the groundwater must be identified. This procedure is quite important because it forms the basis on which the appropriate prevention or mitigation measures will be generated. The latter are preferable to any remediation effort, as adverse effects on the environment and the health of living organisms are prevented. However, since groundwater contamination is less obvious than surface water pollution, it frequently goes unnoticed. In this case, it is mandatory that a remediation strategy be undertaken.
This Special Issue entitled “Groundwater Pollution: Sources, Mechanisms, and Prevention” aims to present new research contributions in the area of groundwater contamination, and will focus on the sources, effects, and exposure of natural and artificial groundwater pollutants, hydrological processes, and hydrochemical properties of groundwater; the intrinsic and specific vulnerability of groundwater to pollution; human health risk assessment; and recent trends in management and pollution mitigation, prevention and remediation strategies.
Potential themes include, but are not limited to, the following:
- Investigating anthropogenic and natural sources of groundwater contamination;
- Intrinsic and specific vulnerability of groundwater to pollution;
- Monitoring of groundwater quality;
- GIS spatial analyses;
- Numerical simulation and statistical analysis of groundwater flow and contamination transport;
- Human health risk assessment of groundwater;
- Impact of climate change on groundwater pollution;
- Prevention and mitigation measures, remediation techniques and policy-making.
Dr. Pantelis Sidiropoulos Dr. Augustina Clara Alexander Guest Editors
Manuscripts should be submitted online at www.mdpi.com by registering and logging in to this website . Once you are registered, click here to go to the submission form . Manuscripts can be submitted until the deadline. All submissions that pass pre-check are peer-reviewed. Accepted papers will be published continuously in the journal (as soon as accepted) and will be listed together on the special issue website. Research articles, review articles as well as short communications are invited. For planned papers, a title and short abstract (about 100 words) can be sent to the Editorial Office for announcement on this website.
Submitted manuscripts should not have been published previously, nor be under consideration for publication elsewhere (except conference proceedings papers). All manuscripts are thoroughly refereed through a single-blind peer-review process. A guide for authors and other relevant information for submission of manuscripts is available on the Instructions for Authors page. Hydrology is an international peer-reviewed open access monthly journal published by MDPI.
Please visit the Instructions for Authors page before submitting a manuscript. The Article Processing Charge (APC) for publication in this open access journal is 1800 CHF (Swiss Francs). Submitted papers should be well formatted and use good English. Authors may use MDPI's English editing service prior to publication or during author revisions.
- groundwater
- hydrochemistry
- water quality
- vulnerability
- remediation
- Ease of navigation: Grouping papers by topic helps scholars navigate broad scope journals more efficiently.
- Greater discoverability: Special Issues support the reach and impact of scientific research. Articles in Special Issues are more discoverable and cited more frequently.
- Expansion of research network: Special Issues facilitate connections among authors, fostering scientific collaborations.
- External promotion: Articles in Special Issues are often promoted through the journal's social media, increasing their visibility.
- e-Book format: Special Issues with more than 10 articles can be published as dedicated e-books, ensuring wide and rapid dissemination.
Further information on MDPI's Special Issue polices can be found here .
Published Papers (12 papers)
Jump to: Research
Jump to: Editorial
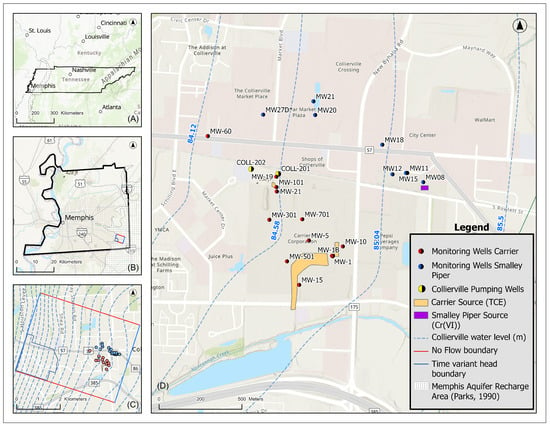

Further Information
Mdpi initiatives, follow mdpi.
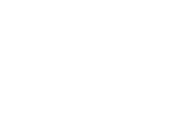
Subscribe to receive issue release notifications and newsletters from MDPI journals
Advertisement
A Comprehensive Review on Groundwater Contamination Due to Sewer Leakage: Sources, Detection Techniques, Health Impacts, Mitigation Methods
- Published: 05 January 2024
- Volume 235 , article number 56 , ( 2024 )
Cite this article
- D. Sridhar 1 &
- S. Parimalarenganayaki ORCID: orcid.org/0000-0001-7174-630X 1
715 Accesses
Explore all metrics
Groundwater pollution poses a significant threat globally, particularly in developing countries where inadequate sanitation facilities contribute to growing concerns about contamination from sewer leaks. Hence, the objective of this study is to present a comprehensive review, offering insights into diverse aspects of sewer leaks and their impacts on the urban groundwater system. This includes an exploration of leak sources, methods for leak detection, quantification approaches, analysis of contaminants in sewage along with their health effects, and strategies for mitigating both sewer leaks and groundwater contamination. This review addresses various factors leading to sewer infrastructure damage, emphasizing its importance in effective maintenance strategies. In this review, a range of contaminants released from sewer leaks were outlined, ranges from emerging contaminants to heavy metals that poses risk to the human health and environment. Further it evaluates various methods for detecting sewer leaks, emphasizing advancements in water quality analysis, visual, electromagnetic, and acoustics techniques. This research assesses diverse techniques for quantifying sewage leaks, including mass balance and wastewater balance and concludes pinpointing specific leak hotspots remains challenging. Furthermore, an appraisal of mitigation measures was also conducted, determining that rehabilitation serves as a more effective approach to stop leaks at their source. This paper delves into groundwater treatment methods, highlighting the difficulties in achieving optimal water quality and reveals that technologies such as Permeable Reactive Barrier and advanced oxidation processes exhibit potential in effectively removing trace-level pollutants. Overall, the review underscores the importance of understanding, detecting, and mitigating sewer leakage for the health and sustainability of groundwater systems.
This is a preview of subscription content, log in via an institution to check access.
Access this article
Subscribe and save.
- Get 10 units per month
- Download Article/Chapter or eBook
- 1 Unit = 1 Article or 1 Chapter
- Cancel anytime
Price includes VAT (Russian Federation)
Instant access to the full article PDF.
Rent this article via DeepDyve
Institutional subscriptions
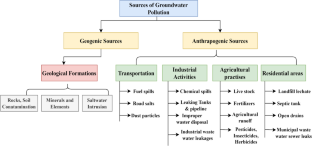
Similar content being viewed by others
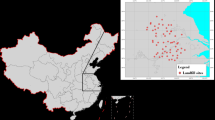
Assessment of the impact of landfills on groundwater quality in Eastern China: a comprehensive analysis of inorganic solutes
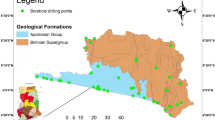
Assessment of groundwater quantity, quality, and associated health risk of the Tano river basin, Ghana
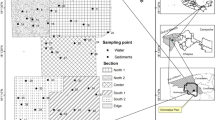
Analysis of Groundwater Quality in Rural Homes in Southeastern Mexico: Comparison of Treatment Methods
Data availability.
Not applicable.
Adetunji, V. O., & Odetokun, I. A. (2011). Groundwater contamination in Agbowo community, Ibadan Nigeria: Impact of septic tanks distances to wells. Malaysian Journal of Microbiology, 7 (3), 159–166.
Google Scholar
Adimalla, N., Li, P., & Qian, H. (2019). Evaluation of groundwater contamination for fuoride and nitrate in semiarid region of Nirmal Province, South India: A special emphasis on human health risk assessment (HHRA). Human and Ecological Risk Assessment: An International Journal , 25 , 1107–1124.
Ahamad, A., Madhav, S., Singh, P., Pandey, J., & Khan, A. H. (2018). Assessment of groundwater quality with special emphasis on nitrate contamination in parts of Varanasi City, Uttar Pradesh, India. Applied Water Science, 8 (4), 1–13.
CAS Google Scholar
Akthar, A., Parveen, M., & Qayoom, I. (2015). Limnological features of Dal Lake of Kashmir valley with special reference to the anthropogenic pressure along its water shed. Ecology, Environment and Conservation, 21 , 497–803.
Al-Hashimi, O., Hashim, K., Loffill, E., Marolt Čebašek, T., Nakouti, I., Faisal, A. A., & Al-Ansari, N. (2021). A comprehensive review for groundwater contamination and remediation: Occurrence, migration and adsorption modelling. Molecules, 26 (19), 5913.
Ali, M. M., Hossain, D., Al-Imran, A., Khan, M. S., Begum, M., & Osman, M. H. (2021). Environmental pollution with heavy metals: A public health concern. Heavy Metals- Their Environmental Impacts and Mitigation , 771–783. https://doi.org/10.5772/intechopen.96805
Amran, T. S. T., Amin, M. S. M., Ahmad, M. R., Sani, S., Masenwat, N. A., Bundak, C. E. A., & Singoi, C. J. (2019). A study on detection water leakage of underground metal and PVC pipes using ground penetrating radar. In IOP Conference Series: Materials Science and Engineering (Vol. 555, No. 1, p. 012012). IOP Publishing.
Askar, A. H., & Illangasekare, T. H. (2023). Controlling potential far-field brine leakage from CO2 storage formations using deep extraction wells: Numerical and experimental testing. Greenhouse Gases: Science and Technology, 13 (1), 3–30.
Bazzoli, G., Visintainer, M., Arman, S., & Gottardi, C. (2005). Caffeine as an anthropogenic marker of pollution of groundwater. Possible uses in mountain basins . Geologia Tecnica e Ambientale, 4 , 5–16.
Biswas, T. K., Jayawardane, N. S., Blackwell, J., Christen, E. W., & Cook, F. J. (1999). The “FILTER” technique for year round treatment of wastewater. In Proc. On-site (Vol. 99, pp. 59–65).
Blankenberg, A. G. B., Paruch, A. M., Paruch, L., Deelstra, J., & Haarstad, K. (2016). Nutrients tracking and removal in constructed wetlands treating catchment runoff in Norway. Natural and Constructed Wetlands: Nutrients, heavy metals and energy cycling, and flow (pp. 23–40). Springer International Publishing.
Burn, S., Desilva, D., Eiswirth, M., Hunaidi, O., Speers, A., & Thornton, J. (1999). Pipe leakage—future challenges and solutions. Pipes Wagga Wagga , Australia
Cao, Y. S., Tang, J. G., Henze, M., Yang, X. P., Gan, Y. P., Li, J., & Daigger, G. T. (2019). The leakage of sewer systems and the impact on the ‘black and odorous water bodies’ and WWTPs in China. Water Science and Technology, 79 (2), 334–341.
Careghini, A., Saponaro, S., & Sezenna, E. (2013). Biobarriers for groundwater treatment: A review. Water Science and Technology, 67 (3), 453–468. https://doi.org/10.2166/wst.2012.599
Article CAS Google Scholar
Carr, R., & Strauss, M. (2001). Excreta-related infections and the role of sanitation in the control of transmission. Water quality: guidelines, standards and health , 89–113.
Casasso, A., Tosco, T., Bianco, C., Bucci, A., & Sethi, R. (2019). How can we make pump and treat systems more energetically sustainable? Water, 12 (1), 67.
Chung, H. I., & Lee, M. (2007). A new method for remedial treatment of contaminated clayey soils by electrokinetics coupled with permeable reactive barriers. Electrochimica Acta, 52 (10), 3427–3431.
Costello, S. B., Chapman, D. N., Rogers, C. D. F., & Metje, N. (2007). Underground asset location and condition assessment technologies. Tunnelling and Underground Space Technology, 22 (5–6), 524–542.
Da’ana, D. A., Zouari, N., Ashfaq, M. Y., Abu-Dieyeh, M., Khraisheh, M., Hijji, Y. M., & Al-Ghouti, M. A. (2021). Removal of toxic elements and microbial contaminants from groundwater using low-cost treatment options. Current Pollution Reports, 7 (3), 300–324.
Damvergis, C. N. (2014). Sewer systems: Failures and rehabilitation. Water Utility Journal, 8 , 17–24.
Daughton, C. G., & Ternes, T. A. (1999). Pharmaceuticals and personal care products in the environment: Agents of subtle change? Environmental Health Perspectives, 107 (suppl 6), 907–938.
Do, N. C., Dix, L., Lambert, M. F., & Stephens, M. L. (2023). Proactive detection of wastewater overflows for smart sanitary sewer systems: case study in South Australia. Journal of Water Resources Planning and Management , 149 (1), 05022016. https://doi.org/10.1061/JWRMD5.WRENG-5589
Easa, A., & Abou-Rayan, A. (2010). Domestic wastewater effect on the pollution of the groundwater in rural areas in Egypt. Aquatic, 3 (4), 5.
Eiswirth, M., Heske, C., Hötzl, H., Schneider, T., & Burn, L. S. (2000). Pipe defect characterisation by multi-sensor systems. In Proceedings of 18th International Conference of No-Dig (October 2000) .
Elmasry, M., Hawari, A., & Zayed, T. (2018). An economic loss model for failure of sewer pipelines. Structure and Infrastructure Engineering, 14 (10), 1312–1323.
EPA. (2002).Onsite wastewater treatment system manual. EPA/625/R-00/ 008 (NTIS PB02-108560). Environmental Protection Agency , Washington DC.
Fennell, C., Misstear, B., O’Connell, D., Dubber, D., Behan, P., Danaher, M., & Gill, L. (2021). An assessment of contamination fingerprinting techniques for determining the impact of domestic wastewater treatment systems on private well supplies. Environmental Pollution, 268 , 115687. https://doi.org/10.1016/j.envpol.2020.115687
Ganesan, P. (2017). Review of Household use of Septic Tanks and Fecal Sludge Management in Rural India. CLTS Knowledge Hub , 1–17.
Ganesh, D. E. (2017). Detection and locating leaks in underground water mains using thermography. International Journal of Engineering Applied Sciences and Technology, 2(3), 117–121. DOI , 10 , m9.
Gokçekuş, H., Kassem, Y., Yunusa, N., Musa, M. K., John, S. O., Usman, S., ... & Ahmad, S. M. (2020). Study on pit latrine minimum design requirement and considerations in Northern Nigeria. International Journal of Scientific & Technology Research , 2277–8616.
Gongora, G. R. A., Lu, R. H., & El Hanandeh, A. (2021). Comparative life cycle assessment of aerobic treatment units and constructed wetlands as onsite wastewater treatment systems in Australia. Water Science and Technology, 84 (6), 1527–1540.
Gotkowitz, M. B., Bradbury, K. R., Borchardt, M. A., Zhu, J., & Spencer, S. K. (2016). Effects of climate and sewer condition on virus transport to groundwater. Environmental Science & Technology, 50 (16), 8497–8504. https://doi.org/10.1021/acs.est.6b01422
Grimmeisen, F., Lehmann, M. F., Liesch, T., Goeppert, N., Klinger, J., Zopfi, J., & Goldscheider, N. (2017). Isotopic constraints on water source mixing, network leakage and contamination in an urban groundwater system. Science of the Total Environment, 583 , 202–213. https://doi.org/10.1016/j.scitotenv.2017.01.0
Guérineau, H., Dorner, S., Carrière, A., McQuaid, N., Sauvé, S., Aboulfadl, K., … Prévost, M. (2014). Source tracking of leaky sewers: A novel approach combining fecal indicators in water and sediments . Water Research, 58 , 50–61. https://doi.org/10.1016/j.watres.2014.03.057
Hale, R. L., Turnbull, L., Earl, S., Grimm, N., Riha, K., Michalski, G., Lohse, K. A., & Childers, D. (2014). Sources and transport of nitrogen in arid urban watersheds. Environmental Science and Technology, 48 , 6211–6219.
Hamilton, S., & Charalambous, B. (2013). Leak detection: technology and implementation (p. 112) . IWA Publishing.
Hanski, J., Luomanen, T., Kortelainen, H., & Välisalo, T. (2013). Methods for value assessment of water and sewer pipelines. International Journal of Strategic Engineering Asset Management 3 , 1 (4), 390–406.
Hao, T., Rogers, C. D. F., Metje, N., Chapman, D. N., Muggleton, J. M., Foo, K. Y., ... & Saul, A. J. (2012). Condition assessment of the buried utility service infrastructure. Tunnelling and Underground Space Technology , 28 , 331–344.
Harris, R. J., & Tasello, J. (2004). Sewer leak detection-electro-scan adds a new dimension case study: City Of Redding, California. In Pipeline Engineering and Construction: What's on the Horizon? (pp. 1–11). https://doi.org/10.1061/40745(146)3
Hawari, A., Alamin, M., Alkadour, F., Elmasry, M., & Zayed, T. (2018). Automated defect detection tool for closed circuit television (cctv) inspected sewer pipelines. Automation in Construction, 89 , 99–109.
Hayward, J. L., Huang, Y., Yost, C. K., Hansen, L. T., Lake, C., Tong, A., & Jamieson, R. C. (2019). Lateral flow sand filters are effective for removal of antibiotic resistance genes from domestic wastewater. Water Research, 162 , 482–491.
Held, I., Wolf, L., Eiswirth, M., & Hötzl, H. (2006). Impacts of sewer leakage on urban groundwater: Review of a case study in Germany. In Urban groundwater management and sustainability (pp. 189–204). Springer Netherlands.
Heo, G., Jeon, J., & Son, B. (2019). Crack automatic detection of CCTV video of sewer inspection with low resolution. KSCE Journal of Civil Engineering, 23 , 1219–1227.
InfraGuide. (2004). Assessment and Evaluation of Storm and Wastewater Collection Systems, InfraGuide (IG) Best Practice , The National Guide to Sustainable Municipal Infrastructure, Ottawa, Ontario.
Kanmani, S., & Gandhimathi, R. (2013). Assessment of heavy metal contamination in soil due to leachate migration from an open dumping site. Applied Water Science, 3 , 193–205.
Kasonga, T. K., Coetzee, M. A., Kamika, I., Ngole-Jeme, V. M., & Momba, M. N. B. (2021). Endocrine-disruptive chemicals as contaminants of emerging concern in wastewater and surface water: A review. Journal of Environmental Management, 277 , 111485.
Kenway, S., Gregory, A., & McMahon, J. (2011). Urban water mass balance analysis. Journal of Industrial Ecology, 15 (5), 693–706.
Kumwenda, S. (2019). Challenges to hygiene improvement in developing countries (Vol. 1, pp. 1–19). IntechOpen.
Lam, T., Yang, X., Ergas, S. J., & Arias, M. E. (2023). Feasibility of landfill leachate reuse through adsorbent-enhanced constructed wetlands and ultrafiltration-reverse osmosis. Desalination, 545 , 116163.
Lapworth, D. J., Baran, N., Stuart, M. E., & Ward, R. S. (2012). Emerging organic contaminants in groundwater: A review of sources, fate and occurrence. Environmental Pollution, 163 , 287–303.
Larrarte, F., Lepot, M., Clemens, F. H. L. R., Bertrand-Krajewski, J-L., Ivetic, D., Prodanovic, D., & Stegeman, B. (2021). Water level and discharge measurements. Metrology in Urban Drainage and Stormwater Management: Plug and Pray (pp. 35-104). International Water Association (IWA). https://doi.org/10.2166/9781789060119_0035
Latosińska, J., Kowalik, R., & Gawdzik, J. (2021). Risk assessment of soil contamination with heavy metals from municipal sewage sludge. Applied Sciences, 11 (2), 548.
LeChevallier, M. W., Gullick, R. W., Karim, M. R., Friedman, M., & Funk, J. E. (2003). The potential for health risks from intrusion of contaminants into the distribution system from pressure transients. Journal of Water and Health, 1 (1), 3–14.
Lei, M., Zhang, L., Lei, J., Zong, L., Li, J., Wu, Z., & Wang, Z. (2015). Overview of emerging contaminants and associated human health effects. BioMed research international, 2015 . https://doi.org/10.1155/2015/404796
Li, J., Zhou, M., Si, Y. N., & Li, J. J. (2014). Trenchless repair technology and application of urban sewer system. Applied Mechanics and Materials, 470 , 992–997.
Ling, C., Revil, A., Qi, Y., Abdulsamad, F., Shi, P., Nicaise, S., & Peyras, L. (2019). Application of the Mise-à-la-Masse method to detect the bottom leakage of water reservoirs. Engineering Geology, 261 , 105272.
Liu, Z., & Kleiner, Y. (2013). State of the art review of inspection technologies for condition assessment of water pipes. Measurement, 46 (1), 1–15. https://doi.org/10.1016/j.measurement.2012.05.032
Article Google Scholar
Liu, P., Xu, C., Xie, J., Fu, M., Chen, Y., Liu, Z., & Zhang, Z. (2023). A CNN-based transfer learning method for leakage detection of pipeline under multiple working conditions with AE signals. Process Safety and Environmental Protection, 170 , 1161–1172.
Liu, Z., Kleiner, Y., Rajani, B., Wang, L., & Condit, W. (2012). Condition Assessment Technologies for Water Transmission and Distribution Systems. U.S. Environmental Protection Agency , Washington, DC, EPA/600/R-12/017, 2012.
Lu, H., Xu, Z. D., Iseley, T., Peng, H., & Fu, L. (2023). Application and cases of pipeline inspection and monitoring. In Pipeline Inspection and Health Monitoring Technology: The Key to Integrity Management (pp. 257–285). Springer Nature Singapore.
MalekMohammadi, M., Najafi, M., Kermanshachi, S., Kaushal, V., & Serajiantehrani, R. (2020). Factors influencing the condition of sewer pipes: State-of-the-art review. Journal of Pipeline Systems Engineering and Practice, 11 (4), 03120002. https://doi.org/10.1061/(asce)ps.1949-1204.0000483
Maxcy-Brown, J., Elliott, M. A., Krometis, L. A., Brown, J., White, K. D., & Lall, U. (2021). Making waves: Right in our backyard-surface discharge of untreated wastewater from homes in the United States. Water Research, 190 , 116647.
Mohamed, M. A. H., Ramadan, M. A., & El-Dash, K. M. (2023). Cost optimization of sewage pipelines inspection. Ain Shams Engineering Journal, 14 (5), 101960.
Mohammed, M. H., Zwain, H. M., & Hassan, W. H. (2021). Modeling the impacts of climate change and flooding on sanitary sewage system using SWMM simulation: A case study. Results in Engineering, 12 , 100307.
Mostafa, M. K., Chawla, R. C., & Peters, R. W. (2016). 7 Wastewater treatment, reuse, and disposal. Sustainable Water Technologies , 199. https://doi.org/10.1201/9781315373867
Müller, A., Österlund, H., Marsalek, J., & Viklander, M. (2020). The pollution conveyed by urban runoff: A review of sources. Science of the Total Environment, 709 , 136125.
Murray, C. J., & Lopez, A. D. (1996). Global health statistics: A compendium of incidence, prevalence and mortality estimates for over 200 conditions. In Global health statistics: a compendium of incidence, prevalence and mortality estimates for over 200 conditions (pp. 906–906).
Musolff, A., Leschik, S., Reinstorf, F., Strauch, G., & Schirmer, M. (2010). Micropollutant loads in the urban water cycle. Environmental Science & Technology, 44 (13), 4877–4883.
Mwegoha, W. J. (2008). The use of phytoremediation technology for abatement soil and groundwater pollution in Tanzania: Opportunities and challenges. Journal of Sustainable Development in Africa, 10 (1), 140–156.
Nabeela, F., Azizullah, A., Bibi, R., Uzma, S., Murad, W., Shakir, S. K., & Häder, D. P. (2014). Microbial contamination of drinking water in Pakistan—A review. Environmental Science and Pollution Research, 21 , 13929–13942.
Nguyen, H. H., Peche, A., & Venohr, M. (2021). Modelling of sewer exfiltration to groundwater in urban wastewater systems: A critical review. Journal of Hydrology, 596 , 126130.
Nielsen, G. D., Nielsen, J. B., Andersen, K. E., & Grandjean, P. (2000). Effects of industrial detergents on the barrier function of human skin. International Journal of Occupational and Environmental Health, 6 (2), 138–142.
Nnaji, N. D., Onyeaka, H., Miri, T., & Ugwa, C. (2023). Bioaccumulation for heavy metal removal: A review. SN Applied Sciences, 5 (5), 125.
Noshahri, H., oldeScholtenhuis, L. L., Doree, A. G., & Dertien, E. C. (2021). Linking sewer condition assessment methods to asset managers’ data-needs. Automation in Construction, 131 , 103878.
O’Driscoll, M. A., Humphrey, C. P., Jr., Deal, N. E., Lindbo, D. L., & Zarate-Bermudez, M. A. (2014). Meteorological influences on nitrogen dynamics of a coastal onsite wastewater treatment system. Journal of Environmental Quality, 43 (6), 1873–1885.
Obradović, D., Šperac, M., & Marenjak, S. (2023). Challenges in sewer system maintenance. Encyclopedia, 3 (1), 122–142.
Ogawa, Y. (2000). Nitrate pollution in groundwater and change of farming methods (in Japanese). Noubunkyo, Tokyo , 68–71.
Okolo, C. K., & Meydan, T. (2018). Pulsed magnetic flux leakage method for hairline crack detection and characterization. AIP Advances, 8 (4), 047207.
Olds, H. T., Corsi, S. R., Dila, D. K., Halmo, K. M., Bootsma, M. J., & McLellan, S. L. (2018). High levels of sewage contamination released from urban areas after storm events: A quantitative survey with sewage specific bacterial indicators. PLOS Medicine, 15 (7), e1002614. https://doi.org/10.1371/journal.pmed.1002614
Pandey, P. K., Kass, P. H., Soupir, M. L., Biswas, S., & Singh, V. P. (2014). Contamination of water resources by pathogenic bacteria. AMB Express, 4 , 1–16.
Park, S., Lim, H., Tamang, B., Jin, J., Lee, S., Park, S., & Kim, Y. (2020). A preliminary study on leakage detection of deteriorated underground sewer pipes using aerial thermal imaging. International Journal of Civil Engineering, 18 , 1167–1178.
Parlak, B. O., & Yavasoglu, H. A. (2023). A comprehensive analysis of in-line inspection tools and technologies for steel oil and gas pipelines. Sustainability, 15 (3), 2783.
Pedrero, F., Kalavrouziotis, I., Alarcón, J. J., Koukoulakis, P., & Asano, T. (2010). Use of treated municipal wastewater in irrigated agriculture—Review of some practices in Spain and Greece. Agricultural Water Management, 97 (9), 1233–1241.
Pennino, M. J., Kaushal, S. S., Mayer, P. M., Utz, R. M., & Cooper, C. A. (2016). Stream restoration and sewers impact sources and fluxes of water, carbon, and nutrients in urban watersheds. Hydrology and Earth System Sciences, 20 (8), 3419–3439.
Perveen, S., Samad, A. B. D. U. S., Nazif, W., & Shah, S. (2012). Impact of sewage water on vegetables quality with respect to heavy metals in Peshawar, Pakistan. Pakistan Journal of Botany, 44 (6), 1923–1931.
Pollock, A. A. (1989). Acoustic Emission Inspection, Metals handbook. ASM International , 17 (1989), 278–294.
Prestor, J., Pestotnik, S., Meglič, P., & Janža, M. (2011). Model of environmental pressures and impacts; A.3.3 Final Report of INCOME Project (LIFE+ Programme); Geological Survey of Slovenia: Ljubljana, Slovenia , 2011 .
Prigiobbe, V., & Giulianelli, M. (2011). Quantification of sewer leakage by a continuous tracer method. Water Science and Technology, 64 (1), 132–138.
Qi, J., Xu, M., Zhang, W., Liu, Y., & Dai, X. (2022). Defect detection of pipeline inner surface based on coaxial digital image correlation with hypercentric lens. Materials, 15 (21), 7543.
Qin, X. S., Huang, G. H., Zeng, G. M., Chakma, A. (2008). Simulation-based optimization of dual-phase vacuum extraction to remove nonaqueous phase liquids in subsurface. Water Resources Research 44 , W04422. https://doi.org/10.1029/2006WR005496
Ratha, D., Babbar, R., Hariprasad, K. S., Ojha, C. S. P., Baranwal, M., Rout, P. R., & Parihar, A. (2023). Mathematical Transport System of Microconstituents. Microconstituents in the Environment: Occurrence, Fate, Removal and Management , 107-131., 107–131. https://doi.org/10.1002/9781119825289
Rayhana, R., Jiao, Y., Zaji, A., & Liu, Z. (2020). Automated vision systems for condition assessment of sewer and water pipelines. IEEE Transactions on Automation Science and Engineering, 18 (4), 1861–1878.
Reynolds, J. H., & Barrett, M. H. (2003). A review of the effects of sewer leakage on groundwater quality. Water and Environment Journal, 17 (1), 34–39.
Rizzo, P. (2010). Water and wastewater pipe nondestructive evaluation and health monitoring: a review. Advances in Civil Engineering, 2010 , 1–13.
Roehrdanz, P. R., Feraud, M., Ervin, J., Anumol, T., Jia, A., Park, M., ... & Holden, P. A. (2015). Wastewater compounds in urban shallow groundwater wells correspond to exfiltration probabilities of nearby sewers. Water Research , 85 , 467–475.
Romanelli, A., Soto, D. X., Matiatos, I., Martínez, D. E., & Esquius, S. (2020). A biological and nitrate isotopic assessment framework to understand eutrophication in aquatic ecosystems. Science of the Total Environment, 715 , 136909.
Rutsch, M., Rieckermann, J., Cullmann, J., Ellis, J. B., Vollertsen, J., & Krebs, P. (2008). Towards a better understanding of sewer exfiltration. Water Research, 42 (10–11), 2385–2394.
Sadeghikhah, A., Ahmed, E., & Krebs, P. (2022). Towards a decentralized solution for sewer leakage detection–A review. Water Science & Technology, 86 (5), 1034–1054.
Sanchez, W., & Egea, E. (2018). Health and environmental risks associated with emerging pollutants and novel green processes. Environmental Science and Pollution Research, 25 (7), 6085–6086.
Schirmer, M., Reinstorf, F., Leschik, S., Musolff, A., Krieg, R., Strauch, G., ... & Schirmer, K. (2011). Mass fluxes of xenobiotics below cities: Challenges in urban hydrogeology. Environmental Earth Sciences , 64 , 607–617.
Selvakumar, A., Tuccillo, M. E., Martel, K. D., Matthews, J. C., & Feeney, C. (2014). Demonstration and evaluation of state-of-the-art wastewater collection systems condition assessment technologies. Journal of Pipeline Systems Engineering and Practice, 5 (2), 04013018.
Shao, S., Yang, X., & Jia, C. (2022). Combining multi-source data to evaluate the leakage pollution and remediation effects of landfill. Journal of Hydrology, 610 , 127889.
Singh, A., & Adachi, S. (2013). Bathtub curves and pipe prioritization based on failure rate. Built Environment Project and Asset Management, 3 (1), 105–122.
Sonkamble, S., & Chandra, S. (2021). GPR for earth and environmental applications: Case studies from India. Journal of Applied Geophysics, 193 , 104422.
Speight, J. G. (2020). Remediation technologies (pp. 263–303). Butterworth-Heinemann.
Stuart, M., Lapworth, D., Crane, E., & Hart, A. (2012). Review of risk from potential emerging contaminants in UK groundwater. Science of the Total Environment, 416 , 1–21.
Suhag, R. (2016). Overview of ground water in India. PRS On Standing Committee On Water Resources, Legislative Research,(February), 12 p.
Thiruvenkatachari, R., Vigneswaran, S., & Naidu, R. (2008). Permeable reactive barrier for groundwater remediation. Journal of Industrial and Engineering Chemistry, 14 (2), 145–156.
Thomas, D. C., Christensen, B. Y., & Gee, K. L. (2015). Phase and amplitude gradient method for the estimation of acoustic vector quantities. The Journal of the Acoustical Society of America, 137 (6), 3366–3376.
Tomczak, E., & Zielińska, A. (2017). Example of sewerage system rehabilitation using trenchless technology. Ecological Chemistry and Engineering S, 24 (3), 405–416.
Tran, N. H., Urase, T., Ngo, H. H., Hu, J., & Ong, S. L. (2013). Insight into metabolic and cometabolic activities of autotrophic and heterotrophic microorganisms in the biodegradation of emerging trace organic contaminants. Bioresource Technology, 146 , 721–731.
Tran, N. H., Reinhard, M., & Gin, K. Y. H. (2018). Occurrence and fate of emerging contaminants in municipal wastewater treatment plants from different geographical regions-a review. Water Research, 133 , 182–207.
Tran, H. Q., Phan, T. K. T., Tanaka, S., & Gogina, E. (2023). The ability of reusing municipal treated water for irrigation the landscaping in Vietnam. The research in constructed wetland in municipal wastewater treatment plant, Danang city. In E3S Web of Conferences (Vol. 403, p. 06006). EDP Sciences.
Tripathi, G., Husain, A., Ahmad, S., Hasan, Z., & Farooqui, A. (2021). Contamination of water resources in industrial zones. In Contamination of Water (pp. 85–98). Academic Press.
Truong, Q., Ramezanianpour, M., Jensen, M., & Yuki, I. (2022). Treatment of septic tank discharge using crushed glass filter media. https://doi.org/10.20944/preprints202204.0180.v1
Tsitonaki, A., Petri, B., Crimi, M., Mosbaek, H. A. N. S., Siegrist, R. L., & Bjerg, P. L. (2010). In situ chemical oxidation of contaminated soil and groundwater using persulfate: A review. Critical Reviews in Environmental Science and Technology, 40 (1), 55–91.
Tuccillo, M. E. (2010). Report on condition assessment technology of wastewater collection systems . National Risk Management Research Laboratory, Office of Research and Development, US Environmental Protection Agency.
U.S. EPA (2010) Superfund remedy report (Thirteenth Edition), EPA-542-R-10–004, U.S. Environmental Protection Agency, Washington, DC, USA.
UNICEF., & World Health Organization. (2002). Children in the new millennium: environmental impact on health . UNEP/Earthprint.
Unicef. (2018). Drinking water, sanitation and hygiene in schools: Global baseline report 2018.
Vallero, D.A. (2019). Hazardous wastes—Chapter 31. In: Letcher, T.M., Vallero, D.A. (Eds.), Waste (pp. 585–630, 2nd ed). Academic Press.
Van Zyl, J., & Clayton, C. R. I. (2007). The effect of pressure on leakage in water distribution systems. In Proceedings of the Institution of Civil Engineers-Water Management (Vol. 160, No. 2, pp. 109–114). Thomas Telford Ltd.
Varghese, J., & Jaya, D. S. (2014). Metal pollution of groundwater in the vicinity of Valiathura sewage farm in Kerala, South India. Bulletin of Environmental Contamination and Toxicology, 93 , 694–698.
Vijay, R., Khobragade, P., & Mohapatra, P. K. (2011). Assessment of groundwater quality in Puri City, India: An impact of anthropogenic activities. Environmental Monitoring and Assessment, 177 (1), 409–418.
Wakode, H. B., Baier, K., Jha, R., & Azzam, R. (2018). Impact of urbanization on groundwater recharge and urban water balance for the city of Hyderabad, India. International Soil and Water Conservation Research, 6 (1), 51–62.
Wang, Q., Guo, S., Ali, M., Song, X., Tang, Z., Zhang, Z., ... & Luo, Y. (2022). Thermally enhanced bioremediation: A review of the fundamentals and applications in soil and groundwater remediation. Journal of Hazardous Materials , 433 , 128749.
Washington, E. (2004). DC: primer for municipal waste water treatment systems . Document no. EPA 832-R-04-001.
Watanabe, R., Harada, H., Yasui, H., Le, T. V., & Fujii, S. (2021). Exfiltration and infiltration effect on sewage flow and quality: A case study of Hue, Vietanam. Environmental Technology, 42 (11), 1747–1757.
Wolf, L., Ludewig, M., Hahne, L. M., & Kölbel, T. (2010, April). Environmental Compliant Planning of Shallow Geothermal District Heating Using Numerical Groundwater Models. In Proceedings World Geothermal Congress, Bali , Indonesia (pp. 25–29).
Wong, Y. J., Shimizu, Y., He, K., & Nik Sulaiman, N. M. (2020). Comparison among different ASEAN water quality indices for the assessment of the spatial variation of surface water quality in the Selangor river basin, Malaysia. Environmental Monitoring and Assessment, 192 , 1–16.
Wong, Y. J., Shimizu, Y., Kamiya, A., Maneechot, L., Bharambe, K. P., Fong, C. S., & Nik Sulaiman, N. M. (2021). Application of artificial intelligence methods for monsoonal river classification in Selangor river basin, Malaysia. Environmental Monitoring and Assessment, 193 (7), 438.
Wood, B. R. A., & Harris, R. W. (2000). Structural integrity and remnant life evaluation of pressure equipment from acoustic emission monitoring. International Journal of Pressure Vessels and Piping, 77 (2–3), 125–132.
World Health Organization. (2022). WHO consolidated guidelines on tuberculosis. Module 4: treatment-drug-resistant tuberculosis treatment, 2022 update . World Health Organization.
Yadav, D., Rangabhashiyam, S., Verma, P., Singh, P., Devi, P., Kumar, P., ... & Kumar, K. S. (2021). Environmental and health impacts of contaminants of emerging concerns: Recent treatment challenges and approaches. Chemosphere , 272 , 129492.
Yao, M., Yuan, Q., Qu, D., Liu, W., Zhao, Y., & Wang, M. (2022). Effects of airflow rate distribution and nitrobenzene removal in an aquifer with a low-permeability lens during surfactant-enhanced air sparging. Journal of Hazardous Materials, 437 , 129383.
Yates, M. V. (2011). On-site wastewater treatment. Encyclopedia of Environmental Health, 256 . https://doi.org/10.1016/B978-0-444-52272-6.00046-5
Yu, Y., Safari, A., Niu, X., Drinkwater, B., & Horoshenkov, K. V. (2021). Acoustic and ultrasonic techniques for defect detection and condition monitoring in water and sewerage pipes: A review. Applied Acoustics, 183 , 108282.
Yussof, N. A. M., & Ho, H. W. (2022). Review of water leak detection methods in smart building applications. Buildings, 12 (10), 1535.
Yusuf, W., Alaka, H., Ahmad, M., Ebenezer, W., Ajayi, S., Toriola-Coker, L. O., & Ahmed, A. (2022) Automatic surface encrustation detection in concrete sewers using Cctv inspection footage: A fusion of deep learning and big data. Available at SSRN 4331293 .
Zeynali, M. J., Pourreza-Bilondi, M., Akbarpour, A., Yazdi, J., & Zekri, S. (2022). Optimizing pump-and-treat method by considering important remediation objectives. Applied Water Science, 12 (12), 268.
Zubia, J. (1999). Principal Engineer, Brown and Caldwell, Seattle, WA, Interviews.
Download references
Author information
Authors and affiliations.
School of Civil Engineering, Vellore Institute of Technology, Vellore, 632014, Tamil Nadu, India
D. Sridhar & S. Parimalarenganayaki
You can also search for this author in PubMed Google Scholar
Contributions
Sridhar D contributed to the conception, design, and drafting of the manuscript. Parimalarenganayaki S contributed to revising the manuscript critically for important intellectual content. Both authors have approved the version of the manuscript to be published.
Corresponding author
Correspondence to S. Parimalarenganayaki .
Ethics declarations
Ethical approval.
The authors declare that they will follow the journal’s guidelines on the reliability of the scientific record.
Conflict of Interest
The authors declare no competing interests.
Additional information
Publisher's note.
Springer Nature remains neutral with regard to jurisdictional claims in published maps and institutional affiliations.
Rights and permissions
Springer Nature or its licensor (e.g. a society or other partner) holds exclusive rights to this article under a publishing agreement with the author(s) or other rightsholder(s); author self-archiving of the accepted manuscript version of this article is solely governed by the terms of such publishing agreement and applicable law.
Reprints and permissions
About this article
Sridhar, D., Parimalarenganayaki, S. A Comprehensive Review on Groundwater Contamination Due to Sewer Leakage: Sources, Detection Techniques, Health Impacts, Mitigation Methods. Water Air Soil Pollut 235 , 56 (2024). https://doi.org/10.1007/s11270-023-06852-1
Download citation
Received : 09 June 2023
Accepted : 13 December 2023
Published : 05 January 2024
DOI : https://doi.org/10.1007/s11270-023-06852-1
Share this article
Anyone you share the following link with will be able to read this content:
Sorry, a shareable link is not currently available for this article.
Provided by the Springer Nature SharedIt content-sharing initiative
- Sewer damages
- Sewage contaminants
- Sewer leak detection
- Groundwater treatment
- Exfiltration/infiltration
- Find a journal
- Publish with us
- Track your research

COMMENTS
In this paper, a review of the importance of groundwater, contamination and biological, physical as well as chemical remediation techniques have been discussed.
Groundwater contamination can impact human health, environmental quality, and socioeconomic development. For example, many studies have shown that high levels of fluoride, nitrate, metals, and persistent organic pollutants are a health risk for human populations (Wu et al. 2020).
The topics of these papers cover a range of contamination issues, including the sources of geogenic and anthropogenic contamination, seasonal cycles in contamination, human health risks, and remediation technologies.
This special issue provides a unique collection of papers which address some of the water quality challenges we face at a global scale. It covers opportunities that groundwater provides for a range of uses, and covers some treatment and management solutions for several key groundwater quality threats.
To prevent microbial contamination of groundwater, it is essential to site boreholes/wells in hygienic areas and uplands to minimize runoff pollution, ensure that wells/boreholes are well maintained, and avoid animals grazing around these water sources.
Groundwater contamination throughout the globe is a major challenge for the sustainability of ecosystems. Rapid industrialization, urbanization, and economic growth mediated huge contaminants generation are one of the major contributors to groundwater contamination.
Groundwater pollution is a worldwide problem with significant effects on human health and environmental security. It requires an in-depth understanding as the access to safe drinking water is a fundamental human right.
This Special Issue entitled “Groundwater Pollution: Sources, Mechanisms, and Prevention” aims to present new research contributions in the area of groundwater contamination, and will focus on the sources, effects, and exposure of natural and artificial groundwater pollutants, hydrological processes, and hydrochemical properties of ...
This paper delves into groundwater treatment methods, highlighting the difficulties in achieving optimal water quality and reveals that technologies such as Permeable Reactive Barrier and advanced oxidation processes exhibit potential in effectively removing trace-level pollutants.
Vicente Vergara-Flórez. José L. Marrugo-Negrete. The Morroa aquifer, which is an essential source of water supply in Sucre, Colombia, with more than 500,000 users, may be at risk because of...