Click through the PLOS taxonomy to find articles in your field.
For more information about PLOS Subject Areas, click here .

Clinical genetics
- Medicine and health sciences
- Chromosomal disorders
- Disorders of imprinting
- Gene therapy
- Genetic counseling
- Genetic diseases
- Genetic testing
- Personalized medicine
- Stem cell therapy
- X-linked traits
- Y-linked traits
- Get an email alert for Clinical genetics
- Get the RSS feed for Clinical genetics
Showing 1 - 13 of 3,182
View by: Cover Page List Articles
Sort by: Recent Popular
Decreased risk of underdosing with continuous infusion versus intermittent administration of cefotaxime in patients with sickle cell disease and acute chest syndrome
Keyvan Razazi, Enora Berti, [ ... ], Armand Mekontso Dessap
From code to care: Clinician and researcher perspectives on an optimal therapeutic web portal for acute myeloid leukemia
Terese Knoppers, Cassandra E. Haley, [ ... ], Ma’n H. Zawati
ATF4 ">miR-214 aggravates oxidative stress in thalassemic erythroid cells by targeting ATF4
Tipparat Penglong, Apisara Saensuwanna, [ ... ], Kanitta Srinoun
Acute care utilization among individuals with sickle cell disease and related cardiopulmonary and renal complications
Ashima Singh, David C. Brousseau, [ ... ], Amanda M. Brandow
Raman difference spectroscopy and U-Net convolutional neural network for molecular analysis of cutaneous neurofibroma
Levi Matthies, Hendrik Amir-Kabirian, [ ... ], Reinhard E. Friedrich
Investigation of HLA susceptibility alleles and genotypes with hematological disease among Chinese Han population
Ye-Mo Li, Yu-Xia Li, [ ... ], Zhong-Zheng Zheng
Qualitative assessment of knowledge, attitude and practice of oncologists about precision medicine in cancer patients- study from Lahore, Pakistan
Rida Naaem, Furqan Khurshid Hashmi, Sulaman Yaqub, Dzul Azri Mohamed Noor
Cystoseira /mesenchymal stem cells in immunosuppressed rats supported by overwhelming immuno-inflammatory crosstalk">Wound healing potential of Cystoseira /mesenchymal stem cells in immunosuppressed rats supported by overwhelming immuno-inflammatory crosstalk
Eman Maher Zahran, Reham H. Mohyeldin, [ ... ], Usama Ramadan Abdelmohsen
Deep learning workflow to support in-flight processing of digital aerial imagery for wildlife population surveys
Tsung-Wei Ke, Stella X. Yu, [ ... ], Timothy P. White
The role of community engagement in promoting research participants’ understanding of pharmacogenomic research results: Perspectives of stakeholders involved in HIV/AIDS research and treatment
Sylvia Nabukenya, David Kyaddondo, [ ... ], Erisa S. Mwaka
137 Cs-contaminated Aralia elata buds for the revival of local wild vegetable cultures">Exploring simple ways to avoid collecting highly 137 Cs-contaminated Aralia elata buds for the revival of local wild vegetable cultures
Masaru Sakai, Mirai Watanabe, [ ... ], Seiji Hayashi
The causal effect of iron status on risk of anxiety disorders: A two-sample Mendelian randomization study
Ruiying Yin, Qi Gao, Guangzhen Fu, Qiang Zhao
CRISPR-Cas9-guided amplification-free genomic diagnosis for familial hypercholesterolemia using nanopore sequencing
Sijia Xu, Hiroki Shiomi, [ ... ], Koh Ono
Connect with Us
- PLOS ONE on Twitter
- PLOS on Facebook
Clinical Genomics
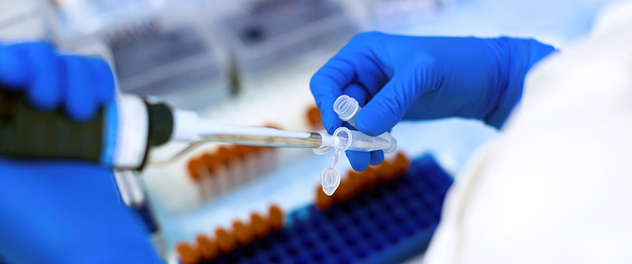
Backed by a team approach and a rich database of tissue samples and patient data, the Department of Clinical Genomics-Research is at the forefront of genomics-based research on everything from common forms of cancer to rare inherited disorders.
The Department of Clinical Genomics-Research at Mayo Clinic conducts research on a wide range of diseases and conditions that have a genetic basis, including common forms of cancer such as breast cancer, and rare and novel genetic disorders, such as lysosomal storage diseases.
The overarching goal of research within the Department of Clinical Genomics-Research is to further the scientific understanding of genetic-based diseases in order to help improve prevention, diagnosis and treatment for each patient.
Clinical genomics is truly a cross-specialty study. Genomics researchers work closely with their counterparts in many other research and clinical areas at Mayo Clinic, including the Department of Clinical Genomics , which includes experienced board-certified medical geneticists and certified genetic counselors who tailor care to each patient's needs.
Research being conducted in the Department of Clinical Genomics-Research also provides opportunities to participate in genetic research studies to improve overall patient care.
Research by our investigators — who are experts in medical genetics, neurology, pediatrics and other specialties — may eventually lead to better methods of screening, prevention and treatment for a wide array of genetic disorders. Our researchers are investigating breast cancer, ovarian cancer, colon cancer, congenital disorders of glycosylation, melanoma, lysosomal storage disease, neurofibromatosis, and rare or suspected genetic disorders.
- Read more about our research .
The research chair for the Department of Clinical Genomics-Research is physician-scientist David R. Deyle, M.D. , a medical geneticist at Mayo Clinic in Rochester, Minnesota, and an assistant professor of medical genetics at Mayo Clinic College of Medicine and Science. Dr. Deyle conducts research on improved methods of targeted genomic editing using viral vectors for the precise alteration of the human genome.
Working in a collaborative environment, we're striving to gain a better understanding of familial and congenital disorders. Contact us about research on breast cancer, ovarian cancer, colon cancer, lysosomal storage diseases, neurofibromatosis, schwannoma and other conditions.
Driven by genomics-focused research, we study breast cancer, ovarian cancer, colon cancer, congenital disorders of glycosylation, lysosomal storage disease, neurofibromatosis and schwannomas.
Meet the faculty of the Department of Clinical Genomics-Research at Mayo Clinic, and explore links to their publications and clinical trials.
- Clinical Trials
Mayo Clinic offers numerous clinical trials related to genetic and inherited conditions.
- Find genetic research studies - Clinical Trials Find genetic research studies
- Clinical Trials Clinical Trials
- Volunteering - Clinical Trials Volunteering
More about research at Mayo Clinic
- Research Faculty
- Laboratories
- Core Facilities
- Centers & Programs
- Departments & Divisions
- Institutional Review Board
- Postdoctoral Fellowships
- Training Grant Programs
- Publications
Mayo Clinic Footer
- Request Appointment
- About Mayo Clinic
- About This Site
Legal Conditions and Terms
- Terms and Conditions
- Privacy Policy
- Notice of Privacy Practices
- Notice of Nondiscrimination
- Manage Cookies
Advertising
Mayo Clinic is a nonprofit organization and proceeds from Web advertising help support our mission. Mayo Clinic does not endorse any of the third party products and services advertised.
- Advertising and sponsorship policy
- Advertising and sponsorship opportunities
Reprint Permissions
A single copy of these materials may be reprinted for noncommercial personal use only. "Mayo," "Mayo Clinic," "MayoClinic.org," "Mayo Clinic Healthy Living," and the triple-shield Mayo Clinic logo are trademarks of Mayo Foundation for Medical Education and Research.

An official website of the United States government
The .gov means it’s official. Federal government websites often end in .gov or .mil. Before sharing sensitive information, make sure you’re on a federal government site.
The site is secure. The https:// ensures that you are connecting to the official website and that any information you provide is encrypted and transmitted securely.
- Publications
- Account settings
Preview improvements coming to the PMC website in October 2024. Learn More or Try it out now .
- Advanced Search
- Journal List
- Portland Press Opt2Pay

Recent developments in genetic/genomic medicine
Rachel h. horton.
Clinical Ethics and Law, Faculty of Medicine, University of Southampton, Southampton, United Kingdom
Anneke M. Lucassen
Advances in genetic technology are having a major impact in the clinic, and mean that many perceptions of the role and scope of genetic testing are having to change. Genomic testing brings with it a greater opportunity for diagnosis, or predictions of future diagnoses, but also an increased chance of uncertain or unexpected findings, many of which may have impacts for multiple members of a person’s family. In the past, genetic testing was rarely able to provide rapid results, but the increasing speed and availability of genomic testing is changing this, meaning that genomic information is increasingly influencing decisions around patient care in the acute inpatient setting. The landscape of treatment options for genetic conditions is shifting, which has evolving implications for clinical discussions around previously untreatable disorders. Furthermore, the point of access to testing is changing with increasing provision direct to the consumer outside the formal healthcare setting. This review outlines the ways in which genetic medicine is developing in light of technological advances.
Introduction
The past two decades have seen major shifts in our technical ability to sequence genetic information at scale. Historically, genetic testing tended to consist of either highly detailed molecular testing of nominated single genes, or broad genome-wide dosage screening at low resolution, for example karyotyping [ 1 , 2 ]. Genome sequencing was too slow and too expensive to be used in clinical contexts: for example the Human Genome Project, which was 99% complete in 2004, cost three billion dollars and took 13 years to sequence [ 3 ].
More recently, advances in sequencing technology have made it possible to undertake broad genetic testing on an individual patient basis within a clinically useful timeframe, via exome and genome sequencing. Exome tests sequence the entire protein-coding region of the genome, representing less than 2% of the genome but containing approximately 85% of known disease-causing variants [ 4 ]; genome sequencing encompasses the exome but also sequences all the non-protein-coding DNA. Initially implementation of such tests was via clinical research studies such as the Deciphering Developmental Disorders project [ 5 ], but more recently exome sequencing has been utilised as a clinical diagnostic test [ 6 ]. Genome sequencing is also due to transition to being available as a standard NHS test in June 2019, having previously only been available via initiatives such as the 100,000 Genomes Project [ 7 ].
Sequencing technology has improved in depth as well as breadth, and this has been of importance in better understanding cancer. The ability to sequence cancer genomes has led to rapid identification of driver mutations and has helped to work out the complex relationships between different cancer subclones over space and time, demonstrating the enormous heterogeneity of cancers and the difficulty of successfully treating them [ 8 ]. As sequencing techniques have advanced to the level where tiny amounts of tumour or individual cells can be sequenced, it has been possible to identify previously unknown mutational mechanisms, such as chromothripsis 1 [ 9 ] and kataegis 2 [ 10 ].
However, our ability to generate genomic data has substantially outstripped our ability to interpret its significance for an individual, and while improvements in genomic technology are in many cases driving improvements in healthcare, we are also encountering new problems as genomic testing shifts into the clinical setting. The Global Alliance for Genomics and Health (GA4GH) predicts that by 2025, over 60 million people will have had their genome sequenced in a healthcare context [ 11 ], but pathways for managing the output from genome sequencing are still in their infancy. The detailed but unfocused approach of genomic tests gives opportunities to answer questions that go beyond the problems that led to a patient having a test. However, deciding which of the multitude of possible outputs from genomic tests should be considered a ‘result’ at any given time is very challenging, not least because the links between many genetic variants and diseases are often unproven or poorly understood [ 12 ]. Multidisciplinary input and collaboration are increasingly key to interpreting the significance of genomic results. This review discusses the developments in practice that are evolving as a result of increasing use of genomic technologies.
New disease gene discovery and changing concepts of diagnosis
Exome and genome sequencing are powerful diagnostic tools – for example the Deciphering Developmental Disorders project, which recruited patients with severe undiagnosed disorders (who had generally already had any currently available diagnostic genetic testing), achieved a 40% diagnosis rate via trio exome sequencing for the first 1133 family trios in the study [ 13 ]. The search for a diagnosis has often been described as a journey [ 14 ], with parents of children with rare genetic disorders anticipating that a diagnosis may guide treatment, prognosis, acceptance and social support [ 15 ]. However, identification of new rare disease genes may be changing the impact of receiving a diagnosis, and in many cases very little is known about the long-term effects of newly identified genetic conditions.
Historically when making a genetic diagnosis, it has usually been possible to give families some information regarding prognosis, and to provide some parameters as to what to expect for the future, based on previous experience of what has happened for other children affected by the same condition. Now, while in some situations due to strong phenotypic match it is possible to be confident that a child’s rare disease has been caused by pathogenic variants in a recently described rare disease gene, often this provides little information about a child’s future.
We are increasingly in the position of learning about the effects of possible disease-causing variation(s) in a gene through meeting the patients in whom such genetic changes have been discovered. Often these changes will be in a gene newly thought to be linked to developmental disorders and there will be little, if any, published literature to draw on. We then have to speculate whether the genetic change detected is the cause of our patient’s health problems, and whether any additional difficulties that have happened for our patient that have not yet been noted in other patients with changes in the same gene are an extension of the phenotype of the newly described disease gene, or coincidental. In situations like this, we are often unable to give people information about what a new diagnosis might mean for them or their child in the longer term.
This has led to patient support and awareness groups taking on an increasingly important role [ 16 ], as families gather to share their lived experience of newly diagnosed rare genetic conditions, in turn informing clinical services. For example, the charity Unique works with families and professionals to develop specialist information relating to many rare and newly described genetic conditions, and to gather information about their long-term effects, increasing awareness and understanding of what it is like to live with rare genetic conditions. The rapidity with which such information can be gathered is also exemplified by the work of the PURA Syndrome Foundation: in 2014 the first patients with a rare condition called PURA syndrome were described in the medical literature [ 17 ]. Shortly afterwards the PURA Syndrome Foundation was established which has catalysed links between families, clinicians and researchers, greatly improving the speed and quality of research into the condition [ 18 ].
The agnostic approach of exome and genome sequencing is also challenging our previous concepts of existing genetic diagnoses, when apparently pathogenic variants are found in well-described disease genes but the patient’s clinical picture falls outside the boundaries of what we would conventionally expect for a patient affected by that particular genetic condition. For example, loss-of-function variants in SOX2 are known to cause anophthalmia and microphthalmia in addition to other phenotypes such as developmental delay and structural brain anomalies. Eye abnormalities were thought to be a key feature of SOX2 -related disorders, and so SOX2 would only be requested as a genetic test in patients who had absent or small eyes. Recently, via ‘genotype-first’ approaches, loss-of-function SOX2 variants have been found in people with developmental delay but without anophthalmia or microphthalmia, broadening the phenotypic spectrum associated with this gene [ 19 ]. Case Study 1 shows a further example where exome testing has extended previous perceptions of the clinical scope of a genetic condition.
Case Study 1
Redefining our understanding of genetic conditions (fictional case based on eggens et al. [ 20 ]).
An 8-year-old girl was referred to clinical genetics in order to investigate her progressive weakness. She had been floppy as a baby and from the age of 5 years had developed worsening limb weakness with frequent unusual movements, and difficulty in swallowing. Serial brain scans had shown progressive cerebellar atrophy.
Exome testing found that she was homozygous for a variant predicted to disrupt the function of EXOSC3 , a gene associated with pontocerebellar hypoplasia. This diagnosis had never been thought of as she did not have one of the defining characteristics: pontine hypoplasia. Her clinical picture also seemed atypical for this condition – most children with pontocerebellar hypoplasia do not survive infancy.
However, recent research has shown genotype–phenotype correlations in EXOSC3 -mediated pontocerebellar hypoplasia – patients homozygous for p.D132A variants (like this patient had) tend to have a milder clinical course and preservation of the pons. This genetic explanation fitted well in retrospect, but would not have been considered in advance of the exome test.
Key messages
- Many well-recognised genetic conditions may have a wider spectrum of effects than previously thought.
- Patients with genetic conditions identified via genomic tests may not conform to the pattern we expect based on experience of patients with the same condition identified via single gene testing. It can be very difficult to be sure whether this reflects an incorrect diagnosis, or a wider disease spectrum than previously recognised.
In many cases, our understanding of why the same genetic condition may be expressed so differently among different people is at an early stage, and this often makes genetic counselling very challenging, particularly in the prenatal setting. For some genetic conditions, it is becoming possible to provide more personalised risk estimates, based on combining knowledge of a person’s genetic diagnosis, with analysis of other factors that may influence their risk. Personalisation of risk in this way has generally been crude and reliant on clinically obvious characteristics: for example, men with pathogenic BRCA variants have a lower risk of developing breast cancer than women with pathogenic BRCA variants. More recently, genetic testing is being developed to complement ‘key’ genetic test results to provide an increasingly refined personal risk. For example, use of a polygenic risk score using breast cancer and ovarian cancer susceptibility SNPs identified via population GWAS showed large differences in absolute cancer risks between women with pathogenic BRCA variants with higher compared with lower polygenic risk score values [ 21 ]. This has yet to translate into routine clinical practice, but has the potential to help women with pathogenic BRCA variants make more informed decisions about how and when to manage their cancer risk.
The downsides of improved sensitivity: increased uncertainty in what tests mean
The prior probability of any one variant identified via genome sequencing being causative for a patient’s rare disease is extremely low. Attempts to catalogue human genetic variation, for example via the 1000 Genomes Project, show that a typical human genome differs from the reference human genome at 4.1–5 million sites [ 22 ]. Most of these variations will be entirely benign, some may subtly impact on risk of various common diseases, and a very small number will have the potential to cause serious disease either in an individual, or in their children (potentially in combination with variants inherited from their partner).
Genome sequencing identifies the majority of these variants, which then need careful filtering to produce a meaningful output. This has required a significant change in mindset from an era when most variants were identified in the context of carefully chosen single gene sequencing, and so had a much higher prior probability of being causative. There is an increasing shift towards a view that variants should be ‘innocent until proven guilty’ [ 23 ], but there is a lack of consensus regarding how to translate this principle into clinical practice.
There is also considerable discrepancy in how different genetics laboratories interpret the same variants. International guidelines for variant interpretation are helpful but insufficient to remove a great deal of noise when attempting to assign significance to particular findings [ 24 ]. This was illustrated in a recent study comparing variant classification among nine genetic laboratories: although they all used the same guidelines, only 34% of variants were given the same classification by all laboratories, and 22% of variants were classified so differently that different medical interventions would be recommended [ 25 ]. At a lower resolution level, even being sure of the relationship between genes and diseases is often difficult. For example, curation of the 21 genes routinely available on Brugada syndrome gene panels using the ClinGen gene curation scoring matrix found that only one of these genes was definitively linked to Brugada syndrome [ 26 ]. Our improving knowledge of variant interpretation leaves us with a difficult legacy, with many patients having been diagnosed incorrectly with genetic conditions. The effects of this can be far-reaching and difficult to undo, as illustrated by Case Study 2 .
Case Study 2
The legacy of incorrect diagnosis (case reported by ackerman et al. [ 27 ]).
A teenage boy died suddenly and genetic testing was then undertaken for his brother, resulting in the finding of a rare variant in KCNQ1 . On the basis of this test, the living brother was diagnosed with long QT syndrome, and the teenage boy’s sudden death was attributed to long QT syndrome. The living brother had an implantable cardioverter defibrillator inserted, and via cascade genetic testing over 24 relatives were diagnosed as having long QT syndrome, despite having normal QT intervals on ECG.
However, subsequent examination of post-mortem samples found that the boy who died had cardiac features inconsistent with long QT syndrome, did not have the KCNQ1 variant found in the wider family, and instead had a clearly disease-causing de novo variant in DES , a gene linked to cardiomyopathy.
- It is very important to consider whether the clinical picture fits when evaluating variant significance: genetic variants will usually only predict disease well if found in the context of a medical or family history of the relevant disease.
- Incorrect (or inappropriately deterministic) genetic test interpretation can affect the clinical care of a whole family, not just the person being tested.
Although this suggests that we need to be very cautious in making firm genetic diagnoses, it is difficult to know where the threshold should lie for communicating genetic variation of uncertain significance. There is some evidence that people find receiving a variant of uncertain significance surprising and disturbing, and some people misinterpret it as being definitely pathogenic or definitely benign [ 28 ]. However, there is also evidence that many people have a strong desire to receive a broad range of results from genetic testing, including uncertain results, and are uncomfortable with the idea that decisions about non-disclosure might be made without involving them [ 29 ].
The fear is that disclosure of uncertain variants will lead to over-diagnosis and over-management, with variants inappropriately being treated as if pathogenic. Excessive and inappropriate interventions (not to mention anxiety and distress) might then cascade through families, going against one of the fundamental principles of medicine to ‘first do no harm’. However, we also fear missing something or being accused of ‘hiding information’. The result is that we tend to end up in purgatory, documenting uncertain variants on lab reports (though sometimes not) and having lengthy conservations with patients about them (though sometimes not), then tacking on a caveat that ‘maybe this means nothing’. This nominally shifts the responsibility to the next person in the chain but feels unsatisfactory for all concerned.
Uncertainty when to stop looking and what to communicate
Another issue arising from improved sensitivity is the ability to find genetic variants that are unrelated to the clinical problem that a patient presents with, but that may be relevant for their health in other ways. This may be viewed as positive or negative, but working out how to handle this information raises difficult questions. In 2013, the American College of Medical Genetics and Genomics (ACMG) suggested that laboratories should automatically seek and report pathogenic variants in 56 genes associated with ‘medically actionable’ conditions when performing clinical sequencing [ 30 ]. The main rationale was the potential to benefit patients and families by diagnosing disorders where preventative measures and/or treatments were available, with the aim of improving health. However, these recommendations proved controversial. The main debate at the time centred around whether patients should have a right to choose not to know such information [ 31 ]. Subsequent questions about the role of clinicians in offering additional findings, what constitutes a ‘medically actionable’ finding, and what is the predictive value of such findings in the absence of a phenotype or family history of the relevant disorder, are yet to be fully addressed.
Analysis of data from the 1000 Genomes cohort demonstrated that approximately 1% of ‘healthy’ people will have a ‘medically actionable’ finding in one of the 56 genes [ 32 ]. However, what this might mean on an individual basis is often unclear. Most of our knowledge regarding the effects of variation in any given gene has been gathered by observing people who have been identified as having variants in the gene because they were tested as they had a personal history or family history of disease, biasing the sample from which our conclusions are drawn. It is less clear what it might mean to find, for example, an apparently pathogenic variant in a gene linked to cardiomyopathy in a person with no personal or family history of heart problems. This has important implications for ‘cascade screening’, where relatives of a patient affected by a condition with a known genetic cause are offered testing to see whether they have the disease-causing genetic variant that was found in their clinically affected family member (meaning that they may also be at risk of developing the disease). To what extent should testing and subsequent screening be offered in a family based on an incidental finding of a genetic variant thought to be predictive of a particular condition, if there is no clinical evidence that anyone in the family, including the person in whom the genetic variant in question was first identified, is actually affected by it?
Broad genomic testing also has the potential to detect carrier status for recessive and X-linked conditions. From population studies, we know that being a carrier for a genetic condition is very common. For example, a gene panel testing carrier status for 108 recessive disorders in 23453 people found that 24% were carriers for at least one of the 108 disorders, and 5.2% were carriers for multiple disorders [ 33 ]. On a disorder-by-disorder status, being a carrier for a genetic condition is very rare (with notable exceptions such as haemochromatosis and cystic fibrosis), but when considered collectively, it is ‘normal’ to be a carrier for a genetic condition. For most people, being a carrier will have no impact on their life at all. However, if their partner happens to be a carrier for the same condition then the implications could be very profound, as each of their children would have a one in four chance of being affected by the genetic condition. This is particularly relevant for couples who are known to be biologically related [ 34 ], and couples with common ancestry, as they will have a higher chance of both being carriers for the same recessive condition. Carrier screening for various autosomal recessive diseases has been available in some instances for many years, for example screening for carrier status for Tay–Sachs disease for people of Ashkenazi Jewish ancestry has been offered since the 1970s [ 35 , 36 ]. More recently, advances in technology have led to development of expanded carrier screening tests, which check carrier status for multiple diseases simultaneously and are often less targeted towards particular genetic populations [ 37 ].
The increased scope of carrier screening, combined with the recognition that it is very common to be a carrier for one or more recessive genetic conditions, has led to an increasing move to consider carrier results for recessive genetic conditions on a couple basis, where carrier status is only communicated if it would be relevant in the context of a particular relationship (i.e. if both people in a couple are carriers for the same condition) [ 38 ]. This avoids pathologising the status of ‘being a carrier’, recognising that most of us are carriers for some genetic conditions, and conserves resources for genetics services by not flooding the system with large volumes of individual carrier results, most of which will be meaningless in the context of that individual’s life. Objections to this approach are that by not communicating individual carrier results, a person would not know this information for future relationships, and their family could not access cascade screening to see whether they are also carriers. However, these objections could be obviated by widespread adoption of couple carrier testing – a person (or their close relatives) could find out their carrier status if relevant when they next had a couple carrier test in the context of their new relationship. In some ways, this could be seen as comparable with management of infectious disease – lots of healthy people carry MRSA, but very few die of MRSA infection. People are therefore screened at times when they might be especially vulnerable to becoming unwell from MRSA, or when they might pass it on to others at risk, for example when admitted to hospital, rather than being tested at random points when they are generally well.
The expanding remit and availability of genetic technology
‘acute genetics’.
For many years, clinical genetics input has at times influenced acute care, for example in diagnosing trisomies in the neonatal period, or informing the care of babies born with ambiguous genitalia. However in many circumstances, the key contribution of clinical genetics was in providing a post hoc explanation for serious medical problems, rather than in influencing treatment decisions on a real-time basis. This is changing as the availability of exome and genome sequencing increases, as shown by Case Study 3 . A recent study in a neonatal intensive care unit in Texas studied outcomes for 278 infants who were referred for clinical exome sequencing, and found that 36.7% received a genetic diagnosis, and medical management was affected for 52% of infants with diagnoses [ 39 ]. There is increasing evidence that this approach is cost-effective: for example, a prospective study of exome sequencing for infants with suspected monogenic disorders found that standard care achieved an average cost per diagnosis of AU$ 27050, compared with AU$ 5047 for early singleton exome sequencing [ 40 ]. Similarly, ‘real-time’ genetic and genomic testing is making an impact in cancer treatment, where in many cases testing is available to help guide treatment choices by identifying actionable genetic variants in tumours that may respond to specific therapies [ 41 , 42 ].
Case Study 3
Insights from exome testing transforming a clinical course (case from wessex genomic medicine centre [ 43 ]).
A young woman was referred for exome testing having spent months in a coma. From childhood she had experienced sensory problems, and as a young adult she had gone on to develop seizures which deteriorated into status epilepticus, necessitating ventilation on intensive care.
After 3 years during which all other avenues had been explored, analysis of her exome was proposed. An unexpected diagnosis of pyridoxine-dependent epilepsy was found; this had not previously been considered as classically it causes seizures in the first few months of life. She began treatment with pyridoxine (vitamin B 6 ). From that point on she had no further seizures and her clinical situation transformed. Over a 6-month period she was weaned off all of her anti-epileptic drugs, and was able to return to a normal life.
Key message
- Exome or genome tests have the potential to make an enormous difference to clinical care and to people’s lives.
Pharmacogenomics
As well as guiding treatment choice, genetic testing will increasingly influence what doses are prescribed, and whether medications are considered unsuitable in view of a high risk of an adverse reaction. Around the time that the Human Genome Project was completed, there was considerable excitement about the possibility of genetic testing guiding use of medication in the clinic [ 44 , 45 ]. The potential of genotype-driven drug dosing has for the most part yet to be realised, in part because the interaction of the genetic factors involved is sometimes complex, and in part because environmental factors may also have a significant impact on how a person responds to a drug. For example, genotype-driven prescription of warfarin, which has notoriously wide inter-individual variation in dosage requirements, largely remains in the realm of research [ 46 ].
However, for some drugs, pharmacogenomics has already had a significant impact in reducing morbidity and mortality. For example, when the antiretroviral drug abacavir was first introduced, approximately 5% of the people treated developed an idiosyncratic hypersensitivity reaction that could be life-threatening on repeated exposure to the drug [ 47 , 48 ]. Research established that immunologically confirmed hypersensitivity reactions to abacavir only occurred in people with the HLA-B*5701 allele, and a clinical trial went on to show that pre-screening patients to check that they did not have HLA-B*5701 prior to starting the drug led to no confirmed hypersensitivity reactions in the pre-screened arm, while 2.4% of the unscreened patients had reactions [ 49 ]. Patients are now screened for HLA-B*5701 as standard before starting abacavir treatment [ 50 ]. Similar screening is likely to become more widespread as we learn more about genetic risk factors for adverse drug reactions. For example, there are increasing suggestions that the mitochondrial variant m.1555A>G should be checked in patients with cystic fibrosis in order to guide antibiotic treatment choices, in view of the evidence that people with this variant may develop hearing loss when exposed to aminoglycosides [ 51 ].
Evolving options in prenatal genetics
Genetic testing is also being used more extensively in the prenatal setting, in part because of developments in non-invasive prenatal testing and diagnosis, which allow genetic screening or testing of a developing pregnancy by doing a blood test for the mother [ 52 ]. This removes the risk of miscarriage associated with conventional prenatal tests (chorionic villus sampling or amniocentesis). While this is in some ways a stride forward, it raises various ethical issues, as the technical test safety may lead to such testing becoming viewed as routine. This raises the concern that couples will give less careful consideration as to whether they really want to know the results before having such tests, and that women may feel that there is an expectation that they should have testing. The worry is that this could potentially lead to people feeling under pressure to terminate pregnancies in response to genetic test results (including in situations where the clinical implications of the results may be far from clear) [ 53 ].
Widening access to genetic testing within healthcare
The expanding options for genetic testing and the escalating expectation for quick results to drive clinical management mean that testing provision is increasingly being pushed out of highly specialised genetics centres into mainstream medicine. For example, many women with ovarian cancer will now be offered BRCA testing via their oncology team, and only referred to genetics if needed based on the test results [ 54 ]. Genetics appointments now frequently focus on interpretation of tests already done, working out if the test outcome seems to match the clinical problem, and arranging testing and surveillance for family members.
The rise of direct-to-consumer genetic testing
As clinical services have increasingly grown to expect and demand genetic answers for patients with complex health problems, on a broader societal level the hunger for genetic information also seems to be increasing. However this is occurring in the context of a public discourse about personalised/precision medicine and genetics that tend to enthusiastically promote it in a very optimistic light, rarely dwelling on potential concerns and limitations, and therefore potentially sculpting inappropriate expectations from technology that is still being developed [ 55 ].
Direct-to-consumer tests currently sit outside much of the regulation that governs clinical genetic testing, but claim to provide insight into issues as diverse as ancestry, nutrition, athletic ability, and child talent [ 56 ]. Many testing providers also claim to help provide insight on health, though the information provided by many direct-to-consumer companies is far from comprehensive. For example, a recent analysis of 15 direct-to-consumer genetic testing companies advertising to U.K. consumers found that none of them complied with all the U.K. Human Genetics Commission principles for good practice regarding consumer information [ 57 ]. There are also examples that might make us reflect sceptically on the value of these tests – for example a case where a family sent a sample from their dog to a direct-to-consumer testing company designed to provide insights on people’s genetic ‘superpowers’ and received a report which did not mention that the sample was not human but conjectured that the client would be talented at basketball [ 58 ].
‘DIY genetics’ has also risen in popularity, with people asking for raw data from direct-to-consumer companies then processing this themselves via third-party interpretation services, as discussed in Case Study 4 . Approximately 40% of genetic changes in direct-to-consumer test raw data sent for clinical confirmation are false positives [ 59 ], but this is often not appreciated by customers or the doctors they may subsequently visit, leading to anxiety and often inappropriate medical interventions [ 60 ]. However, clearly many people see a value in receiving genetic information and are prepared to pay for this. This marks a shift from genetic testing in order to explain health problems or for people at high risk of developing specific genetic conditions, to testing of healthy people with the rationale of facilitating life planning. This idea has been taken to the extreme with initiatives such as the BabySeq project, exploring the medical, behavioural and economic impacts of integrating genome sequencing into the care of healthy newborns [ 61 ].
Case Study 4
Grime on the crystal ball (fictional case based on moscarello et al. [ 60 ]).
A healthy medical student was given a direct-to-consumer genetic test for Christmas, and explored the raw data from this test using an online interpretation programme, finding a variant in MYBPC3 that was predicted to cause hypertrophic cardiomyopathy. He was understandably worried by this result, taking time off university as he came to terms with it, and giving up running, which he used to really enjoy.
He was seen in a hypertrophic cardiomyopathy clinic and had an expert cardiology assessment including ECG, echocardiogram and review of his family history. He was found to have no clinical evidence of hypertrophic cardiomyopathy, and further genetic testing showed that he did not actually have the disease-causing MYBPC3 variant that the online interpretation programme had identified. However, he continued to feel anxious about his risk of heart problems and decided to give up running permanently.
- Information provided from direct-to-consumer testing may be unreliable, especially where online interpretation programmes are used to further explore the raw data from the test: the level of quality control may be very different from that of accredited genetic laboratories, increasing the likelihood of false positives, false negatives and sample mix-up.
- Many direct-to-consumer genetic tests involve no meaningful pre-test counselling – people are often totally unprepared for the information that might come out of such testing (and are unaware that it might be wrong).
Genetic information as family information
The familial nature of genetic information has always generated discussion as to how to respect the confidentiality of individual patients while ensuring that their close relatives have access to information that may be relevant for their own health and life choices. Clinical guidance in this area has increasingly taken the stance that genetic information should be confidential to families, not individuals (though the personal consequences of having a genetic change for a given individual should be confidential to them alone) [ 62 ].
The consequences of this shift are still being navigated in the clinical setting – research indicates that patients often see genetic information as belonging to their family rather than exclusively to them [ 63 ], but healthcare professionals are often reticent about taking a familial approach to the confidentiality of genetic information in practice, worrying that this stance could disrupt family dynamics or erode patient trust in the health service [ 64 ]. A recent BMJ poll which asked, ‘Are there situations when sharing a patient’s genetic information with relatives without consent is acceptable?’ demonstrated the current split in opinion, with 51% of respondents answering ‘yes’ and 49% ‘no’ [ 65 ]. The personal versus familial nature of genetic information is currently being tested in the courts via the ABC case, which centres around non-disclosure of genetic risk to the daughter of a patient with Huntington’s disease [ 66 ].
Treatment for genetic disorders
One of the most exciting recent developments in genetics and genomics is the prospect of treatment for an increasing number of genetic conditions. However this topic has to be treated with caution as the practical reality for many patients and families is that though promising research is ongoing, meaningful treatment is not possible in many cases. Even in situations where evidence-based treatments have been developed, the expense of many of these therapies risks making them inaccessible.
Many different approaches have been taken to try to treat genetic conditions. Gene therapy, which involves delivering functional genetic code, is one approach but its success has been widely variable, often due to difficulty in developing vectors that can deliver genetic material into affected tissues at sufficiently high levels without being destroyed by the immune system. In certain situations this approach can be highly effective, for example promising results have been achieved in various eye conditions, likely because eyes are small and easily accessible, and have a privileged relationship with the immune system [ 67 ]. In cases aiming to deliver gene therapy to a wider area, such as the lungs or the muscles, treatment attempts have generally proved more challenging [ 68 , 69 ].
Other approaches include use of small molecules to modify various steps in the pathway from gene to functional product. For example, Eteplirsen aims to treat Duchenne muscular dystrophy in certain patients by influencing splicing machinery to skip exon 51 from mature DMD mRNA, restoring a more functional reading frame so that a shortened version of dystrophin can be successfully translated [ 70 ]. Ivacaftor potentiates the action of CFTR channels in some patients with cystic fibrosis (G551D pathogenic variant) [ 71 ]. Enzyme replacement therapy is being trialled to treat children with mucopolysaccharidoses, for example idursulphase infusions in mucopolysaccharidosis type 2 [ 72 ].
While lots of these therapies are very exciting and show demonstrable changes at the molecular level in clinical trials, these cellular changes do not always clearly translate into improvements in clinically relevant outcomes. The therapies are also often hugely expensive, which raises very difficult ethical questions regarding whether limited resources should be spent on such treatments where there is often only limited proof of clinical efficacy.
However the increasing possibility of future treatments for genetic conditions is influencing clinical decisions around the care of very ill children. For example, recently nusinersen has shown promise as a treatment for some children with spinal muscular atrophy, but this may begin to raise new questions about whether interventions such as intubation and tracheostomy should be offered to infants with severe spinal muscular atrophy, where previously these would have been considered medically inappropriate [ 73 ]. This has consequences for the clinical conversations happening when these diagnoses are made. In the past, breaking news of such a diagnosis might flow naturally into discussions around palliation. The possibility of treatment now creates new options to consider, but also new challenges in considering with parents how best to care for their child [ 74 ]. The clinical impact and accessibility of emerging treatments is often very uncertain, but parents may prefer to explore even extremely long-shot treatments over accepting a palliative care pathway route, and may expect or seek crowd funding for experimental treatments for which there is as yet very little, if any, evidence of benefit.
Improving genetic technology has also had a significant impact on fertility services, ranging from pre-implantation genetic diagnosis to mitochondrial donation, offering new options for families affected by genetic conditions [ 75 , 76 ]. Increasing technological capability is set to extend the theoretically possible range of options – for example last year a group in China used the CRISPR/Cas9 system to correct pathogenic variants in the HBB and G6PD genes in human zygotes [ 77 ], though the efficiency and accuracy of the correction procedure was variable. This emerging possibility raises significant ethical issues which need debate. A recent report of the Nuffield Council on Bioethics on genome editing in the context of human reproduction suggested that there may be certain contexts in which this may be ethically acceptable, provided that such interventions were intended to secure the welfare of a person who may be born as a result, and that any such interventions would uphold principles of social justice and solidarity [ 78 ].
Conclusions
Insights from genomic technology have great potential to improve health, but we are currently going through a teething process in learning how to respond to the nebulous information that genomic tests can provide in the clinical setting. In part, this learning process is being driven by patients and families, with patient support groups coming to the fore in an era where we can now make extremely rare diagnoses that link different families across the world, but often have very little information on what this might mean for the future. Our current response to the outcomes from genomic tests is often reactive and ad hoc, partly because we are still learning how to interpret genomic variation and are often unable to gain a consensus on whether genetic variants are clinically significant or not. This situation is exacerbated by the different routes in which genomic information is now accessible – rapid tests to establish diagnosis or plan treatment for patients are now a reality in the real-life clinical setting, but healthy people also have increasing access to commercial tests that claim to provide genetic information to improve health and life planning. This raises particular challenges in the context of a public discourse about genomics that tends to present it as far more predictive and certain than it actually is. Some of the most exciting recent developments in genomic medicine relate to potential future treatments and reproductive options for people and families affected by rare genetic conditions. However hurdles relating to treatment efficacy and optimal timing of treatment, mean that we need to keep these advances in perspective and consider how to research potential treatments responsibly, avoiding creating hype that undermines the ability of families to make a balanced decision whether or not to participate in this research. It is also important to consider financial sustainability, avoiding situations where useful new treatments are developed that remain inaccessible to the patients who need them on account of their cost. To summarise, the introduction of genomic testing is having a big impact on patient care, but raises various issues that need further study and debate in order to help us maximise the potential benefits of genomic medicine while minimising the possible harms.
Acknowledgments
We thank the patient in Case Study 3 for her help with the Case Study box and for sharing her story.
Abbreviations
1 Complex chromosome rearrangements, thought to occur due to single catastrophic events where chromosomes ‘shatter’ and are repaired by error-prone mechanisms.
2 Clusters of localised mutations.
This work was supported by funding from a Wellcome Trust collaborative award [grant number 208053/Z/17/Z (to A.L.)].
Competing interests
The authors declare that there are no competing interests associated with the manuscript.
- Introduction to Genomics
- Educational Resources
- Policy Issues in Genomics
- The Human Genome Project
- Funding Opportunities
- Funded Programs & Projects
- Division and Program Directors
- Scientific Program Analysts
- Contact by Research Area
- News & Events
- Research Areas
- Research investigators
- Research Projects
Clinical Research
- Data Tools & Resources
- Genomics & Medicine
- Family Health History
- For Patients & Families
- For Health Professionals
- Jobs at NHGRI
- Training at NHGRI
- Funding for Research Training
- Professional Development Programs
- NHGRI Culture
- Social Media
- Broadcast Media
- Image Gallery
- Press Resources
- Organization
- NHGRI Director
- Mission & Vision
- Policies & Guidance
- Institute Advisors
- Strategic Vision
- Leadership Initiatives
- Diversity, Equity, and Inclusion
- Partner with NHGRI
- Staff Search
Equipped with the power of genomic information and state-of-the art resources available at the National Institutes of Health (NIH), clinical researchers at the National Human Genome Research Institute (NHGRI) are leading a new era in medicine - one where a more profound understanding of the biological basis of disease will pave the way for more effective ways to diagnose, treat and prevent illness.
NHGRI clinical research and field work also spans a wide spectrum of populations, from Colombians living in the Andes Mountains, to Old Order Amish in Lancaster County, Pennsylvania, to two different populations of Hermansky-Pudlak Syndrome patients in Puerto Rico. NHGRI researchers are engaged in collaborations with other institutions that are conducting field studies in West Africa and in Finland in the quest to determine the genetic risk factors for adult-onset, Type II diabetes.
Analyzing data gathered in family studies, NHGRI clinical researchers have played key roles in a number of important gene discoveries, including the identification of genes responsible for holoprosencephaly, for a variety of periodic fevers, for Gray Platelet Syndrome and four types of Hermansky-Pudlak Syndrome, for Proteus syndrome, Arterial Calcification due to Deficiency of CD-73 (ACDC), and for a new form of methylmalonic acidemia.
However, NHGRI's clinical research endeavors extend far beyond searches for disease genes. researchers are studying knock-in mouse with hereditary inclusion body myopathy, developing gene therapy approaches for X-linked severe combined immunodeficiency, conducting clinical trials, conduct of clinical trials of a drug to combat mitochondrial disease, determine the safety and effecacy of drugs, and pursuing therapies to prevent progression of muscle wasting and weakness, among many other avenues.
Another vital component of clinical research at NHGRI are studies aimed at examining the psychosocial, ethical and policy implications of genetics research. Research projects currently underway in this area include an empirical study to determine what patients understand about the storage and use of DNA for future research, investigations into how patients respond to receiving state-of-the-art genetic information, and follow up analysis on the impact of predictive testing for the inherited colon cancer genetic syndrome, hereditary non-polyposis colon cancer or HNPCC.
NHGRI remains strongly committed to the future growth and development of the intramural clinical research program. The NHGRI clinical program also looks forward to increased opportunities for cooperative clinical projects and the exchange of ideas between NHGRI investigators and the rest of the NIH research community.
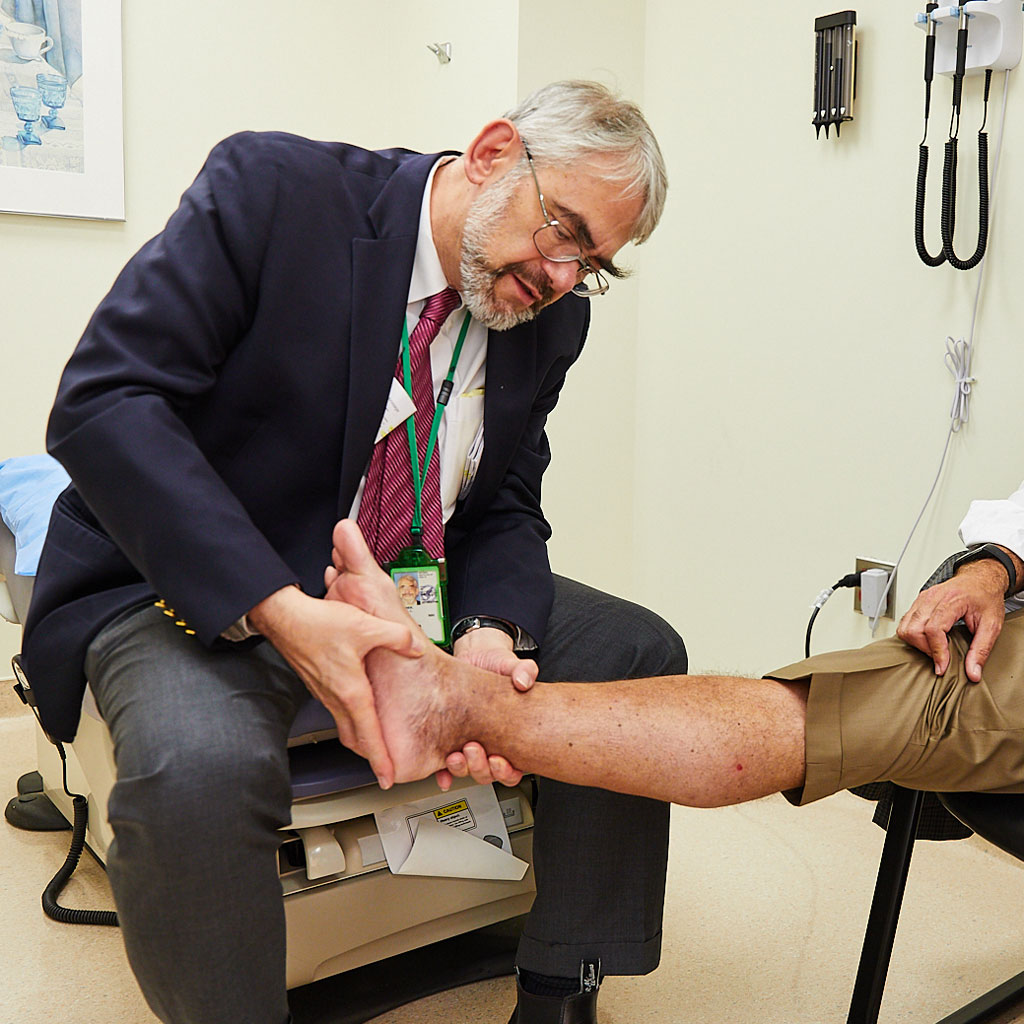
Current Clinical Studies
NHGRI researchers are working with patients and families to better understand of how genes can cause or influence diseases and develop new and more effective diagnostics and treatments.
Related Content
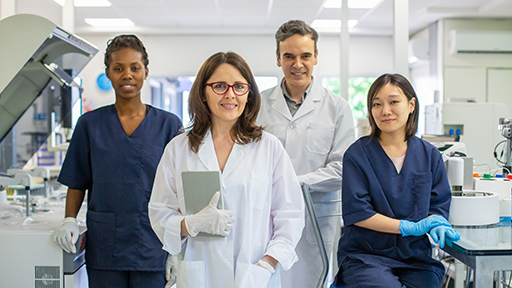
Last updated: November 2, 2021
- Working Groups
- Faculty Jobs
- Postdoc & Postgrad jobs
- Graduate Program
- Fellowship in Laboratory Genetics and Genomics (LGG)
- Clinical Genetics and Genomics Residency Training Program
- Pathway Programs
- Research Labs
- Office for Strategic Research Development
- Spatial Technology
- Bioinformatics
- Project Submission
- Service Fees
- Centers & Affiliations
- Participate in Our Research
- Post-Doctoral
- Junior Faculty
- 2021 Recipient
- 2020 Recipient
- 2019 Recipient
- Leadership & Contacts
- Genetics Graduate Student Executive Council
- Committee Roster
- Equity and Inclusion Program (EQUIP)
What is Clinical Genetics
- Our Clinical Team
- Clinics & Making Appointments
- Impacting COVID-19 Outcomes through Genomics (ICOG)
- Special Events
INFORMATION FOR
- Residents & Fellows
- Researchers
Clinical Genetics
Yale Clinical Genetics is committed to providing excellent clinical care for adults and children in a setting that values compassion, collaboration, and respect for individuals and families.
Five clinical genetics physicians certified by the American Board of Medical Genetics provide state of the art genetic services including diagnosis, evaluation, counseling, and management for genetic and congenital conditions. The clinical team includes genetic counselors, APRNs, a genetic nutritionist and newborn screen nurse.
Clinical genetics
Diagnosis of chromosomal abnormalities, congenital malformations, mental retardation, and developmental delay, dysmorphic syndromes, connective tissue disorders, skeletal dysplasias, inherited neurologic disorders. Cytogenetic and molecular genetic testing and interpretation of results.
Cardiac genetics
Inherited cardiomyopathies, congenital heart disease associated with syndromes, Marfan syndrome, molecular testing and risk assessment for family members. Collaborative clinical assessment with pediatric cardiology; arrangement of assessment with adult cardiology.
Inborn Errors of Metabolism/Biochemical Genetics
Diagnosis, management and clinical care for patients with inborn errors of amino acid, organic acid, fatty acid metabolism and mitochondrial disorders. Newborn screening follow-up and assessment with rapid laboratory assessment and collaborative management with primary care physicians. Lysosomal storage disorders diagnosis and management with enzyme replacement. Molecular genetic testing with assessment of family members at risk.
Neurofibromatosis
Diagnosis and multi-system clinical assessment. Molecular genetic testing. Coordinated ongoing care and anticipatory guidance with collaboration of multiple specialists in neurology, surgery, dermatology, renovascular disease, orthopedics, ophthalomology and oncology for both adults and children.
Collaborative Clinical Research
Under the direction of Michele Spencer-Manzon, MD , Yale is engaged in two important collaborative clinical research projects.
- Urea Cycle Disorders Consortium - this consortium is engaged in research in the incidence, outcome, and treatment of inherited disorders of ammonia metabolism. For more information please Dr. Spencer-Manzon.
- Phenylketonuria: Response to Phenoptin - this consortium is engaged research in the response of phenylketonuria to supplementation with tetrahydrobiopterin. Enrollment for the current trial is closed, but inquiries about the next protocol are welcome. For more information, contact Nurse Coordinator, Brittany Holmes, APRN .
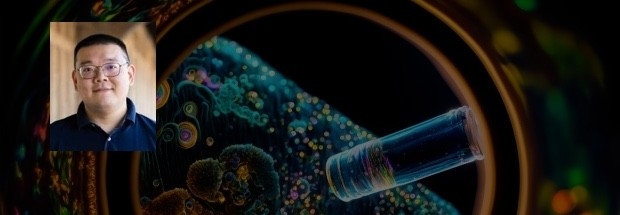
Our bacteria are more personal than we thought, Stanford Medicine-led study shows Learn More
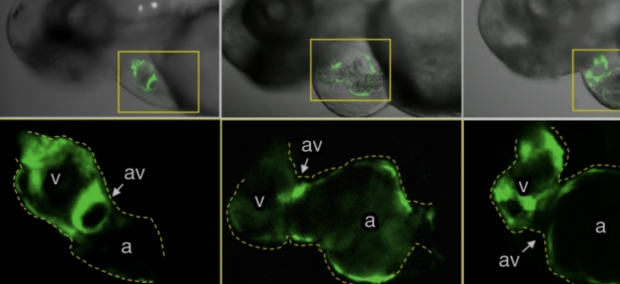
Researchers dial in on genetic culprit of disease Learn More
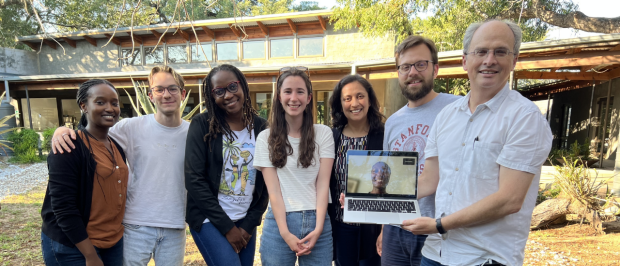
Global Health Equity Scholarship recipient Dylan Maghini conducts microbiome research with her team in South Africa. Learn more about her research
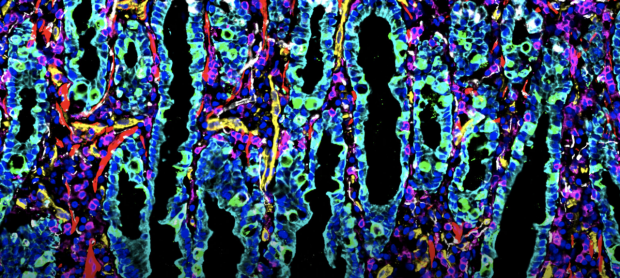
Snyder Lab and collaborators publish the first spatial map of the intestine at the single cell level. Learn More
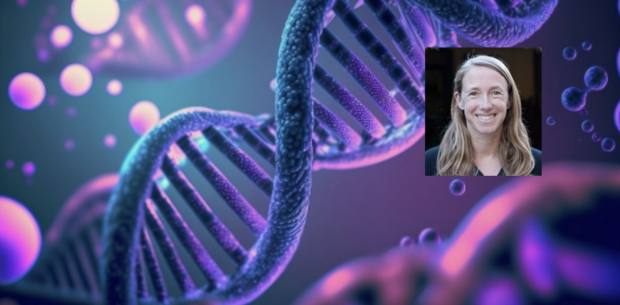
Can non-coding DNA change gene expression? Learn More
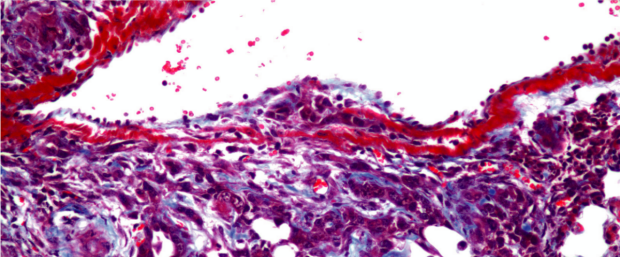
Genetics of Cancer Progression Mouse models of cancer enable an understanding of the metastatic process Learn More
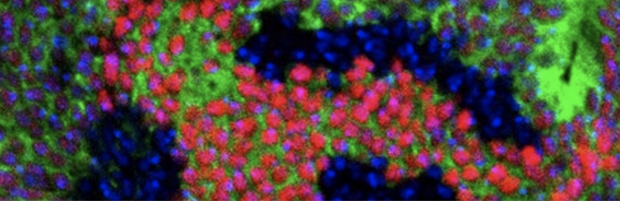
Genetics of Development Mosaic Drosphila Imaginal Disc Learn more
Genetics department news.
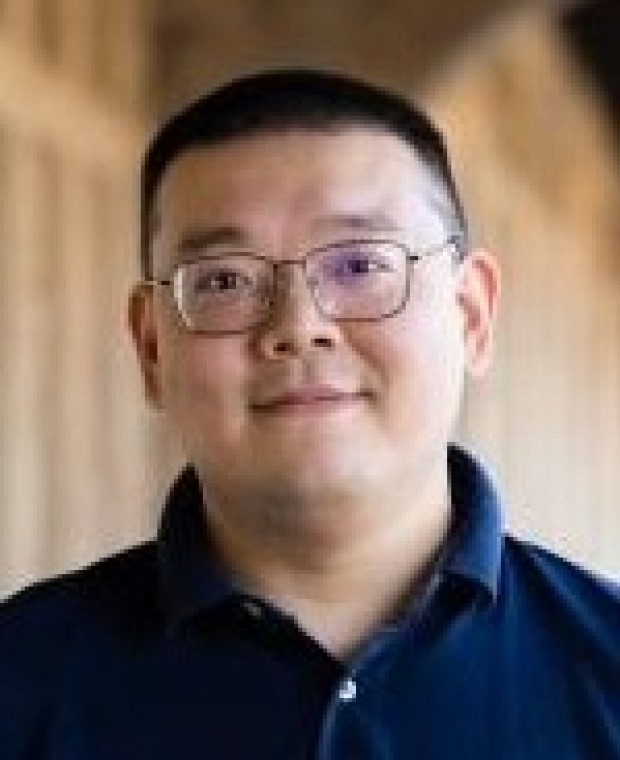
Xin Zhou, Ph.D. fellow researchers from Snyder Lab , and colleagues from multiple universities tracked the gut, mouth, nose and skin bacteria of 86 people for as long as six years to try to gauge what constitutes a healthy microbiome.
“We found that when you get sick with something like a cold, you have this temporary change in the microbiome; it becomes very dysregulated,” Xin Zhou, Ph.D. said. “With diabetes, that signature is the same in many ways except that it is long-term rather than temporary.” Learn More
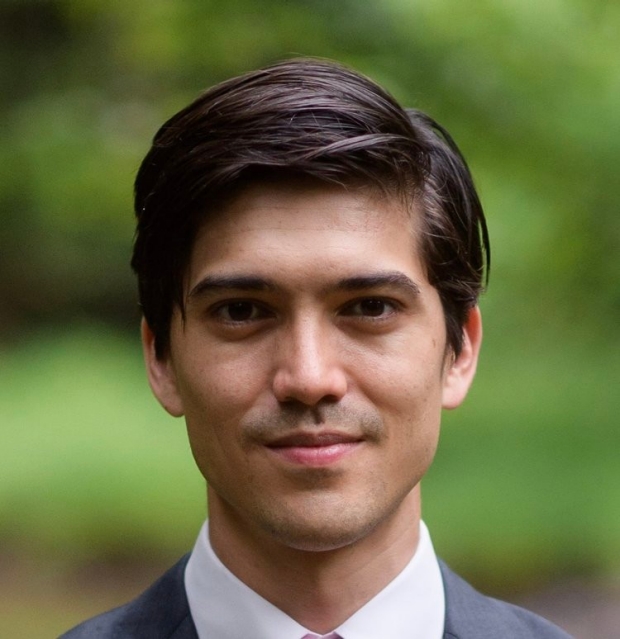
Jesse Engreitz , PhD discusses genetics underlying coronary artery disease in an interview with SCOPE. A new study co-led by researchers at Stanford Medicine and others, published Feb. 7 in Nature , aims to address this challenge by proposing a solution that links disease-causing DNA variants to the deleterious processes they set in motion. Learn More
December 2023
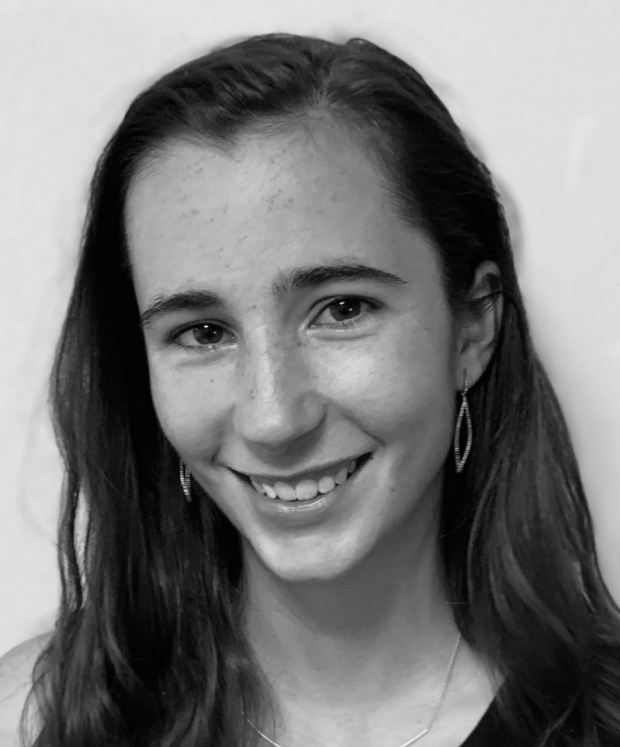
Global Health Equity Scholarship recipient Dylan Maghini conducts microbiome research with her team in South Africa.
Dylan's research focuses on the intersection between the gut microbiome and human health in a large cohort of nearly two thousand women in four countries (Burkina Faso, Ghana, Kenya, and South Africa).
This collaborative project between Stanford University and the University of the Witwatersrand seeks to measure microbiome composition in low- and middle-income populations, identify how the microbiome is shaped by environmental and lifestyle factors, and measure associations between the microbiome and pressing human health concerns in these populations. The project represents one of the largest population-representative gut microbiome studies in LMIC settings to date, and is an excellent example of collaborative, equitable, and community-engaged research. Learn more
August 2023
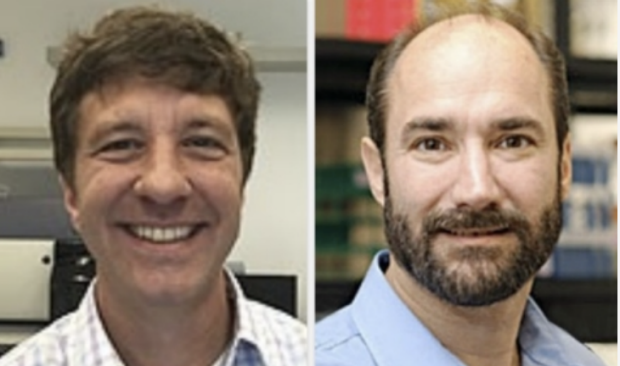
First Spatial Maps at the Single-Cell Level
Michael Angelo, PhD and Michael Snyder, PhD worked with Sanjay Jain, PhD, John Hickey, PhD and collaborators to "uncover how cellular interactions reveal new ways cells can communicate with each other".
By combining cellular imaging techniques, machine learning and other methods of molecular analyses, the teams are creating a comprehensive resource for researchers to better understand all human tissue. The data collected will be publicly available through HuBMAP, enabling researchers to study tissue-specific characteristics, understand disease mechanisms, and develop automated annotation tools that identify and characterize cells. Learn More
December 2022
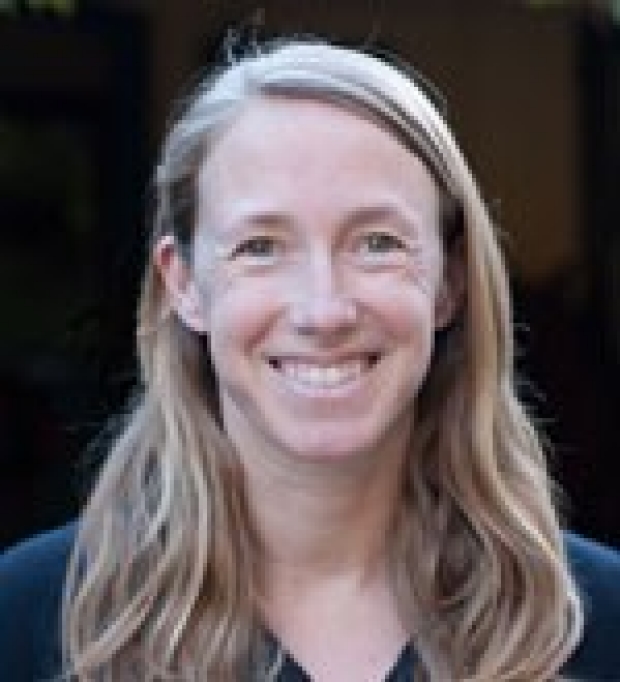
Professor Polly Fordyce is the recipient of the 2023 Eli Lilly Award in Biological Chemistry.
She is being recognized for her significant contributions to biological chemistry, especially her revolutionary work on applying high throughput biochemical techniques and analyses to investigate molecular recognition. Her novel strategies have dissected quantitative relationships that govern biological function. The work has contributed fundamental new insights into genetic variation, enzyme kinetics and thermodynamics.
October 2022
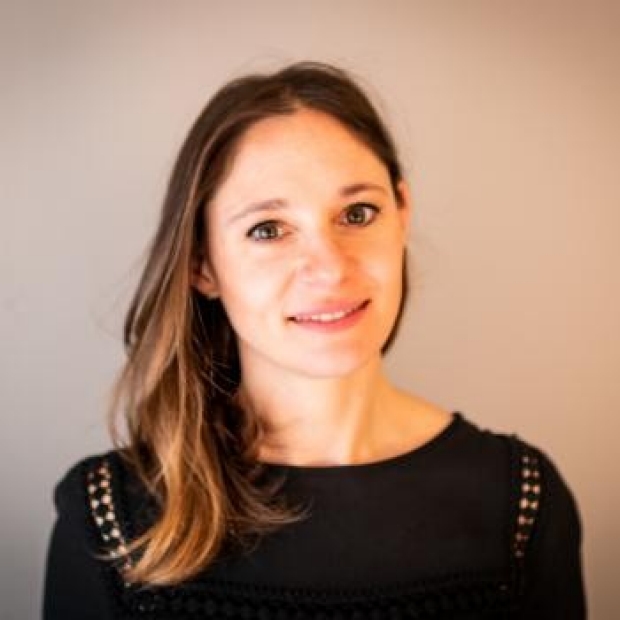
Serena Sanulli is named NIH Director's New Innovator Award Recipient.
Dr. Sanulli's lab studies genome organization across length and time scales with the long-term goal to understand how cells leverage the diverse biophysical properties of chromatin to regulate genome functions. She is the recipient of the Independent Postdoctoral Fellow Award from the program for Breakthrough Biomedical Research, the McCormick and Gabilan Faculty Fellowship, and she was recently named a Searle Scholar.

Two Key Types of Genes Identified The human genome includes millions of "enhancer" sequences that turn genes on and off—but it has been unclear which enhancers can regulate which genes. A new study led by researchers from the Engreitz Lab finds that two types of genes respond differently to enhancers, and that these responses are controlled by specific sequences in gene promoters. Link to article: https://rdcu.be/cNZxa
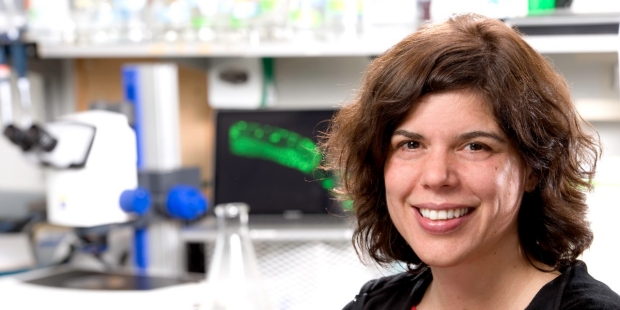
2022 Winners of the FNIH Lurie Prize in Biomedical Sciences Provide Powerful Contributions to Our Understanding of the Aging Process
The Foundation for the National Institutes of Health (FNIH) has named Anne Brunet, Ph.D., and Andrew Dillin, Ph.D., co-winners of the 2022 Lurie Prize in Biomedical Sciences
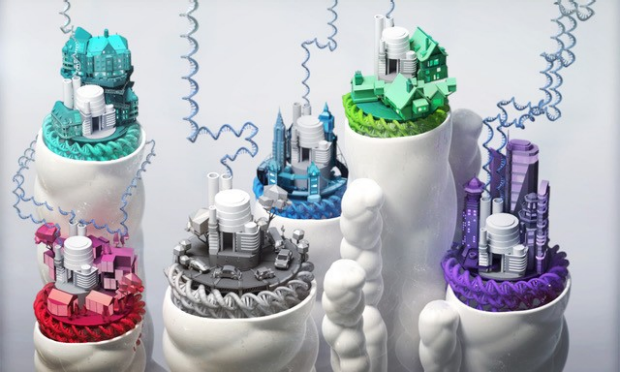
Transcriptional neighborhoods regulate transcript isoform lengths and expression levels
Authors: Aaron N. Brooks1†‡, Amanda L. Hughes1†, Sandra Clauder-Münster1, Leslie A. Mitchell2, Jef D. Boeke2,3, Lars M. Steinmetz1,4,5 *
Affiliations:
1European Molecular Biology Laboratory (EMBL), Genome Biology Unit, 69117 Heidelberg, Germany
2Institute for Systems Genetics and Department of Biochemistry and Molecular Pharmacology, NYU Langone Health, New York, NY 10016, USA.
3Department of Biomedical Engineering, NYU Tandon School of Engineering, Brooklyn, NY 11201, USA
4Stanford Genome Technology Center, Stanford University, Palo Alto, CA 94304, USA 5Department of Genetics, School of Medicine, Stanford University, Stanford, CA 94305, USA *Correspondence to: [email protected] †Equal contribution
Abstract: Sequence features of genes and their flanking regulatory regions are determinants of RNA transcript isoform expression and have been used as context-independent, plug-and-play modules in synthetic biology. However, genetic context, including the adjacent transcriptional environment, also influences transcript isoform expression levels and boundaries. We used synthetic yeast strains with stochastically repositioned genes to systematically disentangle sequence from contextual effects. Profiling 120 million full-length transcript molecules across 612 genomic perturbations, we observed sequence-independent alterations to gene expression levels and transcript isoform boundaries that were influenced by neighboring transcription. We identified features of transcriptional context that could predict these alterations and used these features to engineer a synthetic circuit where neighboring transcription controlled transcript length. This demonstrates how positional context can be leveraged in synthetic genome engineering.
February 2022
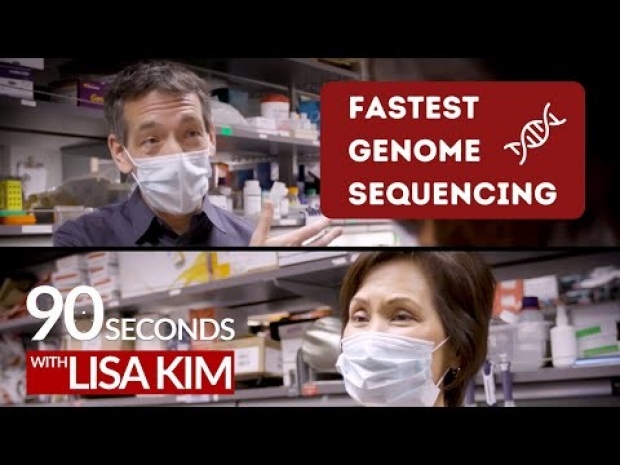
“90 Seconds with Lisa Kim”: Genome sequencing sets Guinness World Record
A new ultra-rapid genome sequencing approach developed by Stanford Medicine scientists sets the first Guinness World Record for the fastest DNA sequencing technique, producing results for one study participant in just over five hours. See the video on StanfordMed TODAY.
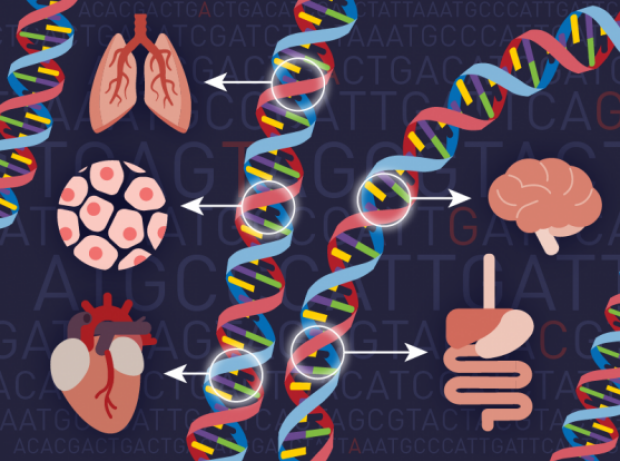
Genome-wide enhancer maps link risk variants to disease genes
Genome-wide association studies (GWAS) have identified thousands of noncoding loci that are associated with human diseases and complex traits, each of which could reveal insights into the mechanisms of disease 1 . Many of the underlying causal variants may affect enhancers 2 , 3 , but we lack accurate maps of enhancers and their target genes to interpret such variants. Read more...
Image credit: Zayna Sheikh
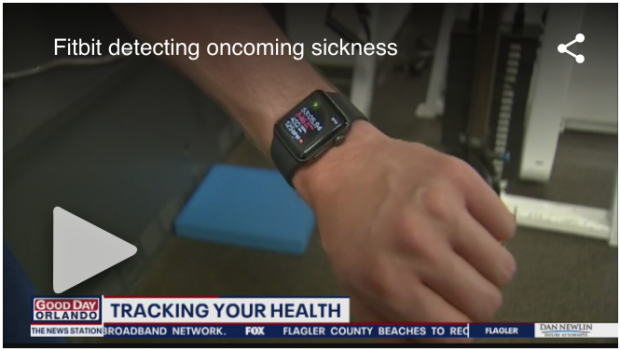
Fitbit detecting oncoming sickness
Dr. Michael Snyder discovered that among the millions of measurements they make every day, subtle variances in a Fitbit's data could be a predictor of an oncoming illness.
Read more...
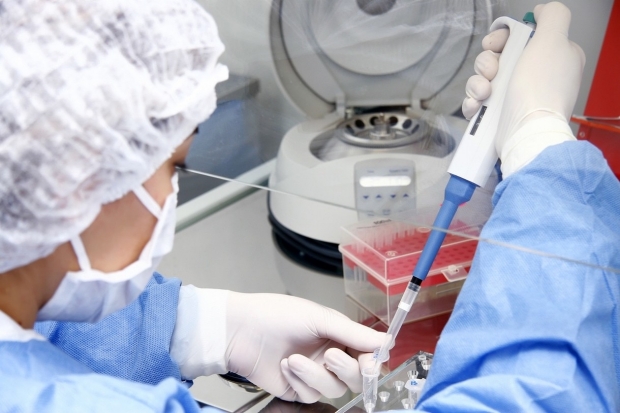
Department of Genetics COVID-19 Research
Our scientists from the Department of Genetics have launched research projects as part of the global response to COVID-19.

Stanford Medicine scientists hope to use data from wearable devices to predict illness, including COVID-19
Researchers from Stanford Medicine and their collaborators aim to predict the onset of viral infection through data provided by wearable technology. What they need now are participants.
Full Story...
September 2018

We are bombarded by thousands of diverse species and chemicals
We are all exposed to a vast and dynamic cloud of microbes, chemicals and particulates that, if visible, might make us look something like Pig-Pen from Peanuts.
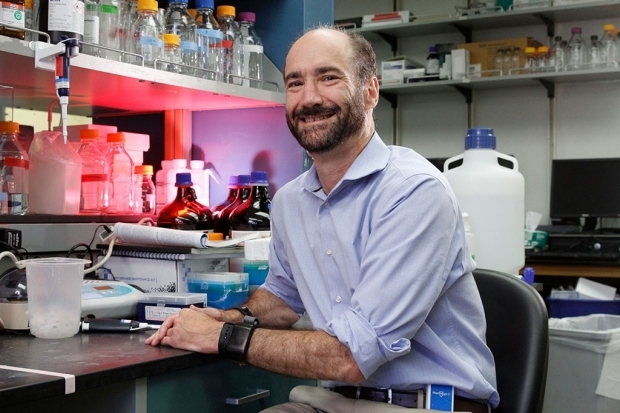
Researchers can forecast risk of deadly vascular condition from genome sequence
A new approach that distills deluges of genetic data and patient health records has identified a set of telltale patterns that can predict a person’s risk for a common, and often fatal, cardiovascular disease, according to a new study from the Stanford University School of Medicine .
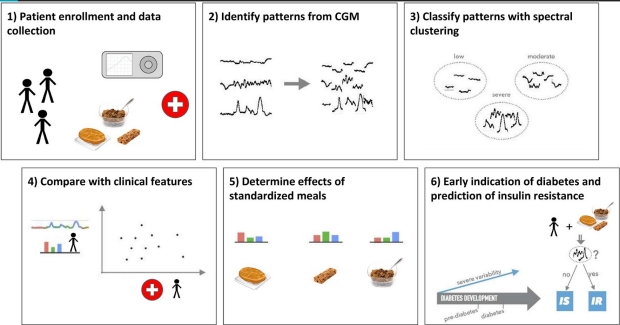
Diabetic-level glucose spikes seen in healthy people
A study out of Stanford in which blood sugar levels were continuously monitored reveals that even people who think they’re “healthy” should pay attention to what they eat.

New center sets out to stop disease before it starts
At the Precision Health and Integrated Diagnostics Center, scientists turn the norms of disease research on their head, searching not for treatments but for ways to prevent disease entirely.
It’s not often that world-class scientists band together to investigate disease with no intention of curing it. Yet upward of 55 scientists at Stanford’s Precision Health and Integrated Diagnostics Center are doing just that in a push to get researchers and physicians off their heels and onto their toes in the battle against disease..
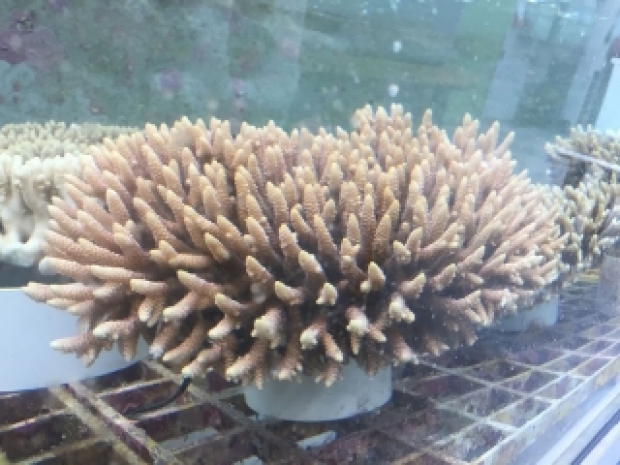

CRISPR used to genetically edit coral
In a proof-of-principle study, Stanford scientists and their colleagues used the CRISPR-Cas9 gene-editing system to modify genes in coral, suggesting that the tool could one day aid conservation efforts.
Coral reefs on the precipice of collapse may get a conservation boost from the gene-editing tool known as CRISPR, according to researchers at the Stanford University School of Medicine and their collaborators.
January 2018

Weight flux alters molecular profile
Stanford scientists have found links between changes in a person’s weight and shifts in their microbiome, immune system and cardiovascular system.
A paper describing the work was published online Jan. 17 in Cell Systems . The lead authors are Stanford postdoctoral scholars Wenyu Zhou, PhD, and Hannes Röst, PhD ; staff scientist Kévin Contrepois, PhD; and former postdoctoral scholar Brian Piening, PhD . Senior authorship is shared by Michael Snyder , PhD, professor of genetics at Stanford; Tracey McLaughlin , MD, professor of medicine at Stanford; and George Weinstock , PhD, professor and director of microbial genomics at the Jackson Laboratory , an independent, nonprofit biomedical research institution.
October 2017
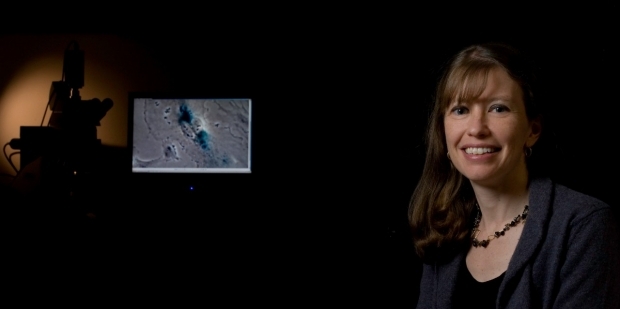
Study uncovers mutation that supercharges tumor-suppressor
Cancer researchers have long hailed p53, a tumor-suppressor protein, for its ability to keep unruly cells from forming tumors. But for such a highly studied protein, p53 has hidden its tactics well.
Now, researchers at the Stanford University School of Medicine have tapped into what makes p53 tick, delineating a clear pathway that shows how the protein mediates anti-tumor activity in pancreatic cancer. The team’s research also revealed something unexpected: A particular mutation in the p53 gene amplified the protein’s tumor-fighting capabilities, creating a “super tumor suppressor.”
Full story...
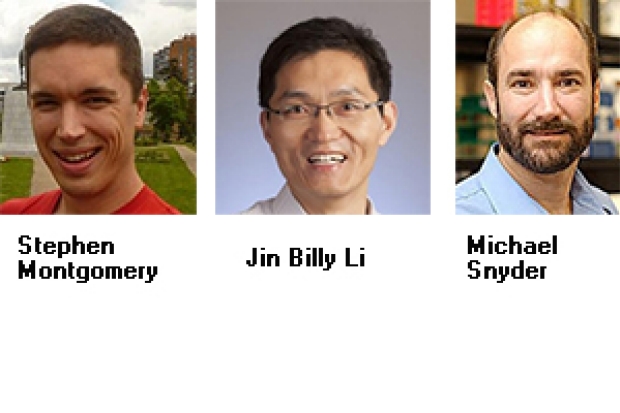
Tissue-specific gene expression uncovered, linked to disease
Understanding how a person’s DNA sequence affects gene expression in various tissues reveals the molecular mechanisms of disease. Stanford scientists involved in the National Institutes Health’s GTEx project have published some of their insights.
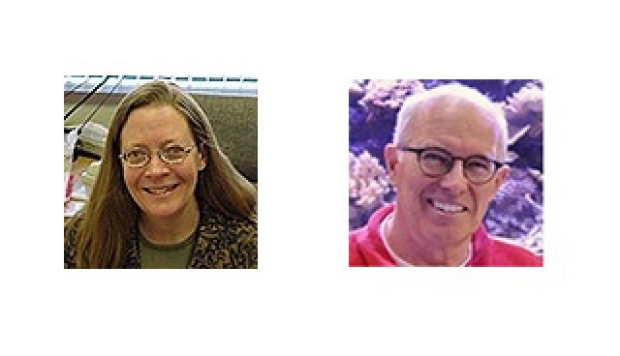
John Pringle and Anne Villeneuve elected to National Academy of Sciences
Three Stanford researchers are among the 84 newly elected members of the National Academy of Sciences .
The new members from Stanford are Dominique Bergmann , PhD, professor of biology; John Pringle , PhD, professor of genetics; and Anne Villeneuve , PhD, professor of developmental biology and of genetics. Full story..
February 2017
$10.5 million awarded to researchers to work on dna encyclopedia.
Stanford’s William Greenleaf, Michael Bassik, Michael Snyder, Jonathan Pritchard and Michael Cherry have won grants to work on the federally funded Encyclopedia of DNA Elements. Full story..
January 2017
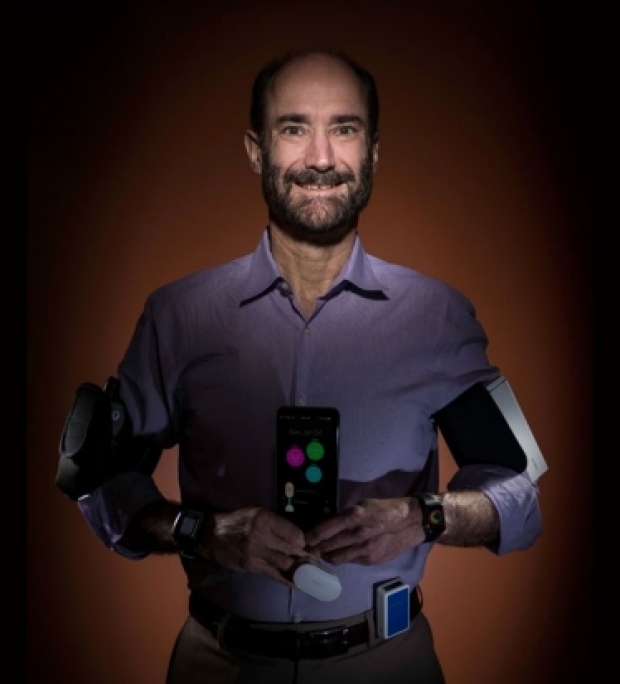
Wearable sensors can tell when you are getting sick
New research from Stanford shows that fitness monitors and other wearable biosensors can tell when an individual’s heart rate, skin temperature and other measures are abnormal, suggesting possible illness. Full story..
Interested in applying to the Ph.D. Program?
The Ph.D. program in the Department of Genetics provides opportunities for graduate study in all major areas of modern genetics, including identification and analysis of human disease genes, molecular evolution, gene therapy, statistical genetics, application of model organisms to problems in biology and medicine, and computational and experimental approaches to genome biology.
Learn more about the Genetics Ph.D. Program here .

Department Chair
" Genetics and genomics are undergoing an unparalleled revolution: our mission is to continue to lead this revolution for a better understanding of biology and human health. "
Michael Snyder, Ph.D. Stanford W. Ascherman Professor and Chair, Department of Genetics Director, Center for Genomics and Personalized Medicine
Show Your Support
A gift to the Stanford Genetics department supports our research and education. Donations are vital to the achievements of our work and are greatly appreciated. Checks payable to Stanford University. Please note on the check WAZC/Genetics and specifics of where the funds should be directed. Thank you.
Kindly send by mail to:
Development Services PO Box 20466 Stanford, CA 94309
CALL US: 650.725.2504
CONTACT US: [email protected]
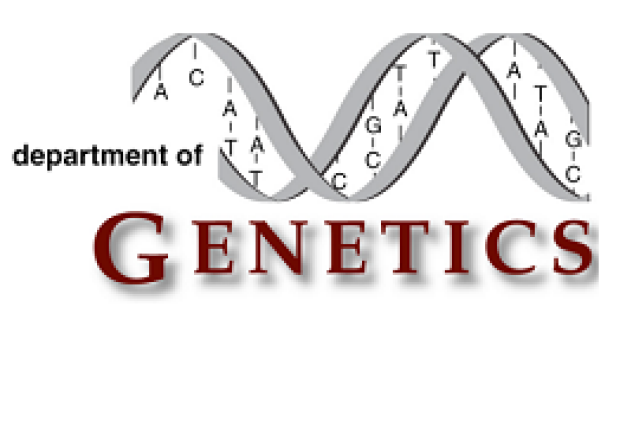
An underlying theme in our Department is that genetics is not merely a set of tools but a coherent and fruitful way of thinking about biology and medicine. To this end, we emphasize a spectrum of approaches based on molecules, organisms, populations, and genomes.
We provide training through laboratory rotations, dissertation research, seminar series, didactic and interactive coursework, and an annual three-day retreat of nearly 200 students, faculty, postdoctoral fellows, and research staff.
The mission of the Department includes education and teaching as well as research; graduates from our program pursue careers in many different venues including research in academic or industrial settings, health care, health policy, and education. We are especially committed to increasing diversity within the program, and to the training of individuals from traditionally underrepresented minority groups to apply.
#1 Graduate School in Genetics/Genomics/Bioinformatics by U.S. News
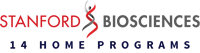
Announcements
Luigi luca cavalli-sforza ( 1922 - 2018), len herzenberg (1931-2013), david r. cox-(1946-2013), the stanford genetics and genomics certificate.
Open to the public: Take online courses in the Stanford Genetics and Genomics Certificate to gain fundamental knowledge and a 'big picture' understanding of the cutting-edge fields of genetics, genomics and personalized medicine.
- Foundations in Genetics and Genomics Certificate
- Advanced Genetics and Genomics Certificate
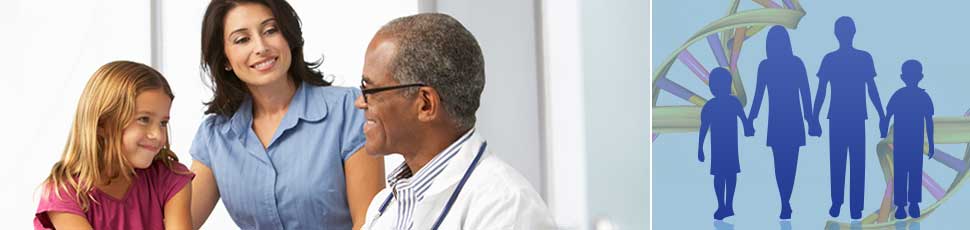
Clinical Genetics Branch
Clinical epidemiology unit.
Research Areas
CGB Principal Investigators
Current Fellows
Research Training Opportunities
Tools & Resources
Publications
CGB Staff Directory
Working towards understanding the causes of cancer to end the burden of cancer in families and populations.
Clinical Genetics Branch (CGB) investigators study individuals at and populations at high genetic risk of cancer in order to improve our understanding of cancer etiology and to advance clinical care. Our multidisciplinary approach combines clinical, genetic, genomic, epidemiologic, behavioral, statistical, and laboratory scientific research modalities. Read about some of our contributions to improving public health for these high-risk individuals .
Research Mission
The CGB research mission is to conduct clinical, genomic, and epidemiologic cancer research and translate that knowledge to improve prevention, screening, and management of cancer in families and populations at high risk. Learn about specific CGB research areas .
The Clinical Epidemiology Unit (CEU) within CGB conducts etiologic research with potential clinical and public health applications and leads studies evaluating population-based early detection and cancer prevention strategies.
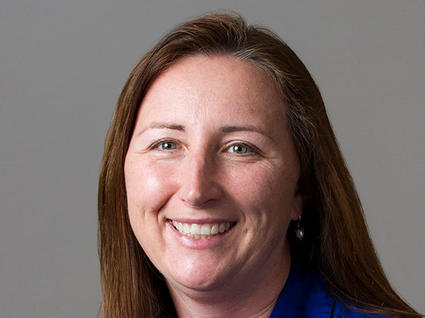
CHARMS: A Stable and Modern Infrastructure for Family Studies’ Data
Dr. Savage describes new infrastructure for managing family study data.
Fellowships
CGB fellows work with researchers engaged in conducting clinical, genetic, and epidemiologic studies focused on high-risk families, individuals, and populations. They pursue astute clinical observations that might provide new clues to cancer etiology, and apply and develop epidemiologic methods to the study of high-risk individuals.
Meet the current CGB fellows and learn about research training opportunities in CGB .
CGB Highlights
- Hansen H et al. Management of individuals with germline pathogenic/likely pathogenic variants in CHEK2: A clinical practice resource of the American College of Medical Genetics and Genomics (ACMG) . Genet Med. 2023 .
- Cohen CM et al. Racial and Ethnic Disparities in Cervical Cancer Incidence, Survival, and Mortality by Histologic Subtype . J Clin Oncol . 2022.
- Brown D et al. Germline-somatic JAK2 interactions are associated with clonal expansion in myelofibrosis. Nature Commun. 2022.
- Egemen D et al. Variation in Human Papillomavirus Vaccination Effectiveness in the US by Age at Vaccination . JAMA Netw Open. 2022 .
- McReynolds LJ et al. Genetic testing in severe aplastic anemia is required for optimal hematopoietic cell transplant outcomes . Blood. 2022.
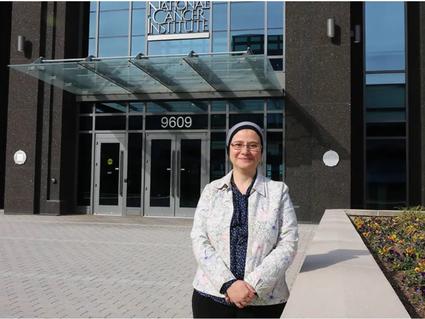
NIH Intramural Research Program Features Senior Investigator Shahinaz Gadalla
Dr. Gadalla discusses her research on stem cell transplants and the path to improving survival.
- Sargen M, et al. Spectrum of Nonkeratinocyte Skin Cancer Risk Among Solid Organ Transplant Recipients in the US . JAMA Dermatol. 2022.
- Kuhs KAL et al. Genetic variation within the human papillomavirus type 16 genome is associated with oropharyngeal cancer prognosis . Ann Oncol. 2022.
- Gadalla SM et al. Association of donor IFNL4 genotype and non-relapse mortality after unrelated donor myeloablative haematopoietic stem-cell transplantation for acute leukaemia: a retrospective cohort study . Lancet Haematol. 2020.
- Mirabello L et al. Frequency of Pathogenic Germline Variants in Cancer-Susceptibility Genes in Patients With Osteosarcoma . JAMA Oncol. 2020.
- Wentzensen N et al. Accuracy and Efficiency of Deep-Learning-Based Automation of Dual Stain Cytology in Cervical Cancer Screening. J Natl Cancer Inst. 2020.
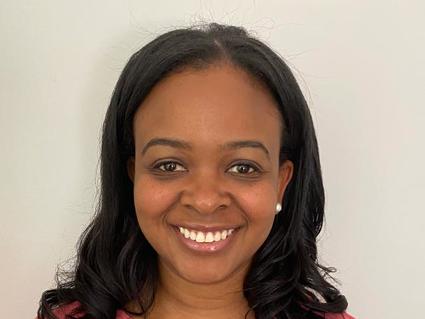
2024 NCI Diversity Career Development Program
Postdoctoral fellow Lesley Chapman Hannah was selected to join the 2024 cohort.
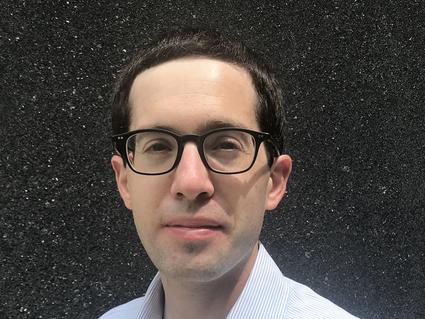
2024 CCR-DCEG FLEX Award
Dr. Michael Sargen co-leads the awarded project, which aims to better understand the causes of pediatric melanoma and identify treatment strategies.

- The Extramural Research Program
- An Overview
- Bioinformatics
- Current Grants
- Education and Training Funding
- Extramural Research News Features
- Funding Divisions
- Funding Opportunities
- Funded Programs and Projects
- Grant Information
- NIH Common Fund
- The Division of Intramural Research (DIR)
- Clinical Research
- DIR Calendar
- DIR News Features
- NHGRI Affiliated Centers
- Online NHGRI Research Resources
- Organizational Chart
- Publications, Books and Resources
- Research Investigators
- For Patients and the Public:
- Community Engagement & Community Health Resources
- Family History Initiative
- Finding Reliable Health Information Online
- Genetic & Genomic Science and Research
- Genetic & Rare Diseases Information Center (GARD)
- Genetic Disorders, Genomics & Healthcare
- Genomic Medicine
- Online Health Resources
- For Health Professionals:
- Competency & Curricular Resources
- Genetics 101
- New Horizons and Research
- Patient Management
- Policy and Ethics Issues
- Quick Links for Patient Care
- All About the Human Genome Project
- Fact Sheets
- Genetic Education Modules
- Genomic Careers
- National DNA Day
- Online Education Kit
- Online Genetics Education Resources
- Smithsonian NHGRI Genome Exhibition
- Talking Glossary: English
- Talking Glossary: Español
- Coverage & Reimbursement of Genetic Tests
- Ethics Research at NHGRI
- Genetic Discrimination
- Regulation of Genetic Tests
- Health Issues in Genetics
- Informed Consent for Genomics Research
- Intellectual Property
- Online Bioethics Resources
- Statute and Legislation Database
- Calendar of Events
- Current News Releases
- Digital Media Database
- Media Contacts
- Media Resources
- NHGRI-Related News
- Recent Journal Articles from NHGRI
- Social Media
- Educational Programs
- Health Professional Education
- Intramural Training Office
- Online Careers & Training Resources
- Training Programs: Funding
- Training Programs: Intramural
- Working at NHGRI
- About the Institute
- Budget and Financial Information
- Director's Page
- Historical Archiving Initiative
- How to Contact Us
- Institute Advisors
- Long-Range Planning
- Minority and Special Populations
- Office of the Director
- Organization
- Reports & Publications
- Español

- External link, please review our disclaimer

Skip to content
Researchers Publish Final Results of Key Clinical Trial for Gene Therapy for Sickle Cell Disease
Published on Apr 24, 2024
Cancer Center
In a landmark study, an international consortium led by researchers at Children’s Hospital of Philadelphia (CHOP) published the final results of a key clinical trial of the gene therapy CASGEVY (exagamglogene autotemcel) for the treatment of sickle cell disease in patients 12 years and older with recurrent vaso-occlusive crises (VOCs). The study found that 96.7% of patients in the study did not have any vaso-occlusive crises (VOCs) – a blockage that results in lack of oxygen and painful episodes – for at least one year, and 100% were able to remain hospitalization-free for the same length of time.
The findings, published today in the New England Journal of Medicine , provide the complete details of the critical clinical trial that led to the FDA approval of CASGEVY™ for the treatment of sickle cell disease in December 2023.
Sickle cell disease is a lifelong condition that causes intense pain due to deformed blood cells that can cause blockages in blood vessels. This can also lead to strokes, organ damage, and shortened lives.
Researchers have been studying the use of gene therapy and CRISPR technology to edit portions of DNA in people with inherited or genetic disorders, like sickle cell disease. In the case of sickle cell disease, the CASGEVY process edits DNA within the patient’s own cells and enables the patient to produce a different form of hemoglobin in their red blood cells. Clinical trials at CHOP and other sites have shown that successful gene editing can prevent cells from developing the distinctive crescent shape apparent in sickle cell disease and have eliminated pain episodes in almost all patients. CASGEVY was the first FDA-approved therapy developed with CRISPR technology.
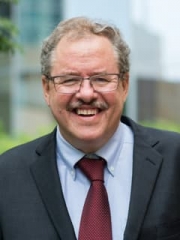
The researchers conducted the CLIMB SCD-121 trial, a phase 3, single-arm, open-label study of exa-cel in patients between 12 and 35 years old with sickle cell disease and at least two severe VOCs in each of the two years before screening. The key primary endpoint of the study was a proportion of patients without severe VOCs for at least 12 consecutive months, with a secondary endpoint of patients who were free from inpatient hospitalization for severe VOCs for at least 12 consecutive months.
A total of 44 patients received exa-cel with a median follow up of 19.3 months. In a total of 30 patients with sufficient follow-up data to be evaluated, 29 (96.7%) were free of VOCs for at least 12 consecutive months. This information is an update for the US Prescribing Information for CASGEVY, which includes an evaluation of 31 patients resulting in a response rate of 93.5%. The safety of treatment was comparable to treatment with hematopoietic and progenitor stem cells, and no malignancies were reported as a result of treatment.
This study was supported by Vertex Pharmaceuticals and CRISPR Therapeutics.
For more information about CASGEVY’s prescribing information, warning and precautions, and adverse reactions, visit here .
Additionally, the results of a clinical trial on the efficacy of exa-cel for the treatment of β-thalassemia were also published today in the New England Journal of Medicine . The preliminary results of the trial led to the FDA approval of CASGEVY for transfusion-dependent β-thalassemia in January 2024. Grupp was also one of the principal investigators for this clinical trial.
Frangoul et al, “Exagamglogene Autotemcel for Severe Sickle Cell Disease.” N Engl J Med . Online April 24, 2024. DOI: 10.1056/NEJMoa2309676.
Locatelli et al, “Exagamglogene Autotemcel for Transfusion-Dependent β-Thalassemia.” N Engl J Med . Online April 24, 2024. DOI: 10.1056/NEJMoa2309673.
Contact: Jennifer Lee, The Children’s Hospital of Philadelphia, 267-426-6084 or [email protected]
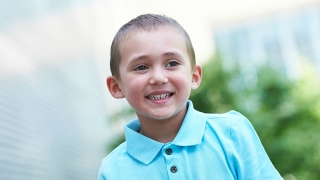
Get a Second Opinion
Our experts are here to review your child’s diagnosis and treatment plan, and work with primary oncologists as needed.
- U.S. Department of Health & Human Services

- Virtual Tour
- Staff Directory
- En Español
You are here
News releases.
Media Advisory
Friday, April 26, 2024
Analysis identifies 50 new genomic regions associated with kidney cancer risk
In a new analysis of genetic susceptibility to kidney cancer, an international team of researchers has identified 50 new areas across the genome that are associated with the risk of developing kidney cancer. These insights could one day be used to advance our understanding of the molecular basis of kidney cancer, inform screening efforts for those at highest risk, and identify new drug targets. The study was led by scientists at the National Cancer Institute (NCI), part of the National Institutes of Health (NIH).
A previous genome-wide association study (GWAS) of people of European ancestry identified 13 regions of the genome that are associated with kidney cancer risk. However, the study population was not diverse. To identify additional regions, researchers conducted a GWAS in participants of many different genetic ancestries that included 29,020 people with kidney cancer and 835,670 people without kidney cancer. Analysis of the data, which came from published studies, biobanks, and a new study, resulted in the identification of 50 new regions associated with the risk of developing kidney cancer, bringing the total number of such regions to 63.
Among the newly identified genetic variants were several associated with a risk of developing papillary renal cell carcinoma, the second most common subtype of renal cell carcinoma. Another variant, in the VHL gene, was common in individuals of African ancestry and was associated with an estimated three times higher risk of developing clear cell renal cell carcinoma, the most common type of kidney cancer.
Finally, the researchers used the study data to develop a measure of an individual’s overall risk of developing kidney cancer, known as a polygenic risk score , that can be combined with established risk factors—such as high blood pressure, smoking, and a high body-mass index measurement—to possibly enable earlier detection of the disease.
Mark P. Purdue, Ph.D., Division of Cancer Epidemiology and Genetics , National Cancer Institute
“ Multi-ancestry genome-wide association study of kidney cancer identifies 63 susceptibility regions ” appears April 26, 2024, in Nature Genetics .
About the National Cancer Institute (NCI): NCI leads the National Cancer Program and NIH’s efforts to dramatically reduce the prevalence of cancer and improve the lives of people with cancer. NCI supports a wide range of cancer research and training extramurally through grants and contracts. NCI’s intramural research program conducts innovative, transdisciplinary basic, translational, clinical, and epidemiological research on the causes of cancer, avenues for prevention, risk prediction, early detection, and treatment, including research at the NIH Clinical Center—the world’s largest research hospital. Learn more about the intramural research done in NCI’s Division of Cancer Epidemiology and Genetics . For more information about cancer, please visit the NCI website at cancer.gov or call NCI’s contact center at 1-800-4-CANCER (1-800-422-6237).
About the National Institutes of Health (NIH): NIH, the nation's medical research agency, includes 27 Institutes and Centers and is a component of the U.S. Department of Health and Human Services. NIH is the primary federal agency conducting and supporting basic, clinical, and translational medical research, and is investigating the causes, treatments, and cures for both common and rare diseases. For more information about NIH and its programs, visit www.nih.gov .
NIH…Turning Discovery Into Health ®
Connect with Us
- More Social Media from NIH
The chromosomal characteristics of spontaneous abortion and its potential associated copy number variants and genes
- Published: 26 April 2024
Cite this article
- Koksear Touch 1 ,
- Menghan Sha 2 ,
- Yanan Sun 1 ,
- Shunran Zhang 1 ,
- Jianli Wu 1 ,
- Yuanyuan Wu 1 ,
- Ling Feng 1 ,
- Suhua Chen ORCID: orcid.org/0000-0001-8425-0768 1 &
- Juan Xiao 1
This study aimed to investigate the correlation between chromosomal abnormalities in spontaneous abortion with clinical features and seek copy number variations (CNVs) and genes that might be connected to spontaneous abortion.
Over 7 years, we used CNV-seq and STR analysis to study POCs, comparing chromosomal abnormalities with clinical features and identifying critical CNVs and genes associated with spontaneous abortion.
Total chromosomal variants in the POCs were identified in 66.8% (2169/3247) of all cases, which included 45.2% (1467/3247) numerical abnormalities and 21.6% (702/3247) copy number variants (CNVs). Chromosome number abnormalities, especially aneuploidy abnormalities, were more pronounced in the group of mothers aged ≥ 35 years, the early miscarriage group, and the chorionic villi group. We further analyzed 212 pathogenic and likely pathogenic CNVs in 146 POCs as well as identified 8 statistically significant SORs through comparison with both a healthy population and a group of non-spontaneously aborted fetuses. Our analysis suggests that these CNVs may play a crucial role in spontaneous abortion. Furthermore, by utilizing the RVIS score and MGI database, we identified 86 genes associated with spontaneous abortion, with particular emphasis on PARP6, ISLR, ULK3, FGFRL1, TBC1D14, SCRIB, and PLEC.
We found variability in chromosomal abnormalities across clinical features, identifying eight crucial copy number variations (CNVs) and multiple key genes that may be linked to spontaneous abortion. This research enhances the comprehension of genetic factors contributing to spontaneous abortion.
This is a preview of subscription content, log in via an institution to check access.
Access this article
Price includes VAT (Russian Federation)
Instant access to the full article PDF.
Rent this article via DeepDyve
Institutional subscriptions
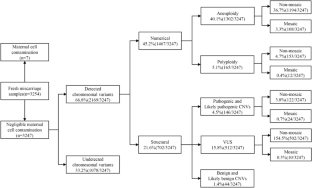
Similar content being viewed by others
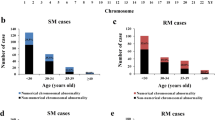
Chromosomal Copy Number Variation Analysis in Pregnancy Products from Recurrent and Sporadic Miscarriage Using Next-Generation Sequencing
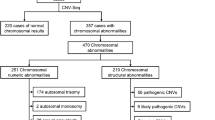
Identification of chromosomal abnormalities in miscarriages by CNV-Seq
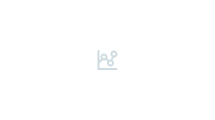
Ontogenetic Pleiotropy of Genes Involved in CNVs in Human Spontaneous Abortions
Data availability.
The datasets generated during and/or analyzed during the current study are available from the corresponding author upon reasonable request.
Luise C, Jermy K, May C, et al. Outcome of expectant management of spontaneous first trimester miscarriage: observational study. BMJ (Clin Res Ed). 2002;324(7342):873–5.
Article Google Scholar
Quenby S, Gallos ID, Dhillon-Smith RK, et al. Miscarriage matters: the epidemiological, physical, psychological, and economic costs of early pregnancy loss. Lancet (London, England). 2021;397(10285):1658–67.
Article CAS PubMed Google Scholar
Kersting A, Wagner B. Complicated grief after perinatal loss. Dialogues Clin Neurosci. 2012;14(2):187–94.
Article PubMed PubMed Central Google Scholar
Cubo AM, Soto ZM, Haro-Pérez A, et al. Medical versus surgical treatment of first trimester spontaneous abortion: a cost-minimization analysis. PLoS One. 2019;14(1):e0210449.
Article PubMed Google Scholar
Hardy K, Hardy PJ, Jacobs PA, et al. Temporal changes in chromosome abnormalities in human spontaneous abortions: results of 40 years of analysis. Am J Med Genet A. 2016;170(10):2671–80.
Zhu XZ, Deng ZM, Dai FF, et al. The impact of early pregnancy metabolic disorders on pregnancy outcome and the specific mechanism. Eur J Med Res. 2023;28(1):197.
Wang Y, Li Y, Chen Y, et al. Systematic analysis of copy-number variations associated with early pregnancy loss. Ultrasound Obstet Gynecol Off J Int Soc Ultrasound Obstet Gynecol. 2020;55(1):96–104.
Article CAS Google Scholar
Fan L, Wu J, Wu Y, et al. Analysis of chromosomal copy number in first-trimester pregnancy loss using next-generation sequencing. Front Genet. 2020;11:545856.
Article CAS PubMed PubMed Central Google Scholar
Zhang X, Wu H, Gu Z, et al. Chromosomal copy number variation analysis in pregnancy products from recurrent and sporadic miscarriage using next-generation sequencing. Reprod Sci. 2022;29(10):2927–36.
Martinez-Portilla RJ, Pauta M, Hawkins-Villarreal A, et al. Added value of chromosomal microarray analysis over conventional karyotyping in stillbirth work-up: systematic review and meta-analysis. Ultrasound Obstet Gynecol Off J Int Soc Ultrasound Obstet Gynecol. 2019;53(5):590–7.
Gao J, Liu C, Yao F, et al. Array-based comparative genomic hybridization is more informative than conventional karyotyping and fluorescence in situ hybridization in the analysis of first-trimester spontaneous abortion. Mol Cytogenet. 2012;5(1):33.
Hayes JL, Tzika A, Thygesen H, et al. Diagnosis of copy number variation by Illumina next generation sequencing is comparable in performance to oligonucleotide array comparative genomic hybridisation. Genomics. 2013;102(3):174–81.
Liang D, Peng Y, Lv W, et al. Copy number variation sequencing for comprehensive diagnosis of chromosome disease syndromes. J Mol Diagn JMD. 2014;16(5):519–26.
Ma N, Xi H, Chen J, et al. Integrated CNV-seq, karyotyping and SNP-array analyses for effective prenatal diagnosis of chromosomal mosaicism. BMC Med Genomics. 2021;14(1):56.
Edwards A, Civitello A, Hammond HA, et al. DNA typing and genetic mapping with trimeric and tetrameric tandem repeats. Am J Hum Genet. 1991;49(4):746–56.
CAS PubMed PubMed Central Google Scholar
Brosens JJ, Bennett PR, Abrahams VM, et al. Maternal selection of human embryos in early gestation: insights from recurrent miscarriage. Semin Cell Dev Biol. 2022;131:14–24.
Bender Atik R, Christiansen OB, Elson J, et al. ESHRE guideline: recurrent pregnancy loss: an update in 2022. Hum Reprod Open. 2023;2023(1):hoad002.
PubMed PubMed Central Google Scholar
Magnus MC, Wilcox AJ, Morken NH, et al. Role of maternal age and pregnancy history in risk of miscarriage: prospective register based study. BMJ (Clin Res Ed). 2019;364:l869.
Google Scholar
Liang D, Cram DS, Tan H, et al. Clinical utility of noninvasive prenatal screening for expanded chromosome disease syndromes. Genet Med Off J American College Med Genet. 2019;21(9):1998–2006.
CAS Google Scholar
Riggs ER, Andersen EF, Cherry AM, et al. Technical standards for the interpretation and reporting of constitutional copy-number variants: a joint consensus recommendation of the American College of Medical Genetics and Genomics (ACMG) and the Clinical Genome Resource (ClinGen). Genet Med Off J American College Med Genet. 2020;22(2):245–57.
Xu H, Poh WT, Sim X, et al. SgD-CNV, a database for common and rare copy number variants in three Asian populations. Hum Mutat. 2011;32(12):1341–9.
Wang J, Chen L, Zhou C, et al. Prospective chromosome analysis of 3429 amniocentesis samples in China using copy number variation sequencing. American journal of obstetrics and gynecology. 2018;219(3):287.e1-.e18.
Petrovski S, Wang Q, Heinzen EL, et al. Genic intolerance to functional variation and the interpretation of personal genomes. PLoS Genet. 2013;9(8):e1003709.
Finley J, Hay S, Oldzej J, et al. The genomic basis of sporadic and recurrent pregnancy loss: a comprehensive in-depth analysis of 24,900 miscarriages. Reprod Biomed Online. 2022;45(1):125–34.
Levy B, Sigurjonsson S, Pettersen B, et al. Genomic imbalance in products of conception: single-nucleotide polymorphism chromosomal microarray analysis. Obstet Gynecol. 2014;124(2 Pt 1):202–9.
Liu S, Song L, Cram DS, et al. Traditional karyotyping vs copy number variation sequencing for detection of chromosomal abnormalities associated with spontaneous miscarriage. Ultrasound Obstet Gynecol Off J Int Soc Ultrasound Obstet Gynecol. 2015;46(4):472–7.
Wang MZ, Lin FQ, Li M, et al. Semiconductor sequencing analysis of chromosomal copy number variations in spontaneous miscarriage. Med Sci Monit. 2017;23:5550–7.
Hanna JS, Shires P, Matile G. Trisomy 1 in a clinically recognized pregnancy. Am J Med Genet. 1997;68(1):98.
Dunn TM, Grunfeld L, Kardon NB. Trisomy 1 in a clinically recognized IVF pregnancy. Am J Med Genet. 2001;99(2):152–3.
Banzai M, Sato S, Matsuda H, et al. Trisomy 1 in a case of a missed abortion. J Hum Genet. 2004;49(7):396–7.
Vicić A, Roje D, Strinić T, et al. Trisomy 1 in an early pregnancy failure. American J Med Genet Part A. 2008;146a(18):2439–41.
Forsberg LA, Gisselsson D, Dumanski JP. Mosaicism in health and disease - clones picking up speed. Nat Rev Genet. 2017;18(2):128–42.
Popovic M, Dhaenens L, Boel A, et al. Chromosomal mosaicism in human blastocysts: the ultimate diagnostic dilemma. Hum Reprod Update. 2020;26(3):313–34.
Malvestiti F, Agrati C, Grimi B, et al. Interpreting mosaicism in chorionic villi: results of a monocentric series of 1001 mosaics in chorionic villi with follow-up amniocentesis. Prenat Diagn. 2015;35(11):1117–27.
Grati FR, Grimi B, Frascoli G, et al. Confirmation of mosaicism and uniparental disomy in amniocytes, after detection of mosaic chromosome abnormalities in chorionic villi. Europ J Hum Gen Ejhg. 2006;14(3):282–8.
Gu C, Li K, Li R, et al. Chromosomal aneuploidy associated with clinical characteristics of pregnancy loss. Front Genet. 2021;12:667697.
Ozawa N, Ogawa K, Sasaki A, et al. Maternal age, history of miscarriage, and embryonic/fetal size are associated with cytogenetic results of spontaneous early miscarriages. J Assist Reprod Genet. 2019;36(4):749–57.
Segawa T, Kuroda T, Kato K, et al. Cytogenetic analysis of the retained products of conception after missed abortion following blastocyst transfer: a retrospective, large-scale, single-centre study. Reprod Biomed Online. 2017;34(2):203–10.
Hassold T, Benham F, Leppert M. Cytogenetic and molecular analysis of sex-chromosome monosomy. Am J Hum Genet. 1988;42(4):534–41.
Simpson JL, Rechitsky S, Kuliev A. Before the beginning: the genetic risk of a couple aiming to conceive. Fertil Steril. 2019;112(4):622–30.
Nguyen B T, chang E J, bendikson K A. Advanced paternal age and the risk of spontaneous abortion: an analysis of the combined 2011–2013 and 2013–2015 National Survey of Family Growth. American J Obstet Gynecol. 2019;221(5):476.e1-.e7.
Jia CW, Wang L, Lan YL, et al. Aneuploidy in early miscarriage and its related factors. Chin Med J (Engl). 2015;128(20):2772–6.
Chen L, Wang L, Tang F, et al. Copy number variation sequencing combined with quantitative fluorescence polymerase chain reaction in clinical application of pregnancy loss. J Assist Reprod Genet. 2021;38(9):2397–404.
Coomarasamy A, Dhillon-Smith RK, Papadopoulou A, et al. Recurrent miscarriage: evidence to accelerate action. Lancet (London, England). 2021;397(10285):1675–82.
Qu S, Wang L, Cai A, et al. Exploring the cause of early miscarriage with SNP-array analysis and karyotyping. J Matern Fetal Neonatal Med. 2019;32(1):1–10.
Dotters-Katz SK, Kuller JA, Grace MR, et al. Management considerations for ongoing pregnancies complicated by trisomy 13 and 18. Obstet Gynecol Surv. 2016;71(5):295–300.
Kosiv KA, Mercurio MR, Carey JC. The common trisomy syndromes, their cardiac implications, and ethical considerations in care. Curr Opin Pediatr. 2023;35(5):531–7.
Silva C, Ferreira MC, Saraiva J, et al. Trisomy 18-when the diagnosis is compatible with life. Eur J Pediatr. 2022;181(7):2809–19.
Bull MJ, Trotter T, Santoro SL, et al. Health Supervision for Children and Adolescents With Down Syndrome. Pediatr. 2022;149(5). https://doi.org/10.1542/peds.2022-057010
Chen Q, Zhang H, Li X, et al. Sequential application of copy number variation sequencing and quantitative fluorescence polymerase chain reaction in genetic analysis of miscarriage and stillbirth. Mol Genet Genomic Med. 2023;11(8):e2187.
Reddy K S. A paternally inherited terminal deletion, del(8)(p23.1)pat, detected prenatally in an amniotic fluid sample: a review of deletion 8p23.1 cases. Prenat. Diagn. 1999;19(9):868–72.
Yi Z, Yingjun X, Yongzhen C, et al. Prenatal diagnosis of pure partial monosomy 18p associated with holoprosencephaly and congenital heart defects. Gene. 2014;533(2):565–9.
Mello CB, Bueno OFA, Benedetto LM, et al. Intellectual, adaptive and behavioural characteristics in four patients with 18p deletion syndrome. J Intellectual Disability Res JIDR. 2019;63(3):225–32.
Tang F, Zeng Y, Wang L, et al. Prenatal phenotype of Wolf-Hirschhorn syndrome: a case series and literature review. Mol Genet Genomic Med. 2023;11(6):e2155.
Holland P, Wildhagen M, Istre M, et al. Cri du chat syndrome patients have DNA methylation changes in genes linked to symptoms of the disease. Clin Epigenetics. 2022;14(1):128.
Zeng W, Qi H, Du Y, et al. Analysis of potential copy-number variations and genes associated with first-trimester missed abortion. Heliyon. 2023;9(8):e18868.
Chen Y, Bartanus J, Liang D, et al. Characterization of chromosomal abnormalities in pregnancy losses reveals critical genes and loci for human early development. Hum Mutat. 2017;38(6):669–77.
Download references
Acknowledgements
We are grateful to Berry Genomics Corporation (Beijing, China), for their expert technical assistance and data analysis (Yuanyuan Zhu, Yang Bai, Xiang Wang, Berry Genomics Corporation, Beijing 102200, China). We thank Shanghai NewCore Biotechnology Co., Ltd. ( https://www.bioinformatics.com.cn , last accessed on 20 Feb 2024) for providing data analysis and visualization support.
This work was supported in part by grants from the National Natural Science Foundation of China (Grant No. 81200354); the National Science and Technology Major Project of China (2017ZX09304022); and the National Key Research and Development Program of China (2018YFC1002900).
Author information
Authors and affiliations.
Department of Obstetrics & Gynecology, Tongji Hospital, Tongji Medical College, Huazhong University of Science and Technology, Jiefang Avenue 1095, Wuhan, 430030, Hubei, China
Yu Qin, Koksear Touch, Yanan Sun, Shunran Zhang, Jianli Wu, Yuanyuan Wu, Ling Feng, Suhua Chen & Juan Xiao
Department of Obstetrics, Maternal and Child Health Hospital of Hubei Province, Tongji Medical College, Huazhong University of Science and Technology, Wuhan, China
Menghan Sha
You can also search for this author in PubMed Google Scholar
Contributions
S.C. and J.X. participated in and designed the study. Y.Q., K.T., M.S., Y.S., S.Z., J.W., Y.W., and L.F. collected medical records and interpreted data. Y.Q. completed data organization and functional enrichment analysis as well as drafted the manuscript. K.T. completed further data analysis and processing. All authors read and approved the final version of the manuscript.
Corresponding authors
Correspondence to Suhua Chen or Juan Xiao .
Ethics declarations
Ethics approval.
The studies involving human participants were reviewed and approved by the guidance of the Ethics Committee of Tongji Hospital, Huazhong University of Science and Technology (TJ-IRB202303141). The patients provided their written informed consent to participate in this study.
Conflict of interest
The authors declare no competing interests.
Additional information
Publisher's note.
Springer Nature remains neutral with regard to jurisdictional claims in published maps and institutional affiliations.
Supplementary Information
Below is the link to the electronic supplementary material.
Supplementary file1 (XLSX 24 KB)
Supplementary file2 (xlsx 225 kb), supplementary file3 (xlsx 35 kb), supplementary file4 (xlsx 15 kb), supplementary file5 (xlsx 13 kb), supplementary file6 (xlsx 46 kb), supplementary file7 (xlsx 60 kb), rights and permissions.
Springer Nature or its licensor (e.g. a society or other partner) holds exclusive rights to this article under a publishing agreement with the author(s) or other rightsholder(s); author self-archiving of the accepted manuscript version of this article is solely governed by the terms of such publishing agreement and applicable law.
Reprints and permissions
About this article
Qin, Y., Touch, K., Sha, M. et al. The chromosomal characteristics of spontaneous abortion and its potential associated copy number variants and genes. J Assist Reprod Genet (2024). https://doi.org/10.1007/s10815-024-03119-4
Download citation
Received : 27 September 2023
Accepted : 02 April 2024
Published : 26 April 2024
DOI : https://doi.org/10.1007/s10815-024-03119-4
Share this article
Anyone you share the following link with will be able to read this content:
Sorry, a shareable link is not currently available for this article.
Provided by the Springer Nature SharedIt content-sharing initiative
- Chromosomal abnormalities
- Copy number variations
- Spontaneous abortion
- Genetic etiology
- Find a journal
- Publish with us
- Track your research
- Open access
- Published: 25 April 2024
Clinical impact of panel gene sequencing on therapy of advanced cancers of the digestive system: a retrospective, single center study
- Lena Dreikhausen 1 ,
- Anna Klupsch 1 ,
- Isabella Wiest 1 ,
- Qiyun Xiao 1 ,
- Nadine Schulte 1 ,
- Johannes Betge 1 , 2 , 3 , 6 ,
- Tobias Boch 4 , 6 ,
- Christoph Brochhausen 5 ,
- Timo Gaiser 5 ,
- Ralf-Dieter Hofheinz 3 , 4 ,
- Matthias Ebert 1 , 3 , 6 &
- Tianzuo Zhan 1 , 3 , 6
BMC Cancer volume 24 , Article number: 526 ( 2024 ) Cite this article
18 Accesses
Metrics details
Panel gene sequencing is an established diagnostic tool for precision oncology of solid tumors, but its utility for the treatment of cancers of the digestive system in clinical routine is less well documented.
We retrospectively identified patients with advanced or metastatic gastrointestinal, pancreaticobiliary or hepatic cancers who received panel gene sequencing at a tertiary university hospital from 2015 to 2022. For these cases, we determined the spectrum of genetic alterations, clinicopathological parameters and treatment courses. Assessment of actionability of genetic alterations was based on the OncoKB database, cancer-specific ESMO treatment guidelines, and recommendations of the local molecular tumor board.
In total, 155 patients received panel gene sequencing using either the Oncomine Focus (62 cases), Comprehensive (91 cases) or Childhood Cancer Research Assay (2 cases). The mean age of patients was 61 years (range 24–90) and 37% were female. Most patients suffered from either colorectal cancer (53%) or cholangiocellular carcinoma (19%). 327 genetic alterations were discovered in 123 tumor samples, with an average number of 2.1 alterations per tumor. The most frequently altered genes were TP53, KRAS and PIK3CA . Actionable gene alterations were detected in 13.5–56.8% of tumors, according to ESMO guidelines or the OncoKB database, respectively. Thirteen patients were treated with targeted therapies based on identified molecular alterations, with a median progression-free survival of 8.8 months.
Conclusions
Actionable genetic alterations are frequently detected by panel gene sequencing in patients with advanced cancers of the digestive tract, providing clinical benefit in selected cases. However, for the majority of identified actionable alterations, sufficient clinical evidence for targeted treatments is still lacking.
Peer Review reports
Introduction
Cancers of the digestive system represent a major fraction of the global tumor burden [ 1 , 2 ]. Despite their high incidence, the therapeutic options for most advanced and metastatic cancers of the digestive system are still limited. Molecular profiling approaches, in particular next-generation tumor genome sequencing, hold the promise of identifying alterations that can be exploited for cancer therapy. This concept of personalized oncology is supported by several large prospective clinical trials showing that actionable mutations can be detected in in 36.7–58.2% of patients with solid cancers [ 3 , 4 ]. Furthermore, results of these studies indicate that genomics-driven cancer therapy can improve overall survival and reduce toxicity-related mortality [ 5 ]. Hence, precision oncology approaches have been integrated into routine oncological care in many academic medical centers. Retrospective real-world data from these centers suggest that actionable mutations can be detected in 20–40% of cancer patients [ 6 , 7 ], matching findings from prospective studies [ 3 , 4 ]. For cancers of the digestive systems, studies have reported that actionable genetic alterations can be found in 5.8 to 27.8% of cases, with highest rates in cholangiocellular and gastroesophageal cancers, and lowest rates in pancreatic cancer [ 8 ]. Different methods for high-throughput DNA profiling have been applied for precision oncology, ranging from whole genome/exome sequencing to more focused approaches such as panel gene sequencing. While mutational profiling by whole genome/exome sequencing is comprehensive, it is also associated with higher costs and requires more extensive bioinformatic resources for analysis compared to more focused sequencing approaches [ 9 ]. In contrast, gene panel sequencing is versatile and less costly, but allows only the assessment of hotspot mutational sites in predefined sets of genes [ 9 ]. In clinical practice, translation of cancer genomics into novel therapeutic options for patients is frequently hindered by a lack of access to suitable clinical trials, delayed coverage of therapy cost by insurance companies or rapid disease progression [ 10 ]. Thus, many studies indicate that only a minor fraction of patients with actionable genetic alteration benefit from alteration-specific therapies [ 6 , 11 ]. While the real-life clinical utility of panel gene sequencing has been evaluated across a broad spectrum of solid tumors [ 12 , 13 ], few studies focused on cancers of the digestive system.
In this retrospective study, we determined the results of panel gene sequencing in 155 patients with locally advanced or metastatic cancers of the digestive system who were treated at a tertiary academic medical center. We describe the spectrum of identified mutations, subsequent mutation-specific therapeutic approaches, and clinical courses of the patients receiving tailored therapies.
Collection of clinical data
Patient selection was performed by a database query of the local data integration center of the Mannheim University Hospital. All histopathological reports generated by the Institute of Pathology of Mannheim University Hospital between 01/2015 and 03/2022 were searched for documentation of the term “oncomine”, which comprises the following panel gene sequencing assays: Oncomine Focus Assay, Oncomine Comprehensive Assay and Oncomine Childhood Cancer Research Assay. Among all identified patients, those who were >18 years and encoded with the following ICD codes were selected for analysis: malignant neoplasm of the esophagus (C15), stomach (C16), small intestine (C17), colon (C18), rectosigmoid junction (C19), rectum (C20), anus and anal canal (C21), liver and intrahepatic bile ducts (C22), gallbladder (C23), biliary tract (C24), pancreas (C25) and other imprecisely defined digestive organs (C26). Next, only patients with locally advanced or metastatic cancers who required systemic therapies were included for in-detail review. A detailed list of all included cancer entities can be found in Supplementary Table 1 . For this patient cohort, we obtained information on demographic parameters (age, sex), cancer entity and stage, lines of systemic therapy and progression-free survival (PFS). PFS was defined as time from initiation of molecular-targeted therapy until radiological disease progression or time of data cut-off (31.12.2022).
Collection of panel sequencing and pathology data
From the panel gene sequencing reports, we obtained the following data: type of assay, number, and types of identified genetic alterations, allele frequency and assessment of pathogenicity. Three panel sequencing assay types were applied in our cohort: the Oncomine Focus panel covers 52, the Comprehensive Panel v3 161 and Childhood Cancer Research Panel 203 unique cancer-related genes. Assay types and versions did not change during the observation period. A list of individual genes that are covered by the respective assays can be found in Supplementary Table 2 . For sequencing, fresh-frozen paraffin-embedded (FFPE) tissue samples were used in all cases. The minimum input of genomic DNA was 10 ng and sequencing was performed using a combination of Thermo Fisher Ion GeneStudio S5 and Ion Chef System.
Furthermore, we collected information on complementary immunohistochemical markers including Her2 amplification and deficient mismatch repair (dMMR) from routine pathology reports. dMMR was determined by immunohistochemical staining of MLH1, PMS2, MSH2 and MSH6. Tumors with loss of expression of at least one of these proteins were considered as dMMR.
Assessment of actionability of genetic alterations
Actionability of genetic alterations was retrospectively assessed on a tumor entity level using the OncoKB database [ 14 ] and the ESMO guidelines for the management of colorectal, gastric, esophageal, pancreatic, biliary and hepatocellular cancers [ 15 , 16 , 17 , 18 , 19 , 20 ]. Clinical evidence supporting actionability of mutations was categorized using the OncoKB score or the ESMO-ESCAT score [ 21 ] as proposed in the ESMO guidelines. Furthermore, we included recommendations of the local molecular tumor board if available. Assessment of actionability by the molecular tumor board was based on the NCT/DKTK classification [ 22 ]. A detailed explanation of the scoring system of the three classifiers can be found in Supplementary Table 3 .
Statistical analysis
The Wilcoxon-rank sum test was used to compare number of detected mutations between different panel sequencing assay types. All figures were generated using Graph Pad Prism, version 9.
Clinico-pathological characteristics of cancer patients
We retrospectively identified a total of 155 patients who were treated for locally advanced or metastatic cancers of the digestive system and received in-house panel gene sequencing. Median age of this cohort was 61 years (range 24–90 years) and 98 (63%) patients were male. The most frequent cancer entities were colorectal cancers (CRC, 83 cases), followed by cholangiocellular carcinomas (CCC, 29 cases) and esophageal/esophagogastric junction/gastric cancers (15 cases) (Fig. 1 A). Rare cancer types in this cohort included one case of duodenal adenocarcinoma, one of neuroendocrine carcinoma of the esophagogastric junction, one of neuroendocrine tumor of the midgut, one of small bowel adenocarcinoma, two of ampullary cancers, two of mixed hepatocellular-cholangiocellular carcinomas and three of cancers of unknown primary (total 11 cases).
Median time from first diagnosis of the disease to panel gene sequencing was 23.4 months, with the longest median time periods for patients with hepatocellular carcinoma (34.8 months). For panel sequencing, biopsy material from either metastatic lesions (68 cases) or primary tumors (86 cases) was used. In one case, biopsy from a local tumor recurrence was analyzed. The majority of biopsies was obtained during diagnosis of the disease. For most cancer types, panel gene sequencing was performed during first or second-line therapy, whereas it was performed during third-line therapy in patients with hepatocellular carcinoma (HCC).
The most frequently applied panel gene sequencing assay was Oncomine Comprehensive Assay v3 (91), followed by Oncomine Focus Assay (62) and Oncomine Childhood Cancer Research Assay (2) (Fig. 1 B). Selection of assay type was based on individual oncologists’ preferences. Distribution of assay types was not balanced between tumor entities. The Oncomine Focus Panel was most frequently used in CRC (40/83) while the Oncomine Comprehensive Panel was mostly applied in cholangiocellular carcinoma (16/29), esophagogastric junction cancer (9/15), pancreatic adenocarcinoma (12/13), and hepatocellular carcinoma (4/4).
Data on complementary predictive immunohistochemical markers such as Her2 amplification and microsatellite instability were collected from routine pathology reports. Her2 expression status was available from 55 patients and was negative in all cases. Mismatch repair deficiency was assessed in 128 cases and could be detected in tumors of five patients with CRC, one with CCC and one with duodenal adenocarcinoma. Clinico-pathological characteristics are displayed in Table 1 .
Spectrum of genetic alterations determined by panel gene sequencing
Next, we determined the spectrum of genetic alterations that was detected by panel gene sequencing. On average, 2.1 alterations were detected per tumor sample (range 0–7). No genetic alterations were detected in 32 cases, of which 29 used the Oncomine Focus panel (Fig. 1 B). We observed a significant difference in median number of detected alterations between assay types (Oncomine Focus Assay: 0.7 per sample, Oncomine Comprehensive Assay: 3.0 per sample, Fig. 1 C). Between tumor entities, the mean number of detected alterations also differed (e.g. 1.8 alterations per sample in CCC versus 3.1 alterations in pancreatic adenocarcinoma). In total, 327 genetic alterations were detected, of which 191 were classified as pathogenic or likely pathogenic by the Oncomine database. Most genetic alterations were gene mutations (89.3% of all alterations), while copy number changes (7%), gene fusions (1.5%), splice site alterations (1.8%) and translocations (0.3%) were detected in a minority of cases (Fig. 1 D). Mutations were predominantly detected in TP53 (61), KRAS (47), PIK3CA (17), BRAF (11) and FBXW7 (10). The most common single mutations were KRAS G12D (14), KRAS G12V (12), BRAF V600E (9) and KRAS G13D (6), predominantly detected in CRC and pancreaticobiliary cancers (Fig. 1 E and F). Average allele frequency of detected mutations was 38% (range: 3.85–100%). Twenty-three copy number changes were detected in 4 cancer entities (CRC, hepatocellular carcinoma, esophagogastric cancer and pancreatic adenocarcinoma). The most common copy number changes were observed in MYC (3) and EGFR (2). Gene fusions detected include PTPRK-RSPO3 (3), GOPC-ROS1 (1) and BRD4-PPARG (1), all observed in CRC. Splice site alterations were found in RICTOR in hepatocellular carcinoma and ampullary cancer (2), as well as in CDKN2A in CCC (1) and in PMS2 (1), TP53 (1) and FBXW7 (1) in CRC.
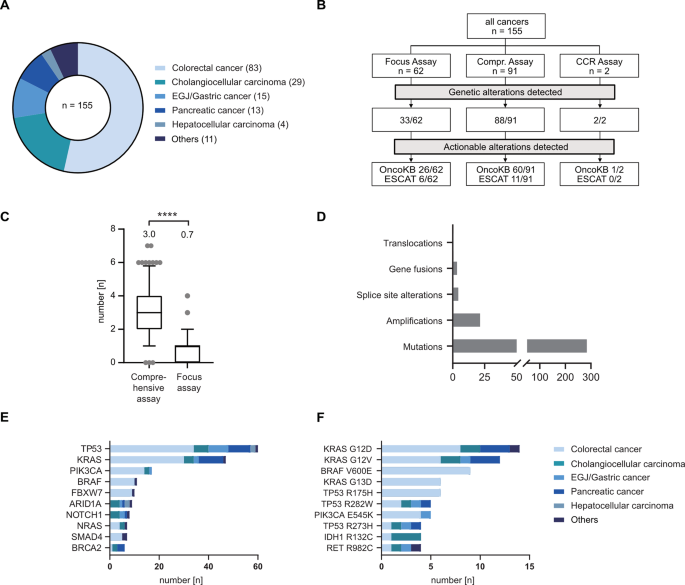
Spectrum of cancer mutations detected by panel gene sequencing. ( A ) Pie chart showing distribution of cancer entities in panel gene sequencing cohort. ( B ) Flowchart showing distribution of assay types, and the fraction of assays that detected genetic alterations or actionable alterations. Actionability of genetic alterations were either classified using the OncoKB database (“OncoKB”) or the ESMO guidelines (“ESCAT”). ( C ) Frequency of detected mutations between assay types are shown as box plots with whiskers indicating 10/90 percentiles. The mean number of genetic alterations per assay is shown above the box plots. Statistical testing was done using a Wilcoxon-rank sum test. **** indicates p < 0.001. ( D ) Bar chart showing distribution of different genetic alterations in the cohort. ( E-F ) Bar chart showing the ten most frequently altered genes ( E ) and the most frequent genetic alterations ( F ). Abbreviations : CCR– Childhood Cancer Research (Assay)
Actionability of genetic alterations detected by panel gene sequencing
We then determined the proportion of genetic alterations for which genomics-directed therapies have been described, by using different resources. First, we used the publicly accessible OncoKB precision oncology database [ 14 ]. Here, actionable alterations are assigned to four levels of evidence, based on published clinical and preclinical studies (see Supplementary Table 3 for explanation of scores). Using the OncoKB database, 35.8% (117/327) of all identified alterations were classified as actionable. Classifications of actionable alterations were as follows: 10% were assigned to level 1, 2% to level 3A, 38% to level 3B and 50% to level 4. We observed a difference in the frequency of detected, actionable alterations between assay types, with 66% of all Oncomine Comprehensive Assays and 42% of all Oncomine Focused Assays detecting at least one actionable mutation.
Next, we evaluated actionability of genetic alterations using the ESMO guidelines for gastrointestinal, hepato- and pancreaticobiliary cancers [ 15 , 16 , 17 , 18 , 19 , 20 ]. In the ESMO guidelines, the ESMO-ESCAT classification was used to assess the clinical evidence underlying actionable genetic alterations [ 21 ] (see Supplementary Table 3 ). In total, only 5.5% (18/327) of alterations were considered as actionable by the ESMO guidelines, with 14 classified as I-A and 4 as III-A by the ESMO-ESCAT score. Except for the BRCA K3326* mutation, all other alterations were also classified as actionable by OncoKB. Deficient mismatch repair (dMMR) is considered as an actionable alteration with high level of evidence by both the OncoKB database and ESMO guidelines. After including 7 cases with dMMR, actionable alterations were found in 56.8% (88/155) and 13.5% (21/155) of tumors/patients, using OncoKB and ESMO guidelines respectively (Fig. 2 A-B). Actionable alterations with highest levels of evidence (OncoKB level 1 or ESMO-ESCAT I-A) occurred most frequently in CRC and CCC, and included dMMR, BRAF V600E , and IDH R132C (Fig. 2 C and D). In many tumors, multiple actionable alterations were detected (most frequently KRAS plus PIK3CA mutations or dMMR plus BRAF V600E in CRC). Only one druggable gene translocation was identified in our cohort (ELM4-ALK2 in colorectal cancer, ESMO-ESCAT level III-A).
We also reviewed recommendations by the local molecular tumor board which was introduced at our university hospital in 2020. A total of 31 cases from our cohort were presented in the molecular tumor board and actionable alterations could be identified in 17 tumors/patients. In accordance with national guidelines [ 23 ], the most frequently presented tumor entity in the molecular tumor board was CCC. Actionability of mutations was most frequently identified for the following genes: ARID1A , ERBB3, SMARCA4 and PIK3CA . A list of all identified actionable alterations and their recommended drugs is presented in Supplementary Table 4 .
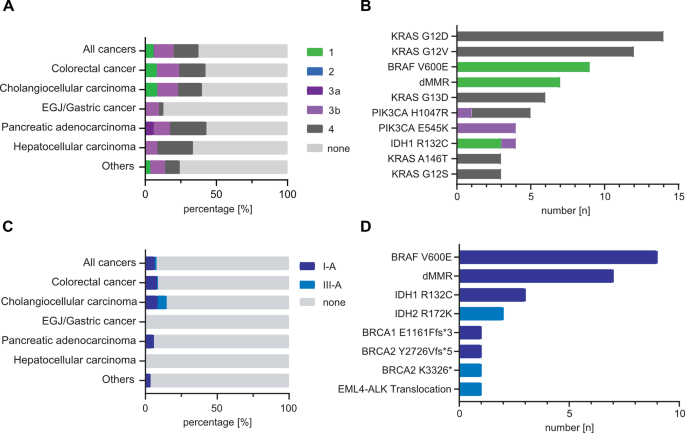
Spectrum of detected actionable genetic alterations. ( A - B ) Bar chart showing the fraction of actionable mutations per cancer entity ( A ) and ten most frequent actionable genetic alterations ( B ), as assessed and classified using the OncoKB database. ( C - D ) Bar chart showing the fraction of actionable mutations per cancer entity ( C ) and all actionable genetic alterations ( D ), as assessed by the EMSO treatment guidelines for gastrointestinal, hepato- and pancreaticobiliary cancers and classified by the ESCAT score
Clinical courses of cancer patients with actionable alterations
A total of 13 patients received one or more tailored therapies, including seven patients with CRC, four with CCC, one with duodenal adenocarcinoma and one with pancreatic adenocarcinoma (Table 2 ). Except for two cases, all actionable alterations belonged to the highest evidence levels (OncoKB evidence level 1, ESMO-ESCAT level I-A). The median number of preceding lines of therapy in this cohort was 1 (range 0–4). The most frequent alterations were dMMR (6), treated with pembrolizumab or nivolumab/ipilimumab, and BRAF V600E (4), treated with encorafenib/cetuximab +/- binimetinib. We also found two patient cases of IDH1 R132C mutations in CCC, who were treated with the IDH1 inhibitor ivosidenib. At data cut-off (December 2022), median PFS of this patient cohort was 8.8 months (range 2.3–67.3 months) and in 6 patients, biomarker-directed therapy was ongoing. Best responses to therapy were one case with complete response, four with partial response, three with mixed response, four with stable disease and one with progressive disease. PFS was longest in patients with dMMR CRC receiving pembrolizumab (median 12.4 months, four patients) and shortest in patients with IDH1 R132C mutant CCC who were treated with ivosidenib (median 2.4 months, two patients).
Two patients received therapies following the recommendations of the local molecular tumor board, as mutations with only low or no levels of evidence for actionability were detected ( BRCA2 S497L in pancreatic adenocarcinoma; ERBB3 G284R in CCC). The patient with pancreatic adenocarcinoma presented with a germline loss-of-function mutation of BRCA1 ( E1161Ffs*3 ) and germline mutation of unknown significance in BRCA2 ( S497L ). Two months after completion of neoadjuvant chemotherapy with eight cycles of FOLFIRINOX, pancreaticoduodenectomy was performed. Subsequently, the patient developed recurrent and metastatic disease which progressed under six cycles of treatment with gemcitabine/nab-paclitaxel. Therapy was switched to 5-fluorouracil/folic acid/nanoliposomal irinotecan but had to be discontinued due to uncontrollable diarrhoea and fatigue, despite radiological partial disease response. Due to severe neuropathy, re-induction with platin-based chemotherapy regimens was not possible. He then received olaparib monotherapy based on recommendations of the molecular tumor board, with an ongoing treatment response of nine months at data cut-off. The patient with CCC and ERBB3 G284R mutation received a combination therapy with lapatinib and trastuzumab after disease progression under treatment with gemcitabine/cisplatin, with an ongoing disease control for 7.3 months.
This retrospective, real-world analysis of 155 patients with advanced cancers of the digestive system shows that gene panel sequencing can uncover actionable genetic alterations that result in individualized therapeutic options.
In our patient cohort, actionable molecular alterations were observed in 13.5–56.8% of cases, using the ESMO treatment guidelines or OncoKB database, respectively. When using OncoKB as reference, our number of detected actionable alterations is comparable to published, prospective studies that used panel gene sequencing and reported frequencies between 36.7 and 58.2% [ 3 , 4 ]. However, significantly fewer actionable alterations were detected when the ESMO treatment guidelines were applied.
In general, our study shows that the frequency of identified actionable alterations was dependent on two main factors: the genomic coverage of the assay type and the classifier used. In 29 out of 32 cases in which no mutations could be detected, a focused panel gene sequencing assay was used. Similarly, the percentage of tumors with actionable mutations, as classified by the OncoKB database, was significantly lower when the Oncomine Focused assay was used (Fig. 2 B). These findings indicate that to maximize the clinical utility of gene panel sequencing, comprehensive gene panels are needed.
Secondly, we observed that the number of actionable alterations significantly differed between the OncoKB database and ESMO treatment guidelines. We found a major overlap between the two resources for actionable alterations with high levels of clinical evidence, such dMMR or BRAF V600E . However, the OncoKB database also includes many actionable genetic alterations with low levels of clinical evidence which were not evaluated by the ESMO guidelines and thus not assigned an ESMO-ESCAT score. This difference in classification is most apparent for alterations in KRAS , which was the second most frequently mutated gene in our cohort. While OncoKB suggested MEK inhibitors as a treatment for KRAS mutant cancers, most early clinical trials did not find any clinical benefit of MEK inhibitor monotherapy in gastrointestinal cancers [ 24 ]. In contrast, only KRAS G12C was classified as an actionable target by the ESMO guidelines, which is supported by several clinical trials [ 25 , 26 , 27 ]. Overall, KRAS G12C mutations are rare in cancers of the digestive tract and we only detected one case in a patient with pancreatic cancer. For this patient, sotorasib was recommended by the molecular tumor board, but treatment with olaparib was initiated due to concurrent BRCA1/2 mutations. However, as other genotype-specific or pan-KRAS inhibitors are currently undergoing (pre-)clinical testing [ 28 ], the importance of KRAS mutations as an actionable target will steadily increase.
In general, it is challenging to compare the frequencies of detected actionable alterations between panel gene sequencing studies, as the actionability can be assessed by many different approaches. Besides the OncoKB database, several other public databases (e.g. Clinical Interpretation of Variants in Cancer [ 29 ], Cancer Genome Interpreter [ 30 ]), and commercial resources (e.g. Jackson Laboratory Clinical Knowledge Base [ 31 ]) exist that assess actionability of genetic alterations. Depending on the evidencelevels that are defined as thresholds by these databases and the depth of literature research, the actionability of genetic alterations might be assessed differently. Furthermore, in many past studies, treatment selection was guided by the recommendations of the local molecular tumor boards or clinical experts [ 32 , 33 ], providing another layer of heterogeneity. To reduce this heterogeneity of recommendations, an important step would be to establish publicly accessible, transparent databases that collect clinical and pre-clinical evidence for molecular-directed therapies. These databases would enable a stronger unification of treatment recommendations, especially for druggable mutations that occur at low frequencies and for which clinical evidence is rare. An ongoing data curation would be necessary as well as regular validation of recommendations by expert panels.
Lastly, the frequency of actionable alterations also depends on the composition of tumor entities in the respective cohorts. Several recent retrospective studies have shown that panel sequencing can uncover actionable mutations across different solid tumors at high rates [ 12 , 13 ]. However, cancers of the digestive system only account for a small fraction in these studies and the frequency of actionable alterations is less clear in this subgroup. The large proportion of CRC and CCC in our cohort might introduce a bias towards cancer types of the digestive system that have a comparatively high frequency of actionable mutations.
Due to its retrospective design, our study has some limitations. First, we included three panel gene sequencing assay types which differed in their coverage of genetic alterations. We also observed differences in the usage of these assays depending on the tumor entity. The selection of the assay type was not based on predefined algorithms but rather on individual clinical decisions. Thus, comparing frequencies of specific mutations between tumor types must be regarded with care, as the data might be biased by differential selection of panel sequencing assays. To overcome this problem in clinical practice, guidelines that clearly define the optimal time point and assay type for specific tumor entities should be implemented. Secondly, performance of panel gene sequencing assays depends on the quality of the tissue material [ 34 ]. In some cases, FFPE tissue from primary cancers were used that have been stored over longer time periods. This might affect the quality of DNA and specifically of RNA, leading to lower sensitivity for specific alterations such as gene fusions [ 35 ]. Lastly, our data indicates that those patients who received a genomics-directed therapy had an overall high clinical benefit. However, this observation is biased by the large proportion of patients with dMMR CRC who received immune checkpoint inhibitors which are highly effective in this tumor subtype [ 36 ]. In contrast, the clinical benefit of ivosidenib in CCC (median PFS 2.4 months in two patients) or cetuximab/encorafenib in CRC (median PFS 3.4 months in 4 four patients) was less pronounced, but matching results of the respective trials (median PFS of 2.7 months for ivosidenib [ 37 ]and median PFS 4.2 for cetuximab/encorafenib [ 38 ].
Despite these limitations, our study shows that selected patients can benefit greatly from molecular-directed therapies. We report two cases of mutations with low evidence for actionability based on the OncoKB database ( BRCA2 S497L and ERBB3 G284R ), but for which our local molecular tumor board suggested therapeutic options that led to sustained tumor responses. Based on the results of the POLO trial, patients with pancreatic carcinoma with germline loss-of-function BRCA2 mutations benefit from olaparib maintenance therapy following disease control with first-line oxaliplatin-based chemotherapy regimen [ 39 , 40 ]. While the trial only included deleterious and likely deleterious germline mutations, the clinical impact of non-synonymous germline mutations such as BRCA2 S497L remain unknown [ 41 ]. Here, we report the case of a patient with metastatic pancreatic carcinoma with a combination of germline BRCA1 E1161Ffs*3 and BRCA2 S497L mutations that benefit from treatment with olaparib monotherapy after failure of several lines of preceding systemic therapies. Missense mutations of BRCA2 can moderately increase the risk for breast cancer [ 42 ], but it is unknown if they also modulate the response to PARP inhibitors. Considering the durable response that we observed in our patient case, further preclinical studies should be performed to decipher the actionability of BRCA2 missense mutations. ERBB3 mutations can occur in various cancer entities, but are overall rare events [ 43 ]. Mutations of ERBB3 can induce activation of the MAPK pathway, and a previous case report showed that targeting ERBB3 G284R mutant breast cancer with trastuzumab plus lapatinib can lead to disease control [ 44 ]. The ERBB3 G284R mutation is considered an activating alteration [ 45 ], and to our knowledge, our case shows for the first time that treatment with trastuzumab/lapatinib can result in prolonged disease control in metastatic CCC with this specific ERBB3 mutation.
In summary, our study shows that panel gene sequencing can reveal actionable genetic alterations in cancers of the digestive tract, with clinical benefit for selected patients in real-life. The utility of panel gene sequencing depends on the genomic coverage of the panel and comprehensive gene panels should be used. In-depth assessment of identified mutations by molecular tumor boards can identify novel actionable genetic alterations that are not enlisted in public precision medicine databases.
Data availability
No datasets were generated or analysed during the current study.
Abbreviations
Cholangiocellular carcinoma
Colorectal cancer
ESMO Scale for Clinical Actionability of Molecular Targets
European Society For Medical Oncology
Fresh-frozen paraffin-embedded
Hepatocellular carcinoma
National Center for Tumor Diseases/Deutsches Konsortium für Translationale Krebsforschung (German Cancer Consortium)
Mismatch repair deficiency
Progression-free Survival
Arnold M, Abnet CC, Neale RE, Vignat J, Giovannucci EL, McGlynn KA, et al. Global burden of 5 major types of gastrointestinal Cancer. Gastroenterology. 2020;159:335–e34915.
Article PubMed Google Scholar
Sung H, Ferlay J, Siegel RL, Laversanne M, Soerjomataram I, Jemal A, et al. Global Cancer statistics 2020: GLOBOCAN estimates of incidence and Mortality Worldwide for 36 cancers in 185 countries. CA Cancer J Clin. 2021;71:209–49.
Zehir A, Benayed R, Shah RH, Syed A, Middha S, Kim HR, et al. Mutational landscape of metastatic cancer revealed from prospective clinical sequencing of 10,000 patients. Nat Med. 2017;23:703–13.
Article CAS PubMed PubMed Central Google Scholar
Sunami K, Ichikawa H, Kubo T, Kato M, Fujiwara Y, Shimomura A, et al. Feasibility and utility of a panel testing for 114 cancer-associated genes in a clinical setting: a hospital-based study. Cancer Sci. 2019;110:1480–90.
Schwaederle M, Zhao M, Lee JJ, Eggermont AM, Schilsky RL, Mendelsohn J, et al. Impact of precision medicine in diverse cancers: a meta-analysis of phase II clinical trials. J Clin Oncol. 2015;33:3817–25.
Bregni G, Sticca T, Camera S, Akin Telli T, Craciun L, Trevisi E, et al. Feasibility and clinical impact of routine molecular testing of gastrointestinal cancers at a tertiary centre with a multi-gene, tumor-agnostic, next generation sequencing panel. Acta Oncol (Madr). 2020;59:1438–46.
Article CAS Google Scholar
Takeda H, Imoto K, Umemoto K, Doi A, Arai H, Horie Y, et al. Clinical utility of genomic profiling tests in patients with Advanced gastrointestinal cancers. Target Oncol. 2022;17:177–85.
Flaherty KT, Gray RJ, Chen AP, Li S, McShane LM, Patton D, et al. Molecular landscape and actionable alterations in a genomically guided cancer clinical trial: national cancer institute molecular analysis for therapy choice (NCI-MATCH). J Clin Oncol. 2020;38:3883–94.
Morganti S, Tarantino P, Ferraro E, D’Amico P, Viale G, Trapani D, et al. Complexity of genome sequencing and reporting: next generation sequencing (NGS) technologies and implementation of precision medicine in real life. Crit Rev Oncol/Hematol. 2019;133:171–82.
Inagaki C, Maeda D, Hatake K, Sato Y, Hashimoto K, Sakai D, et al. Clinical utility of next-generation sequencing‐based panel testing under the universal health‐care system in Japan: a retrospective analysis at a single university hospital. Cancers (Basel). 2021;13:1–14.
Article Google Scholar
Lamarca A, Kapacee Z, Breeze M, Bell C, Belcher D, Staiger H, et al. Molecular profiling in daily clinical practice: practicalities in advanced cholangiocarcinoma and other biliary tract cancers. J Clin Med. 2020;9:1–18.
Cobain EF, Wu YM, Vats P, Chugh R, Worden F, Smith DC, et al. Assessment of Clinical Benefit of Integrative genomic profiling in Advanced Solid tumors. JAMA Oncol. 2021;7:525–33.
PubMed Google Scholar
Vingiani A, Agnelli L, Duca M, Lorenzini D, Damian S, Proto C et al. Molecular Tumor Board as a clinical Tool for converting Molecular Data Into Real-World patient care. JCO Precis Oncol. 2023;7.
Chakravarty D, Gao J, Phillips S, Kundra R, Zhang H, Wang J, et al. OncoKB: a Precision Oncology Knowledge Base. JCO Precis Oncol. 2017;2017:1–16.
Conroy T, Pfeiffer P, Vilgrain V, Lamarca A, Seufferlein T, O’Reilly EM, et al. Pancreatic cancer: ESMO Clinical Practice Guideline for diagnosis, treatment and follow-up†. Ann Oncol. 2023. https://doi.org/10.1016/j.annonc.2023.08.009 .
Obermannová R, Alsina M, Cervantes A, Leong T, Lordick F, Nilsson M, et al. Oesophageal cancer: ESMO Clinical Practice Guideline for diagnosis, treatment and follow-up. Ann Oncol. 2022;33:992–1004.
Lordick F, Carneiro F, Cascinu S, Fleitas T, Haustermans K, Piessen G, et al. Gastric cancer: ESMO Clinical Practice Guideline for diagnosis, treatment and follow-up. Ann Oncol. 2022;33:1005–20.
Article CAS PubMed Google Scholar
Vogel A, Cervantes A, Chau I, Daniele B, Llovet J, Meyer T, et al. Hepatocellular carcinoma: ESMO Clinical Practice guidelines for diagnosis, treatment and follow-up. Ann Oncol. 2018;29(Suppl 4):iv238–55.
Cervantes A, Adam R, Roselló S, Arnold D, Normanno N, Taïeb J, et al. Metastatic colorectal cancer: ESMO Clinical Practice Guideline for diagnosis, treatment and follow-up ☆ . Ann Oncol. 2023;34:10–32.
Vogel A, Bridgewater J, Edeline J, Kelley RK, Klümpen HJ, Malka D, et al. Biliary tract cancer: ESMO Clinical Practice Guideline for diagnosis, treatment and follow-up ☆ . Ann Oncol. 2023;34:127–40.
Mateo J, Chakravarty D, Dienstmann R, Jezdic S, Gonzalez-Perez A, Lopez-Bigas N, et al. A framework to rank genomic alterations as targets for cancer precision medicine: the ESMO scale for clinical actionability of molecular targets (ESCAT). Ann Oncol. 2018;29:1895–902.
Lier A, Penzel R, Heining C, Horak P, Fröhlich M, Uhrig S, et al. Validating Comprehensive Next-Generation sequencing results for Precision Oncology: the NCT/DKTK molecularly aided stratification for Tumor Eradication Research Experience. JCO Precis Oncol. 2018;2:1–13.
Groβ S, Bitzer M, Blödt S, Follmann M, Langer T, Freudenberger P, et al. Leitlinienreport Der S3-Leitlinie Diagnostik Und Therapie Des Hepatozellulären Karzinoms und biliärer Karzinome - Version 3.0 - Juli 2022, AWMF-Registernummer: 032/053OL. Z Gastroenterol. 2022;61:E157–71.
Google Scholar
Infante JR, Fecher LA, Falchook GS, Nallapareddy S, Gordon MS, Becerra C, et al. Safety, pharmacokinetic, pharmacodynamic, and efficacy data for the oral MEK inhibitor trametinib: a phase 1 dose-escalation trial. Lancet Oncol. 2012;13:773–81.
Sacher A, LoRusso P, Patel MR, Miller WH, Garralda E, Forster MD, et al. Single-Agent Divarasib (GDC-6036) in solid tumors with a KRAS G12C mutation. N Engl J Med. 2023;389:710–21.
Strickler JH, Satake H, George TJ, Yaeger R, Hollebecque A, Garrido-Laguna I, et al. Sotorasib in KRAS p.G12C-Mutated Advanced Pancreatic Cancer. N Engl J Med. 2023;388:33–43.
Yaeger R, Weiss J, Pelster MS, Spira AI, Barve M, Ou S-HI, et al. Adagrasib with or without Cetuximab in Colorectal Cancer with Mutated KRAS G12C. N Engl J Med. 2023;388:44–54.
Kim D, Herdeis L, Rudolph D, Zhao Y, Böttcher J, Vides A, et al. Pan-KRAS inhibitor disables oncogenic signalling and tumour growth. Nature. 2023;619:160–6.
Griffith M, Spies NC, Krysiak K, McMichael JF, Coffman AC, Danos AM, et al. CIViC is a community knowledgebase for expert crowdsourcing the clinical interpretation of variants in cancer. Nat Genet. 2017;49:170–4.
Tamborero D, Rubio-Perez C, Deu-Pons J, Schroeder MP, Vivancos A, Rovira A et al. Cancer Genome Interpreter annotates the biological and clinical relevance of tumor alterations. Genome Med. 2018;10.
Patterson SE, Liu R, Statz CM, Durkin D, Lakshminarayana A, Mockus SM. The clinical trial landscape in oncology and connectivity of somatic mutational profiles to targeted therapies. Hum Genomics. 2016;10.
Welland S, DeCastro T, Bathon M, Wirth TC, Reineke-Plaaß T, Saborowski M, et al. Molecular diagnostics and therapies for gastrointestinal tumors: a real-world experience. J Cancer Res Clin Oncol. 2022;148:2137–44.
Dhir M, Choudry HA, Holtzman MP, Pingpank JF, Ahrendt SA, Zureikat AH, et al. Impact of genomic profiling on the treatment and outcomes of patients with advanced gastrointestinal malignancies. Cancer Med. 2017;6:195–206.
Agrawal L, Engel KB, Greytak SR, Moore HM. Understanding preanalytical variables and their effects on clinical biomarkers of oncology and immunotherapy. Sem Cancer Biol. 2018;52:26–38.
von Ahlfen S, Missel A, Bendrat K, Schlumpberger M. Determinants of RNA quality from FFPE samples. PLoS ONE. 2007;2.
André T, Shiu K-K, Kim TW, Jensen BV, Jensen LH, Punt C, et al. Pembrolizumab in microsatellite-instability–high Advanced Colorectal Cancer. N Engl J Med. 2020;383:2207–18.
Abou-Alfa GK, Macarulla T, Javle MM, Kelley RK, Lubner SJ, Adeva J, et al. Ivosidenib in IDH1-mutant, chemotherapy-refractory cholangiocarcinoma (ClarIDHy): a multicentre, randomised, double-blind, placebo-controlled, phase 3 study. Lancet Oncol. 2020;21:796–807.
Kopetz S, Grothey A, Yaeger R, Van Cutsem E, Desai J, Yoshino T, et al. Encorafenib, binimetinib, and cetuximab in BRAF V600E–mutated colorectal cancer. N Engl J Med. 2019;381:1632–43.
Kindler HL, Hammel P, Reni M, Van Cutsem E, Macarulla T, Hall MJ, et al. Overall survival results from the POLO trial: a phase III study of active maintenance Olaparib Versus Placebo for Germline BRCA-Mutated metastatic pancreatic Cancer. J Clin Oncol. 2022;40:3929–39.
Golan T, Hammel P, Reni M, Van Cutsem E, Macarulla T, Hall MJ, et al. Maintenance olaparib for germline BRCA -Mutated metastatic pancreatic Cancer. N Engl J Med. 2019;381:317–27.
Rebbeck TR, Friebel TM, Friedman E, Hamann U, Huo D, Kwong A, et al. Mutational spectrum in a worldwide study of 29,700 families with BRCA1 or BRCA2 mutations. Hum Mutat. 2018;39:593–620.
Shimelis H, Mesman RLS, Von Nicolai C, Ehlen A, Guidugli L, Martin C, et al. BRCA2 hypomorphic missense variants Confer moderate risks of breast Cancer. Cancer Res. 2017;77:2789–99.
Kiavue N, Cabel L, Melaabi S, Bataillon G, Callens C, Lerebours F, et al. ERBB3 mutations in cancer: biological aspects, prevalence and therapeutics. Oncogene. 2020;39:487–502.
Bidard FC, Ng CKY, Cottu P, Piscuoglio S, Escalup L, Sakr RA, et al. Response to dual HER2 blockade in a patient with HER3-mutant metastatic breast cancer. Ann Oncol. 2015;26:1704–9.
Jaiswal BS, Kljavin NM, Stawiski EW, Chan E, Parikh C, Durinck S, et al. Oncogenic ERBB3 mutations in human cancers. Cancer Cell. 2013;23:603–17.
Download references
Acknowledgements
We thank Dr. Fabian Siegel from the Department of Medical Bioinformatics for assistance in identification of patients.
T.Z. was supported by the Clinician Scientist program “Interfaces and Interventions in Chronic Complex Conditions” funded by the DFG (EB 187/8 − 1). Q.X. was supported by a fellowship of the Chinese Scholarship Council (CSC).
Open Access funding enabled and organized by Projekt DEAL.
Author information
Authors and affiliations.
Department of Medicine II, Medical Faculty Mannheim, Heidelberg University, Mannheim, Germany
Lena Dreikhausen, Anna Klupsch, Isabella Wiest, Qiyun Xiao, Nadine Schulte, Johannes Betge, Matthias Ebert & Tianzuo Zhan
Junior Clinical Cooperation Unit Translational Gastrointestinal Oncology and Preclinical Models, German Cancer Research Center, Heidelberg, Germany
Johannes Betge
Mannheim Cancer Center, Medical Faculty Mannheim, Heidelberg University, Mannheim, Germany
Johannes Betge, Ralf-Dieter Hofheinz, Matthias Ebert & Tianzuo Zhan
Department of Medicine III, Medical Faculty Mannheim, Heidelberg University, Mannheim, Germany
Tobias Boch & Ralf-Dieter Hofheinz
Institute of Pathology, Medical Faculty Mannheim, Heidelberg University, Mannheim, Germany
Christoph Brochhausen & Timo Gaiser
Medical Faculty Mannheim, DKFZ-Hector Cancer Institute, Heidelberg University, Mannheim, Germany
Johannes Betge, Tobias Boch, Matthias Ebert & Tianzuo Zhan
You can also search for this author in PubMed Google Scholar
Contributions
All authors contributed to the study conception and design. Data collection and analysis were performed by L.D., A.K. and T.Z. The manuscript was written by L.D. and T.Z., and I.W., Q.X., N.S., J.B., T.B., C.B., T.G., R.H. and M.E. commented on previous versions of the manuscript. All authors read and approved the final manuscript.
Corresponding author
Correspondence to Tianzuo Zhan .
Ethics declarations
Ethics approval and consent to participate.
This study was performed in line with the principles of the Declaration of Helsinki. Approval was granted by the Ethics Committee of Heidelberg University (identifier: 2022-823-AF11).
Due to the retrospective design of the study, no written informed consent from patients was required, as decided by the local ethics committee.
Consent for publication
Not applicable.
Competing interests
The authors declare no competing interests.
Additional information
Publisher’s note.
Springer Nature remains neutral with regard to jurisdictional claims in published maps and institutional affiliations.
Electronic supplementary material
Below is the link to the electronic supplementary material.
Supplementary Material 1
Rights and permissions.
Open Access This article is licensed under a Creative Commons Attribution 4.0 International License, which permits use, sharing, adaptation, distribution and reproduction in any medium or format, as long as you give appropriate credit to the original author(s) and the source, provide a link to the Creative Commons licence, and indicate if changes were made. The images or other third party material in this article are included in the article’s Creative Commons licence, unless indicated otherwise in a credit line to the material. If material is not included in the article’s Creative Commons licence and your intended use is not permitted by statutory regulation or exceeds the permitted use, you will need to obtain permission directly from the copyright holder. To view a copy of this licence, visit http://creativecommons.org/licenses/by/4.0/ . The Creative Commons Public Domain Dedication waiver ( http://creativecommons.org/publicdomain/zero/1.0/ ) applies to the data made available in this article, unless otherwise stated in a credit line to the data.
Reprints and permissions
About this article
Cite this article.
Dreikhausen, L., Klupsch, A., Wiest, I. et al. Clinical impact of panel gene sequencing on therapy of advanced cancers of the digestive system: a retrospective, single center study. BMC Cancer 24 , 526 (2024). https://doi.org/10.1186/s12885-024-12261-2
Download citation
Received : 27 November 2023
Accepted : 15 April 2024
Published : 25 April 2024
DOI : https://doi.org/10.1186/s12885-024-12261-2
Share this article
Anyone you share the following link with will be able to read this content:
Sorry, a shareable link is not currently available for this article.
Provided by the Springer Nature SharedIt content-sharing initiative
- Targeted therapy
- Panel gene sequencing
- Cancer therapy
- Precision oncology
ISSN: 1471-2407
- Submission enquiries: [email protected]
- General enquiries: [email protected]

IMAGES
VIDEO
COMMENTS
An international journal of medical genetics, molecular medicine and personalized medicine, Clinical Genetics links research to the clinic, translating advances in our understanding of the molecular basis of genetic disease for the practicing clinical geneticist. We publish research articles, short reports, reviews and mini-reviews that connect medical genetics research with clinical practice.
Participants in clinical studies help current and future generations. Through these studies, researchers develop new diagnostic tests, more effective treatments, and better ways of managing diseases with genetic components. Participants in studies are actively involved in understanding their disorder and current research.
RSS Feed. Clinical genetics involves the study, counselling and treatment of individuals and families with heritable disorders and disease predisposition. Diagnostic tools include standard ...
AIMS AND SCOPE. Clinical Genetics links the clinic to research or provides a link between academia and the clinic, translating advances in our understanding of the molecular basis of genetic disease for the practicing clinical geneticist. The journal publishes high quality research papers, short reports, and reviews that connect medical ...
Genomic research has evolved from seeking to understand the fundamentals of the human genetic code to examining the ways in which this code varies among people, and then applying this knowledge to ...
The genetics of obesity: from discovery to biology. In this Review, Loos and Yeo summarize our current understanding of the genetic underpinnings of monogenic and polygenic obesity. They highlight ...
Aberrant phase separation and nucleolar dysfunction in rare genetic diseases. Frameshift mutations that create arginine-rich basic tails in transcription factors and other proteins can lead to ...
The role of community engagement in promoting research participants' understanding of pharmacogenomic research results: Perspectives of stakeholders involved in HIV/AIDS research and treatment ... Create a weekly email alert for: Clinical genetics You can change these options by clicking ...
1. Introduction. The past two decades have witnessed major advances in technologies and clinical applications of genetics and genomics. Twenty years ago, the human genome project [1-2] provided the first glimpses into the human genome sequence and launched a new era of human genetics: biologists and geneticists for the first time had got almost the entire human genome at their fingertips ...
JHG is a collaborative effort between the Departments of Genetic Medicine and Pathology to provide a variety of DNA genotyping and sequencing services, both research and clinical, to the patients, physicians and scientists of Johns Hopkins Medicine. The Department of Genetic Medicine also houses research centers with significant funding from ...
The Department of Clinical Genomics-Research at Mayo Clinic conducts research on a wide range of diseases and conditions that have a genetic basis, including common forms of cancer such as breast cancer, and rare and novel genetic disorders, such as lysosomal storage diseases. The overarching goal of research within the Department of Clinical ...
For many years, clinical genetics input has at times influenced acute care, for example in diagnosing trisomies in the neonatal period, or informing the care of babies born with ambiguous genitalia. ... The consequences of this shift are still being navigated in the clinical setting - research indicates that patients often see genetic ...
Another vital component of clinical research at NHGRI are studies aimed at examining the psychosocial, ethical and policy implications of genetics research. Research projects currently underway in this area include an empirical study to determine what patients understand about the storage and use of DNA for future research, investigations into ...
Clinical Genetics. Yale Clinical Genetics is committed to providing excellent clinical care for adults and children in a setting that values compassion, collaboration, and respect for individuals and families. Five clinical genetics physicians certified by the American Board of Medical Genetics provide state of the art genetic services ...
A gift to the Stanford Genetics department supports our research and education. Donations are vital to the achievements of our work and are greatly appreciated. Checks payable to Stanford University. Please note on the check WAZC/Genetics and specifics of where the funds should be directed. Thank you. Kindly send by mail to: Development Services
The conduct of human subjects research involves many complex issues that must be addressed in applications submitted to the IRBs. The JHM IRBs have noted over the years that in addition to the issues that must be addressed for all research projects, there are several specific points that arise in the conduct of genetic testing protocols.
Technological improvements in Clinical Genetics have progressed apace, transforming the landscape of both diagnosis and management of inherited genetic diseases. Initially testing for single gene ...
Clinical Genetics Branch (CGB) investigators study individuals at and populations at high genetic risk of cancer in order to improve our understanding of cancer etiology and to advance clinical care. Our multidisciplinary approach combines clinical, genetic, genomic, epidemiologic, behavioral, statistical, and laboratory scientific research ...
Clinical Genomic Database. A key barrier to translating the power of genomic sequencing to clinically-oriented research analyses involves the time and resources required for clinically-relevant analysis. To help address this barrier, we constructed the Clinical Genomic Database (CGD), a manually curated database of conditions with known genetic ...
Congenital heart disease (CHD) and cardiomyopathies are the leading cause of morbidity and mortality worldwide. These conditions are often caused by genetic factors, and recent research has shown that genetic and genomic testing can provide valuable information for patient care. By identifying genetic causes, healthcare providers can screen for other related health conditions, offer early ...
In a landmark study, an international consortium led by researchers at Children's Hospital of Philadelphia (CHOP) published the final results of a key clinical trial of the gene therapy CASGEVY (exagamglogene autotemcel) for the treatment of sickle cell disease in patients 12 years and older with recurrent vaso-occlusive crises (VOCs).
1Department of Clinical Genetics, University Hospitals of Leicester NHS Trust, Leicester Royal Inrmary, Leicester LE1 5WW, UK. 2Department of Genetics and Genome Biology, fi University of ...
Media Advisory. Friday, April 26, 2024. Analysis identifies 50 new genomic regions associated with kidney cancer risk. What. In a new analysis of genetic susceptibility to kidney cancer, an international team of researchers has identified 50 new areas across the genome that are associated with the risk of developing kidney cancer. These insights could one day be used to advance our ...
This research enhances the comprehension of genetic factors contributing to spontaneous abortion. Similar content being viewed by others. ... a joint consensus recommendation of the American College of Medical Genetics and Genomics (ACMG) and the Clinical Genome Resource (ClinGen). Genet Med Off J American College Med Genet. 2020;22(2):245-57.
An international journal of medical genetics, molecular medicine and personalized medicine, Clinical Genetics links research to the clinic, translating advances in our understanding of the molecular basis of genetic disease for the practicing clinical geneticist. We publish research articles, short reports, reviews and mini-reviews that connect medical genetics research with clinical practice.
Panel gene sequencing is an established diagnostic tool for precision oncology of solid tumors, but its utility for the treatment of cancers of the digestive system in clinical routine is less well documented. We retrospectively identified patients with advanced or metastatic gastrointestinal, pancreaticobiliary or hepatic cancers who received panel gene sequencing at a tertiary university ...
Medical genetics involves the application of genetics to medical care, including research on the causes and inheritance of genetic disorders, and their diagnosis and management. ... and clinical ...
Oral presentations include updated results from clinical study of otoferlin gene therapy DB-OTO demonstrating restoration in children with profound genetic hearing loss Additional presentations cover progress in novel genetic medicine delivery systems and immune response modulation TARRYTOWN, N.Y.