Thank you for visiting nature.com. You are using a browser version with limited support for CSS. To obtain the best experience, we recommend you use a more up to date browser (or turn off compatibility mode in Internet Explorer). In the meantime, to ensure continued support, we are displaying the site without styles and JavaScript.
- View all journals
- My Account Login
- Explore content
- About the journal
- Publish with us
- Sign up for alerts
- Open access
- Published: 28 August 2023

Green synthesis of copper oxide nanoparticles and its efficiency in degradation of rifampicin antibiotic
- Dennis Mwanza Nzilu 1 ,
- Edwin Shigwenya Madivoli 1 ,
- David Sujee Makhanu 2 ,
- Sammy Indire Wanakai 1 ,
- Gideon Kirui Kiprono 1 &
- Patrick Gachoki Kareru 1
Scientific Reports volume 13 , Article number: 14030 ( 2023 ) Cite this article
18k Accesses
20 Citations
23 Altmetric
Metrics details
- Environmental sciences
- Nanoscience and technology
In recent ages, green nanotechnology has gained attraction in the synthesis of metallic nanoparticles due to their cost-effectiveness, simple preparation steps, and environmentally-friendly. In the present study, copper oxide nanoparticles (CuO NPs) were prepared using Parthenium hysterophorus whole plant aqueous extract as a reducing, stabilizing, and capping agent. The CuO NPs were characterized via UV–Vis Spectroscopy, Fourier Transform Infrared Spectroscopy (FTIR), powder X-Ray Diffraction (XRD), Scanning Electron Microscopy (SEM), Transmission Electron Microscopy (TEM), and Dynamic Light Scattering (DLS). The UV–Vis spectra of CuO NPs showed a surface plasmonic resonance band to occur at 340 nm. FTIR analysis revealed the presence of secondary metabolites on the surface of CuO NPs, with a characteristic Cu–O stretching band being identified at 522 cm −1 . Scanning electron micrographs and transmission electron micrographs showed that CuO NPs were nearly spherical, with an average particle of 59.99 nm obtained from the SEM micrograph. The monoclinic crystalline structure of CuO NPs was confirmed using XRD, and crystallite size calculated using the Scherrer-Debye equation was found to be 31.58 nm. DLS showed the presence of nanoparticle agglomeration, which revealed uniformity of the CuO NPs. Furthermore, the degradation ability of biosynthesized nanoparticles was investigated against rifampicin antibiotic. The results showed that the optimum degradation efficiency of rifampicin at 98.43% was obtained at 65℃ temperature, 50 mg dosage of CuO NPs, 10 mg/L concentration of rifampicin solution, and rifampicin solution at pH 2 in 8 min. From this study, it can be concluded that CuO NPs synthesized from Parthenium hysterophorus aqueous extract are promising in the remediation of environmental pollution from antibiotics. In this light, the study reports that Parthenium hysterophorus -mediated green synthesis of CuO NPs can effectively address environmental pollution in cost-effective, eco-friendly, and sustainable ways.
Similar content being viewed by others
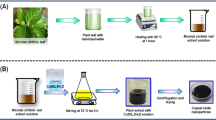
Green synthesis, characterization, antibacterial, and antifungal activity of copper oxide nanoparticles derived from Morinda citrifolia leaf extract
Manogar Priya, Raja Venkatesan, … Seong-Cheol Kim
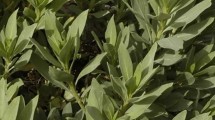
Green synthesis of multifunctional carbon coated copper oxide nanosheets and their photocatalytic and antibacterial activities
Hamida Bibi, Mudassar Iqbal, … Suliman Mohammed Alghanem
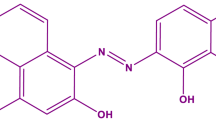
Green synthesis and characterization of α-Mn2O3 nanoparticles for antibacterial activity and efficient visible-light photocatalysis
Saeid Taghavi Fardood, Farzaneh Moradnia, … Ky Nguyen
Introduction
Water shortage remains one of the global challenges affecting many of the world's population as it is documented that about 26% (2 billion people) lack access to safe drinking water per the UNESCO 2023 report 1 . The report further indicates that 2–3 billion people worldwide experience water shortage, with water scarcity projected to increase in the coming years. It is reported that about half of the global population is at risk of experiencing water scarcity 2 . A report published during Africa Health Agenda International Conference (AHAIC2023) revealed that climatic changes have worsened water scarcity challenges in Africa 3 . Climatic changes affect terrestrial water storage, further exacerbating water scarcity and leading to a global water crisis. Amidst the water shortage crisis, water pollution from active pharmaceutical compounds (APIs), such as antibiotics classified as emerging pollutants, continues to rise 3 , 4 , 5 . Pathways for antibiotics into the environment (soil or surface water) are infiltration from wastewater treatment plants and domestic discharge of human excretion 6 , 7 , 8 . These antibiotics exhibit detrimental effects on humans and water ecosystems, attributed to their higher concentrations above the predicted environmental concentration 8 . The existence of antibiotics in the environment propagates antimicrobial resistance 9 , 10 , 11 , and their low concentrations are difficult to remove using conventional wastewater treatment plants 12 . The World Health Organization declared this resistance a public health crisis, threatening modalities to treat the increasing disease burden 13 . Another potential harm exhibited by antibiotics in the environment results from their interference with physiological processes when absorbed by plants hence a propagation of ecotoxicity effect 7 .
Rifampicin is one of the antibiotics prescribed to treat tuberculosis (TB), with a global disease burden of about 10.6 million people 13 , 14 , 14 . In developing countries, where minimal wastewater treatment plants exist, these antibiotics, which are not fully assimilated into the body, have been observed in surface water 16 . Conventional methods to remove various wastewater antibiotics include physical, chemical, and biological methods 17 . However, these methods have limitations due to their financially intensive nature, high power consumption, and ineffectiveness in completely removing these pollutants 17 , 18 . To this end, researchers are considering embracing nanoparticles as catalysts for removing some of these pollutants due to their ability to find multiple applications 19 , 20 , 21 . These nanomaterials exhibit improved physicochemical properties than their large materials. For instance, metal nanoparticles used in the adsorption and degradation of pollutants have enhanced surface area to volume ratio and chemical stability compared to their bulk counterparts 22 . The increased surface area strengthens the removal capacity of the antibiotic into active sites of the nanoparticles 20 , 21 , 23 . Several methods exist to synthesize metallic nanoparticles, including physical, chemical, and biological methods 19 , 24 . Some chemical and physical methods used to synthesize nanoparticles include sonochemistry, co-precipitation, solvothermal, pyrolysis, thermal hydrolysis, ball milling, and sol–gel 24 , 25 , 26 . However, chemical and physical methods for synthesizing nanoparticles have drawbacks due to their expensive nature, are time-consuming, energy inefficient, and produce toxic products making them non-environmental friendly 24 , 26 , 27 , 28 . Due to limitations associated with chemical and physical methods, researchers have recently produced eco-friendly nanoparticles by using organisms such as algae, bacteria, fungi, yeast, and plants to produce nanoparticles 20 , 21 , 26 . The use of microorganisms such as fungi and bacteria has limitations in reducing metal ions due to their long synthesis time compared to plant-mediated synthesis 29 .
In particular, the green synthesis method utilizing plant extracts as reducing, stabilizing, and capping agents in the formation of nanoparticles is an alternative to eliminating the challenges associated with the abovementioned methods and promoting environmentally friendly chemistry 19 , 30 , 31 . The green synthesis method is cost-effective, rapid, environmentally friendly, and sustainable since plant materials are available for large-scale nanoparticle production 26 , 28 , 28 , 32 , 33 . CuO NPs have been previously prepared using plant extracts. CuO NPs were synthesized using Catha edulis leaf extracts as reducing and capping agents 34 . In another study, CuO NPs were synthesized using Eucalyptus Globulus leaf extracts for adsorption studies 35 . Celastrus paniculatus was also used to prepare CuO NPs using the secondary metabolites in its leaf extracts 36 . Another study obtained CuO NPs using leaf extracts of the Justicia schimperiana plant 37 . Using plant extracts to synthesize CuO NPs has been proven to be cost-effective, fast, simple, and environmentally friendly 38 .
Table 1 below summarizes some of the nanoparticles or methodologies employed in the degradation of antibiotics.
A common concern for nanoparticles used for wastewater treatment is their toxicity, ecotoxicity, and cytotoxicity nature 45 . The release of CuO NPs into the environment after the degradation process induces toxicity to organisms by oxidative stress responses and is affected by various factors such as shape, size, and particle concentration 45 . Therefore, in large-scale wastewater treatment, it would be challenging to identify the ultimate effect of CuO NPs after their release into the environment following a degradation process. Another consideration relates to understanding the fate of degradation products after their release into the environment. Hence, studying the ecotoxicity of CuO NPs is an ongoing area in research since there is little information available on human exposure data of nanoparticles contained in treated water 46 .
Parthenium hysterophorus is an invasive weed, which is much-branched and short-lived and observed to grow in many parts of the world. It belongs to the Asteraceae family. It can grow up to 1.5 m tall. It can grow in cultivated and agricultural lands and along the roadsides. It is reported to be a host of crop plant diseases and causing dermatitis in animals and humans 47 . The plant has been used as a medicinal agent and bioherbicide 48 , 49 , 50 . Secondary metabolites contained in Parthenium hysterophorus include saponins, major and trace oils, flavonoids, alkaloids, terpenoids, phenols, and tannins which can reduce \({\mathrm{Cu}}^{2+}\) to zero-valent species \({\mathrm{Cu}}^{0}\) 51 . The availability of the plant makes its sustainability and scalability potential in the production of CuO NPs for large-scale wastewater treatment and removal of antibiotics feasible.
In this study, we sought to synthesize CuO NPs using Parthenium hysterophorus whole plant aqueous extract, and the prepared nanoparticles were characterized using UV–Vis spectroscopy, FTIR spectroscopy, XRD, TEM, SEM, and DLS techniques. The CuO NPs were then assessed on their ability to degrade rifampicin antibiotics. The degradation of rifampicin antibiotic using CuO NPs was also investigated by varying temperature, pH, the dosage of nanoparticles, concentration of the antibiotic solutions, and reaction time. To the best of our knowledge, this is the first study reporting degradation of rifampicin antibiotic using CuO NPs prepared from Parthenium hysterophorus whole plant aqueous extracts.
Materials and methods
Collection and preparation of samples.
Fresh Parthenium hysterophorus plant was collected from Kalimoni, Juja, Kiambu County in Kenya. It was identified and authenticated by Mr. John Kamau Muchuku, a Botanist from the Department of Botany, Jomo Kenyatta University of Agriculture and Technology (JKUAT), and a voucher specimen deposited in the JKUAT Botany Herbarium with the accession number DMN-JKUATBH/001/2023A-C. The sample was thoroughly washed with running tap water, rinsed with distilled water, and shed-dried at room temperature for 2 weeks. The dry sample was then ground using a milling machine to a fine powder.
Extraction of secondary metabolites
The procedure for aqueous extraction of Parthenium hysterophorus was adopted from Ref. 52 , in which 20 g of the plant sample was dissolved in 200 mL of distilled water. The mixture was heated in a hot plate at 40 °C for 45 min with constant stirring. The extract was obtained by filtration using Whatman No. 1 filter paper. The extract was then used to synthesize copper oxide nanoparticles 53 .
Green synthesis of copper oxide nanoparticles using parthenium aqueous extract
Synthesis of copper oxide nanoparticles utilized copper (II) sulphate as a metal precursor. 0.01 M CuSO 4 solution was prepared by dissolving 1.59 g in 100 mL of distilled water. The metal solution was then mixed with parthenium aqueous extract in a ratio of 1:4, with the formation of nanoparticles being monitored by a color change and UV–Vis spectrophotometric measurement. The colloidal solution was centrifuged at 4000 rpm for 15 min, washed several times, and the nanoparticles dried in an oven at 80 °C 54 .
Figure 1 shows a schematic diagram of the synthesis of CuO NPs using Parthenium hysterophorus whole plant aqueous extract.
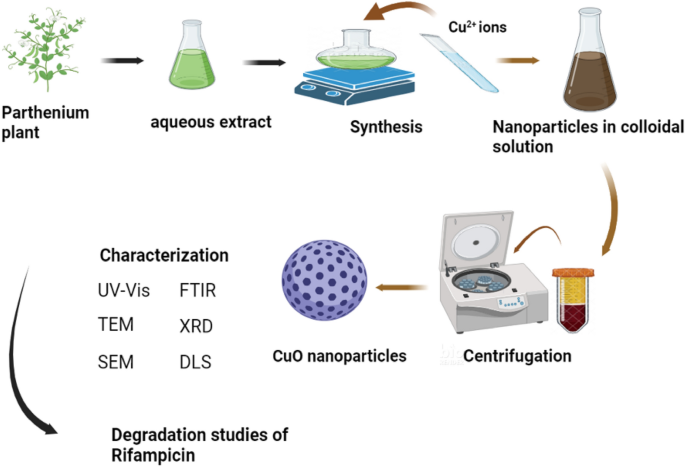
Schematic diagram showing the synthesis of CuO NPs from Parthenium hysterophorus aqueous extract, characterization, and use in degradation studies. Created in BioRender.com.
Characterization of copper oxide nanoparticles
The synthesized nanoparticles were characterized using a Shimadzu UV-1800 UV–Vis spectrophotometer (Shimadzu, Japan) to confirm their surface plasmonic resonance at 200–800 nm wavelengths. The IR spectra of the CuO nanoparticles were acquired in the frequency range of 4000–400 cm −1 using a Bruker Tensor II FT-IR spectrophotometer (Bruker, Ettlingen, Germany) 55 , 56 . The X-ray diffractograms were obtained using a Bruker D8 Advance Diffractometer (Bruker, Ettlingen, Germany) with a copper tube operating under a voltage and current of 40 kV and 40 mA. The samples were irradiated with a monochromatic CuKα radiation of 0.1542 nm, and the diffractograms acquired between 2θ values of 5°–90° at 0.05° intervals with a measurement time of 1 s per 2θ intervals. The nanoparticles' crystalline size was calculated using Debye Scherrer's equation
where D is the average particle size (nm), K is a constant equal to 0.94, λ is the wavelength of X-ray radiation, β is full-width at half maximum of the peak in radians, and theta is the diffraction angle (degree). Morphological analysis of the nanocomposite was observed using the Tescan Mira3 LM FE scanning electron microscope (Tescan, Brno—Kohoutovice, Czech Republic) operating under an accelerating voltage of 3 kV. The samples were sputter coated with 4 nm gold before analysis to avoid charging using AGB7340 Agar Sputter Coater (Agar Scientific, Essex, United Kingdom) 55 , 57 . TEM analysis was performed on a Tecnai G2 Spirit (ThermoFischer Scientific, Oregon USA) under an operating voltage of 120 kV equipped with veleta 2048 × 2048 wide angle and Eagle 4096 × 4096 bottom mount detectors. The dry samples were suspended in ultrapure water (Barnstead Genpure, Thermoscientific, Germany), ultra-sonicated to obtain a solution that was drop casted on 300 mesh carbon films before analysis 55 , 57 . To determine the particle size distribution and polydispersity index of the suspended nanoparticles, the metallic nanoparticles were resuspended in ultrapure water (18 MΩ cm Barnstead Genpure UV-TOC, Thermoscientific, Germany) and ultrasonicated to obtain a solution of suspended nanoparticles. The solutions were then filtered through 0.25 µM PTFE syringes into glass vials, and 45 µL of each solution was then transferred onto quartz cuvettes before analysis. The particle size distribution and polydispersity index were then measured using a Bechman Coulter DelsaMax pro dynamic light scattering analyzer (Beckman Coulter, Indianapolis, United States) 58 , 59 .
Degradation studies
To determine the efficiency of CuO nanoparticles in the degradation of rifampicin antibiotic, degradation studies were conducted while varying pH, degradation time, the concentration of the antibiotic, temperature, and nanoparticles dose. Rifampicin solution was prepared according to Ref. 11 in which 0.0025 g of rifampicin was dissolved in 1 mL methanol and 10 mL distilled water and the contents were transferred into a 250 mL volumetric flask 11 , 60 . The resultant rifampicin solution (10 mg/L) was used, and the degradation efficiency was calculated using Eq. ( 1 ) 36
where A o, the initial absorbance of rifampicin, and A t, the absorbance of rifampicin after time, t.
Ethics approval
The plant materials were collected and used according to the national regulations. The utilization of this plant species for experimental purposes does not require any special permit. All methods used for this study comply with relevant institutional, national, and international guidelines and legislation. The authors recognize that the collection of plant species might be subject to guidelines established under the IUCN Policy Statement on Research Involving Species at Risk of Extinction Protocols. However, our search from the IUCN database found that Parthenium hysterophorus is not Red Listed or classified as a threatened species.
Results and discussion
Uv–vis spectral analysis.
The formation of CuO NPs was followed through measurement of its SPR peak using a UV–Vis spectrophotometer, and the results are depicted in Fig. 2 .
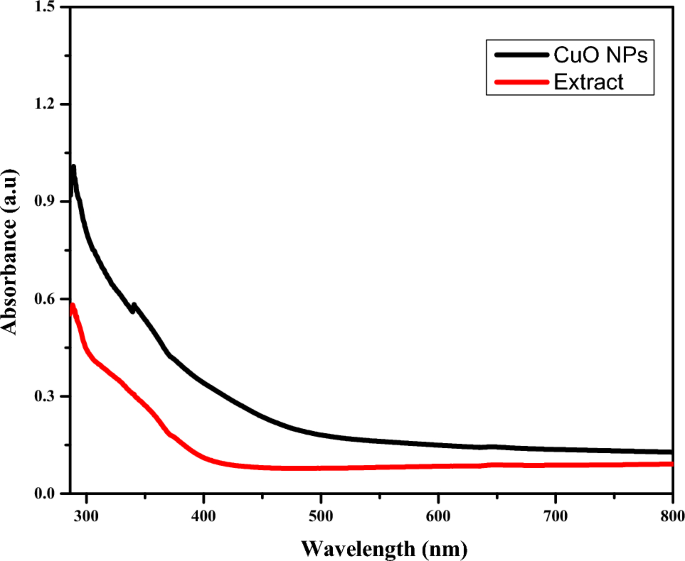
UV–Vis spectra of extract and CuO NPs.
As shown in Fig. 2 , the maximum absorption peak occurred at 340 nm, attributed to the SPR absorption band of CuO NPs. The SPR absorption band of CuO NPs at 340 nm confirmed the formation of CuO NPs 61 . As the wavelength increased, the absorbance intensity decreased, indicating that formation did not occur at a large wavelength. CuO NPs formed in agreement with studies that proposed that CuO NPs formed between 200 and 350 nm 62 .
Fourier transform infrared spectroscopy analysis
The functional groups present in Parthenium hysterophorus aqueous extract and CuO NPs were identified using FTIR spectrometry in the range of 4000–400 cm −1 (Fig. 3 ).
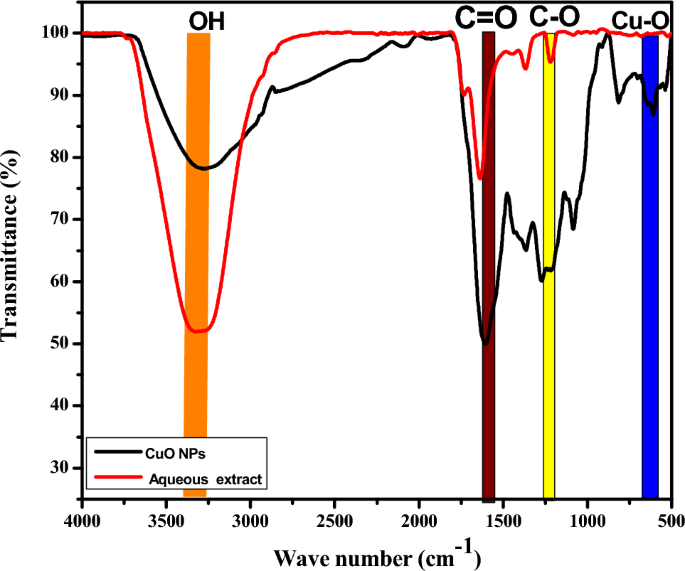
IR spectra of Parthenium hysterophorus aqueous extract and CuO NPs.
The parthenium aqueous extract revealed four peaks. A broad peak in the 3371–3212 cm −1 ranges and sharp peaks at 1630, 1368, and 1209 cm −1 . The FTIR spectrum of CuO NPs was observed at 3284, 1600, 1368, 1278, 1084, 820, 522, and 590 cm −1 . The peak in the 3371–3212 cm −1 range indicates the presence of the O–H group typical of phenols in the extract was present in both the extract and CuO NPs spectra. These peaks correspond to previous study findings 63 , 64 . A peak at 1630 cm −1 and 1600 cm −1 for extract and nanoparticles are associated with a C=O bond stretching vibration. The C=O bond stretching vibration is typical of amides 65 . The peaks at 1368 cm −1 for the extract and CuO NPs spectra represent the amines' C–N stretching vibration 34 . A peak at 1084 cm −1 is associated with C–O stretching vibrations typical to flavonoids 34 , 63 . The characteristic peak at 522 cm −1 and 590 cm −1 indicated the formation of Cu–O stretching vibrations, confirming the formation of CuO NPs 34 , 35 . Therefore, from the FTIR analysis, it can be indicated that the phytochemicals present in the aqueous extract, such as phenols and flavonoids, were responsible for reducing and stabilizing metal ions to form CuO NPs.
Scanning electron microscope analysis
The morphology and surface structure of the synthesized CuO NPs were analyzed using SEM, and obtained micrograph is shown in Fig. 4 .
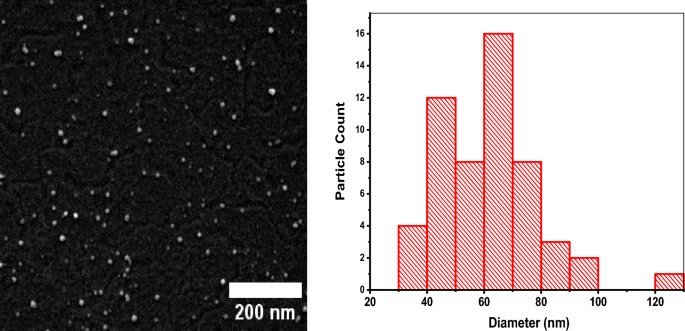
SEM micrograph and size distribution of CuO NPs.
The shape of the nanoparticles was nearly spherical, with less evidence of agglomeration. Previous studies confirmed CuO NPs' spherical shape 66 , 67 . The average particle size determination using ImageJ software showed that the average particle size was 59.99 nm. The particle size distribution for CuO NPs obtained in this study agreed with previous studies 67 , 68 .
Transmission electron microscope
The size of the synthesized copper oxide nanoparticles was also visualized using TEM micrographs, as depicted in Fig. 5 .
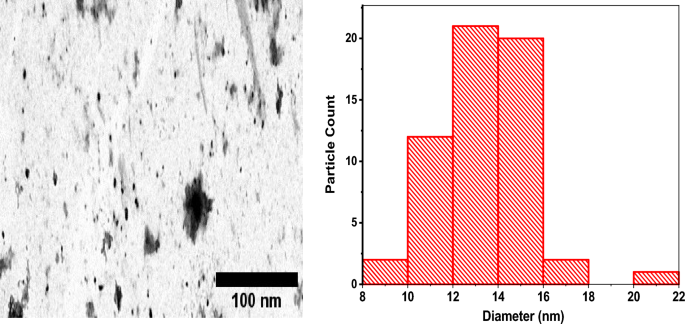
TEM micrograph and size distribution of CuO NPs.
The TEM micrographs revealed that the nanoparticles were nearly spherical 69 . The particle size distribution from the TEM micrograph was 8–22 nm which falls within the size range of CuO NPs obtained in previous studies 69 , 70 .
X-ray diffraction analysis
The XRD diffraction pattern of CuO NPs is presented in Fig. 6 .
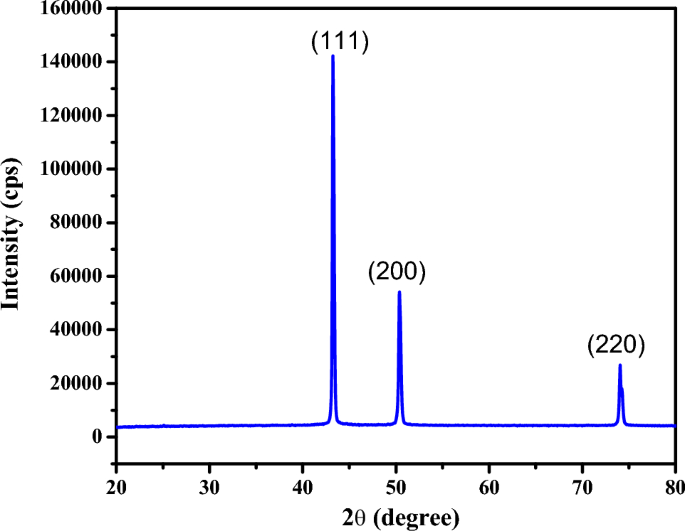
XRD pattern of CuO NPs.
X-ray diffraction analysis shows the presence of three prominent peaks at 2θ values in the range of 20° to 80° at 43.6°, 50.8°, and 73.5°. These 2θ values present (111), (200), and (220) crystallographic planes from the JCPDS database, respectively 38 , 41 . The XRD pattern confirmed the high crystallinity nature of CuO NPs 31 . The peaks can be indexed to be typical monoclinic structures of CuO NPs 34 . The average crystallite size was calculated using the Scherrer equation 34 , 43 and was found to be 31.58 nm. Other researchers have confirmed the green synthesis of CuO NPs in this size range. 22.4 nm of CuO NPs were synthesized using Bacopa monnieri leaf extract 71 . The average size of CuO NPs was calculated as 29.7 nm in a green synthesis using Curcuma longa root extracts 31 . The d-spacing and lattice constant parameters were determined using Bragg’s law to be 0.2 nm and 0.34 nm, respectively. These results correspond to previous study 72 .
Dynamic light scattering analysis
Dynamic light scattering was used to determine the size distribution of the synthesized copper oxide nanoparticles, and the results are depicted in Fig. 7 .
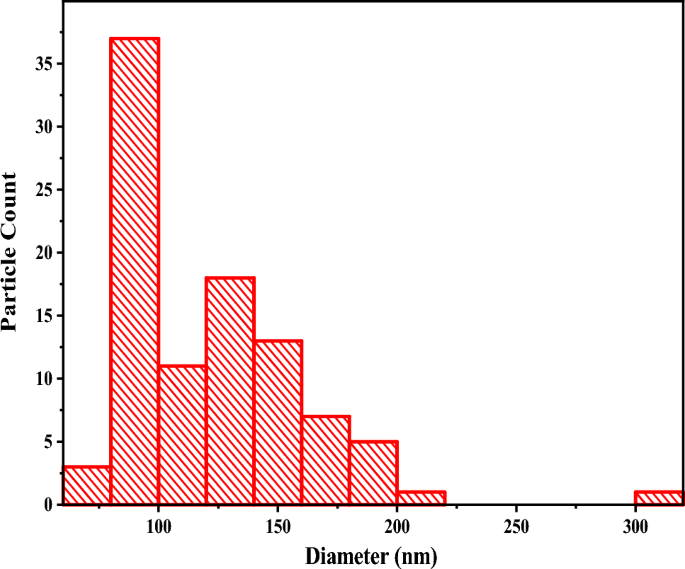
Size distribution of CuO NPs from DLS.
The DLS histogram shows that the particles were larger and polydisperse compared to those observed in SEM micrographs which range from 20 to 120 nm (Fig. 4 ). The diameter of the particles was in the range of 0 to 200 nm, with a large population of nanoparticles having an average size below 100 nm. The polydispersity index (PDI) was 0.3 or less, which indicated that the individual size distribution was monodispersed 73 . As a result, individual monodispersity can be attributed to agglomeration or aggregation during the nanoparticle synthesis process 74 .
Degradation studies of rifampicin using CuO NPs
Effect of cuo nps dose on degradation efficiency.
Figure 8 depicts the effect of CuO NPs dose on the degradation of the rifampicin antibiotic.
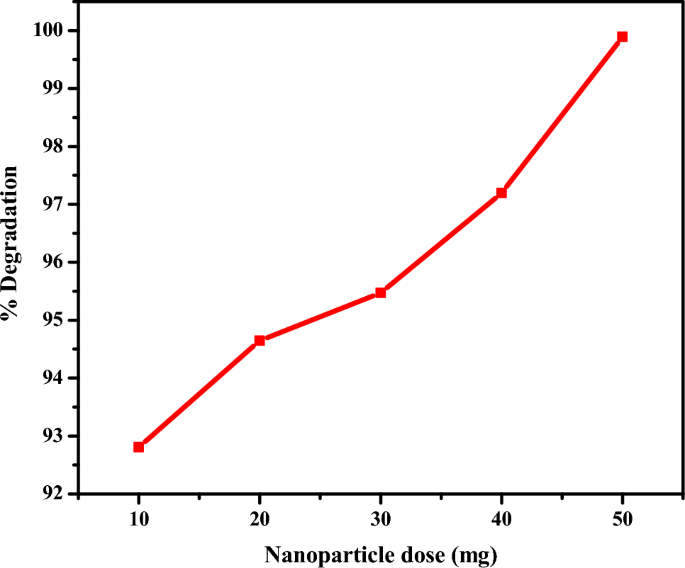
Effect of CuO NPs dose on degradation efficiency.
As can be seen in Fig. 8 as the dose of CuO NPs was increased from 10 to 50 mg, the percentage of degradation of rifampicin increased from 92.81 to 99.89%, respectively, within 210 min. The increase in the degradation percentage upon increasing the dose of CuO NPs can be attributed to the increased total surface area of the nanoparticles, which increases the active sites that interact with the rifampicin antibiotic 75 , 76 . Therefore, as the number of active sites on the surface of CuO NPs increases, more reactive radicals are generated, which are involved in the degradation of rifampicin, and the degradation efficiency improves 76 , 77 . It was observed that the intensity of absorption of rifampicin decreased as the amount of CuO NPs was increased. This supports the fact that more molecules of rifampicin were bound to the active sites of the CuO NPs, and their structure was destroyed quickly and faster as more active sites were available at higher doses of CuO NPs. The decrease in absorbance intensity at higher nanoparticle doses indicates the formation of intermediate degradation byproducts.
Effect of rifampicin concentration on degradation
Figure 9 depicts the effect of the concentration of rifampicin antibiotic on the degradation efficiency using CuO NPs.
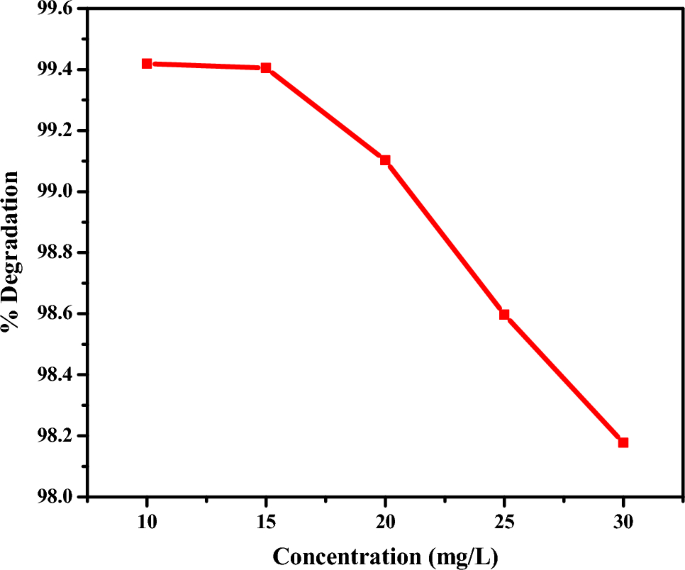
Effect of rifampicin concentration on degradation efficiency.
As the concentration of rifampicin increased from 10 to 30 mg/L, the percent of degradation of rifampicin decreased from 99.42 to 97.78%, respectively, within 12 min of the reaction. At higher rifampicin concentrations, all active sites of CuO NPs were occupied and saturated to react and degrade the rifampicin, resulting in less degradation percent at high concentrations of rifampicin 76 , 77 . All the active sites of CuO NPs were used at higher concentrations of rifampicin, and more molecules of rifampicin remained in the solution. Simultaneously, the generation of reactive species such as OH radical from the hydrogen peroxide in the solution becomes suppressed when the concentration of rifampicin solution is high 76 . Another possible reason for a decreasing degradation efficiency as the concentration of rifampicin increases results from the production of degradation byproducts which competes with the rifampicin molecules for the limited number of active sites on the surface of CuO NPs and the reactive radicals present. Regarding absorbance from the UV–Vis spectrum, it was observed that at higher concentrations of rifampicin, the absorbance intensity of rifampicin was higher, indicating minimal degradative interaction between rifampicin and CuO NPs 78 , 79 .
Effect of pH on degradation
Figure 10 depicts the effect of varying pH on the efficiency of degradation of rifampicin using CuO NPs.
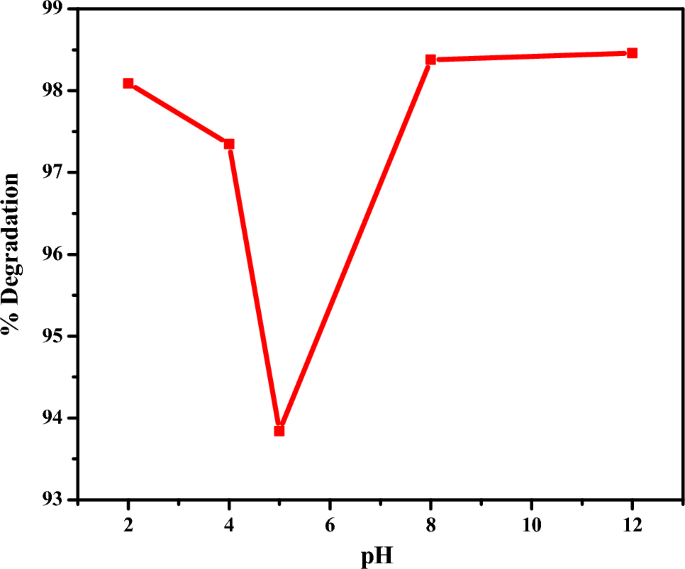
Effect of pH of rifampicin solution on degradation.
The pH of the rifampicin solution was varied using 0.1 M HCl and 0.1 M NaOH solutions. By varying the pH of the solution, the concentration of H + and OH − ions become affected, which are responsible for generating reactive species involved in the degradation process of rifampicin antibiotic. In acidic conditions at pH 2 and 4, the percentage degradation of rifampicin by CuO NPs was calculated and found to be 98.09% and 97.35%, respectively. In basic conditions, the degradation studies were carried out at pH 8 and 12, which yielded a degradation efficiency of 98.38% and 98.46%, respectively. Compared to the solution of rifampicin which was at pH 5 and had a degradation efficiency of 93.84%, it was observed that the rate of degradation of rifampicin increased significantly at both acidic and basic media. The increase in the efficiency of degradation in acidic media can be attributed to the generation of hydrogen radicals upon the interaction of hydrogen peroxide with CuO NPs 80 . Simultaneously, in acidic media, the nanoparticles become positively charged, which increases the rate of interaction of H 2 O 2 with CuO NPs resulting in the generation of more H + ions, and increasing the degradation of rifampicin 11 , 75 . The increase in efficiency of degradation of rifampicin in basic media can be attributed to the increase in OH − ions leading to the generation of more hydroxyl radicals which activates the active sites of CuO NPs. The increase in hydroxyl radicals advances the interaction rate of nanoparticles with rifampicin solution leading to improved degradation efficiency compared to rifampicin solution at pH 5. The degradation of rifampicin can be described to be pH-dependent, and its alteration in the surface charge of the CuO NPs results in varying percentage degradation 81 .
Effect of time on degradation
Figure 11 depicts the effect on the interaction time of the rifampicin antibiotic with the CuO NPs.
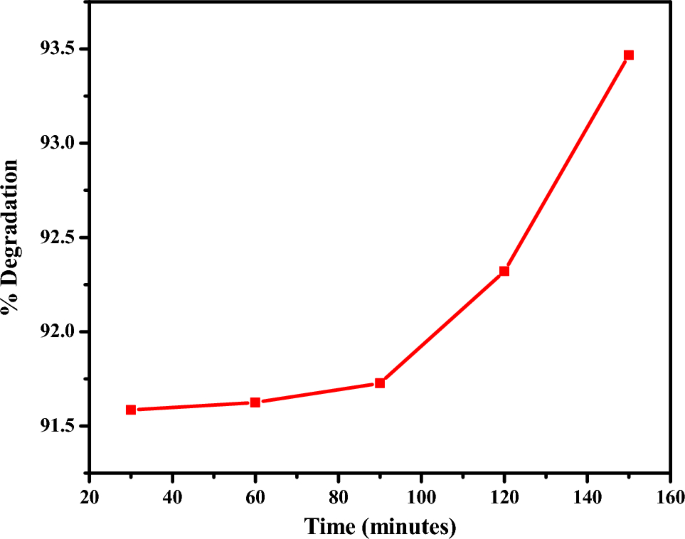
Effect of time on the rate of degradation of rifampicin.
The degradation of rifampicin was observed by measuring the intensity of absorption at regular time intervals. The decrease in absorption intensity at λ max = 480 nm was correlated to the reduction in rifampicin concentration, which indicated the increasing degradation rate of rifampicin by CuO NPs with interaction time 82 . These results were consistent with previous studies which reported improved removal efficiency of levofloxacin antibiotic using CuO NPs from 27% in 15 min to 71% within 120 min 83 .
Effect of temperature on degradation
Figure 12 depicts the observable changes in the effect of temperature on the percent of degradation of rifampicin using CuO NPs.
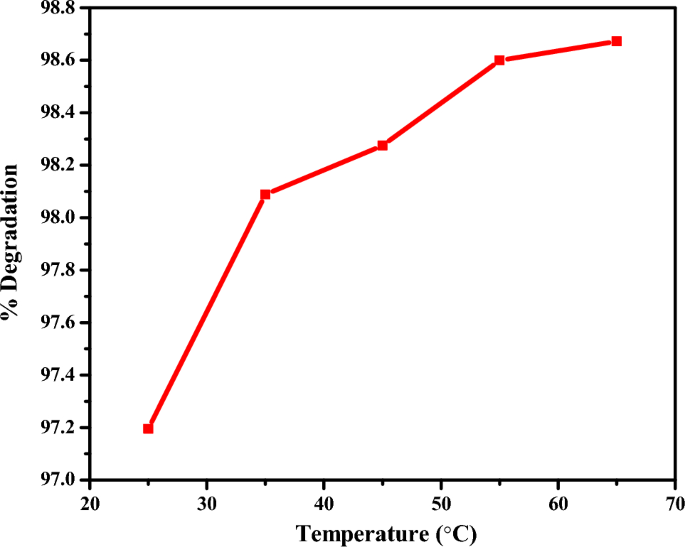
Effect of temperature on degradation efficiency.
As the temperature increased from 25 to 65 °C, the percent of degradation of rifampicin increased from 97.19 to 98.76%, respectively. As reported in previous studies, increases in temperature improve the kinetics of degradation because of an increase in Brownian motion 75 , 84 . It was also observed through the change in absorbance of rifampicin, which was reduced upon increasing the temperature after 24 min 11 .
The change in heat and entropy of the degradation reaction of rifampicin using CuO NPs was determined from the slope and intercept values, respectively, of Van't Hoff's plot of \(ln {k}_{eq}\) against \(\frac{1}{T}\) , and the results are depicted in Fig. 13 . The activation energy of the degradation process was also determined using Arrhenius plot of lnk against 1/T and the results are represented in Fig. 14 .
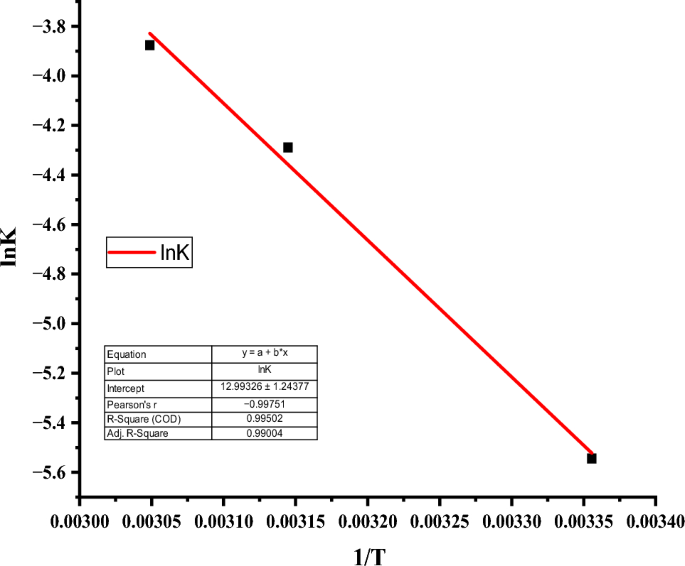
Van’t Hoff plot for degradation of rifampicin.
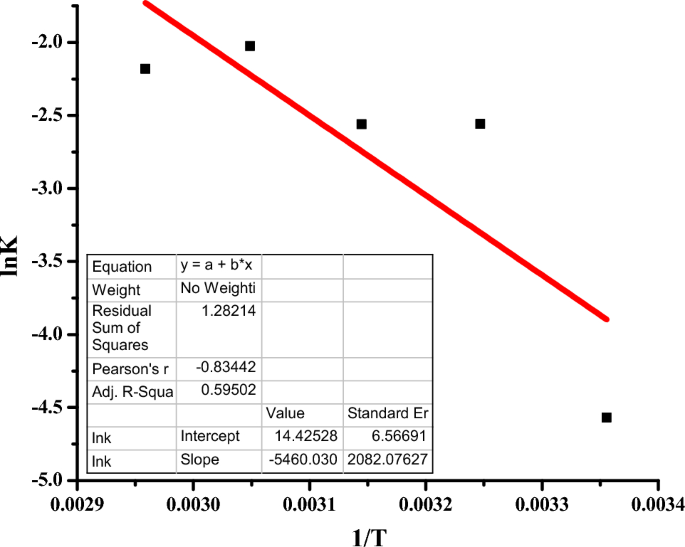
Arrhenius plot of lnk against 1/T to obtain activation energy of the degradation reaction of rifampicin.
Van't Hoff's equation relate the change in equilibrium constant with a decrease in the concentration of rifampicin with increasing temperature. It was observed that at higher temperatures, the rifampicin degradation rate was higher (Fig. 12 ), indicating that the reaction was endothermic 81 . Temperature plays a significant role in enhancing the degradation rate of the antibiotic. The entropy of an endothermic reaction decreases when the reaction temperature increases due to the formation of degradation byproducts. For this reaction, the change in the heat was calculated from the experimental data to be 45.87 kJ mol −1 , while the change in entropy of the reaction was 108.03 J K −1 11 . The value of activation energy (E a ) of rifampicin degradation was calculated from the slope of the Arrhenius plot of lnK against 1/T by following pseudo-second-order kinetics established to occur during the degradation of rifampicin antibiotic by CuO NPs. The value of E a was determined to be 454 kJ mol −1 .
Kinetics of degradation of rifampicin
To understand the degradation kinetics of rifampicin, the kinetic models were fitted using pseudo-first order and pseudo-second order at 298, 308, 328, 328, and 338 K temperatures, as depicted in Table 2 , Figs. 15 , and 16 .
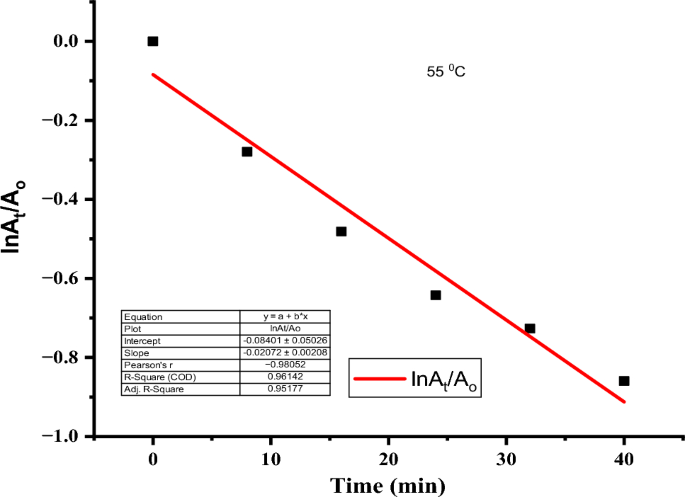
Pseudo-first order plot at 55 °C.
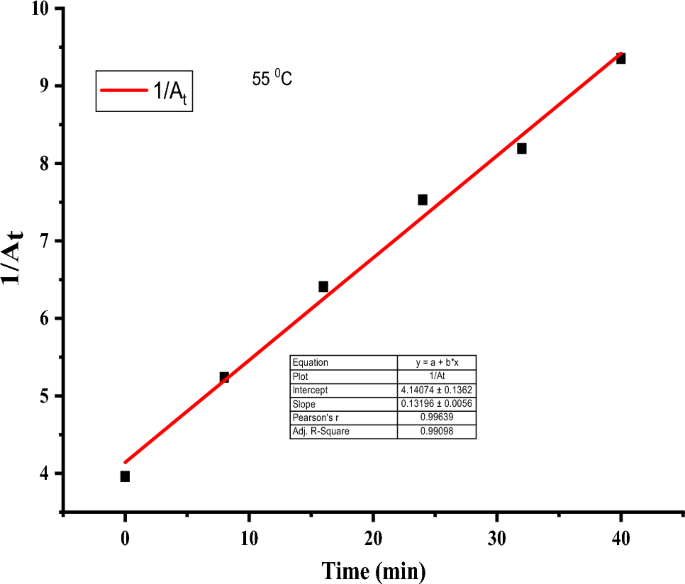
Pseudo-second order plot at 55 °C.
Under varying temperature conditions, the kinetic data fitted pseudo-second order because the R 2 (correlation coefficient) values were ≥ 0.98 for all temperature conditions, which were higher than pseudo-first-order R 2 values. The increase in the rate constants indicated that the reaction was endothermic, consistent with previous findings on the degradation of rifampicin antibiotic 11 .
Degradation efficiency of rifampicin at optimal condition
Figures 17 and 18 depict the degradation efficiency at optimum conditions (10 mg/L rifampicin concentration, 65 °C, and 50 mg CuO NPs, pH 2 and 8).
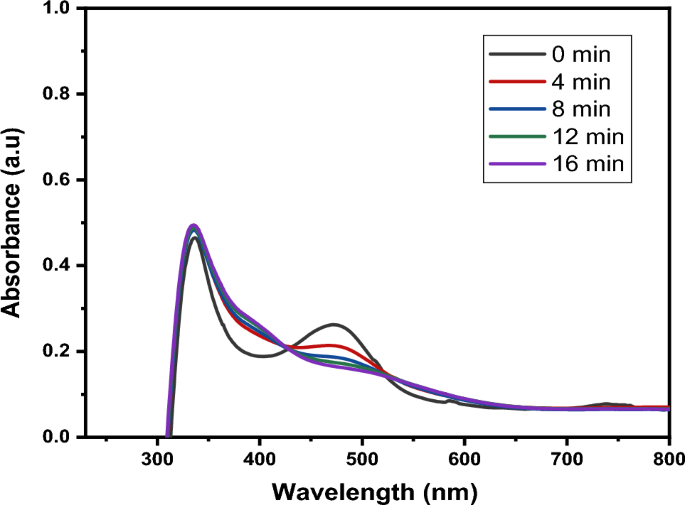
Degradation of rifampicin at optimal conditions at pH 8.
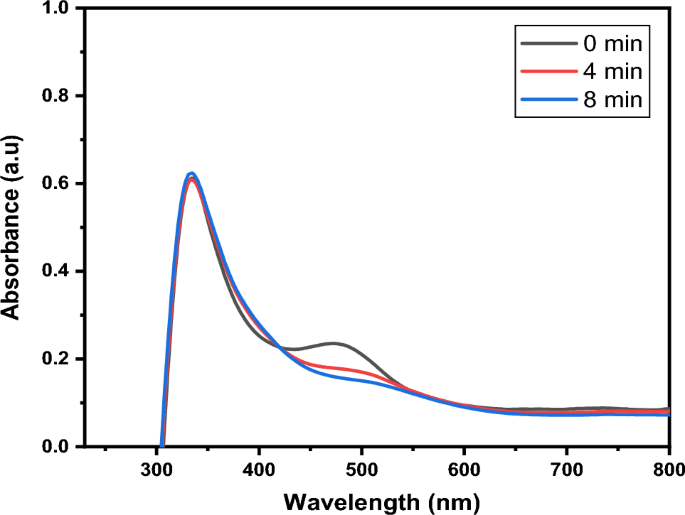
Degradation of rifampicin at optimal conditions at pH 2.
The percentage degradation of rifampicin at pH 8 using the mentioned optimal conditions was calculated and found to be 98.37%, achieved at 16 min. Using the same optimal parameters at acidic pH 2, the degradation efficiency was calculated to be 98.43%, achieved after 8 min. It can be concluded that at the highest dose of CuO NPs (50 mg), more active sites are available to react with the rifampicin molecules, while acidic (pH 2) and basic (pH 8) and increased temperature conditions increase the generation of hydrogen and hydroxyl radicals which further activates the surface of the nanoparticles leading to enhanced degradation efficiency. These optimal conditions increase the generation of radicals, improving the rate at which the rifampicin antibiotic is degraded 11 .
Mechanism of degradation of rifampicin using CuO NPs
Several studies have reported the mechanism of degradation of antibiotics using metallic nanoparticles 41 , 77 , 78 , 79 . The presence of incident light on the surface of CuO NPs results in a hole in the valence band (VB). This creates a positive charge on the VB \(\left({\mathrm{h}}_{\mathrm{VB}}^{+}\right)\) . As a result, the electron is excited from VB to conduction band CB \(({\mathrm{e}}_{\mathrm{CB}}^{-})\) 85 . The presence of excited electrons on the CB reduces oxygen molecules to oxygen radicals, while the water molecules in the reaction are oxidized to hydroxyl radicals 86 , 87 . Figure 19 illustrates the mechanism of degradation of rifampicin using CuO NPs. The mechanisms of degradation of MB dye by ZnO NPs can also be described using the steps shown in the equations below.
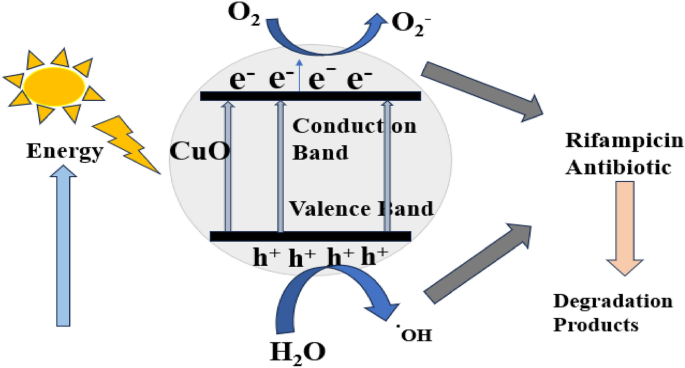
Probable degradation mechanism of rifampicin antibiotic using CuO NPs.
FTIR analysis of CuO NPs after degradation studies
Figure 20 depicts FTIR spectra showing the changes in CuO NPs after degradative interaction with rifampicin.
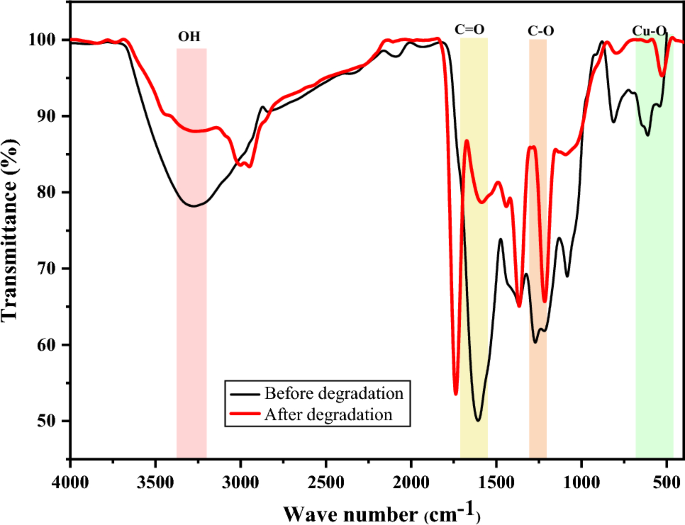
FTIR spectra of CuO NPs before and after degradation of rifampicin.
As shown in Fig. 20 , slight changes were observed in the FTIR spectra of CuO NPs after its use in the degradation of rifampicin antibiotic. Shifts in the wavenumber of the functional groups were observed after the CuO NPs interacted with rifampicin. The broadband typical of an OH group shifted from 3270 cm −1 to two peaks at 3288 cm −1 and 2949 cm −1 after degradation were slightly narrow than before degradation studies. The band typical of C=O groups shifted from 1609 cm −1 before degradation to 1737 cm −1 after degradation. The shift of the C–O was observed from 1271 cm −1 before degradation to 1209 cm −1 after degradation studies. The transitions were attributed to the adsorption of rifampicin on the surface of CuO NPs, which altered the frequencies. The peaks assigned to Cu–O functional group exhibited a slight shift from two peaks at 590 cm −1 and 522 cm −1 to a single peak after degradation at 528 cm −1 . These changes in the peaks of CuO NPs before and after degradation studies of rifampicin demonstrate that the functionality of the CuO NPs was not lost by their degradative ability in removing antibiotics 84 .
Recyclability potential of CuO NPs in the degradation process
The recycle and reuse ability of CuO NPs in the degradation of rifampicin antibiotic was investigated in four cycles for 90 min each. After each use, the nanoparticles were decanted, washed with water, and dried in an oven at 60 °C for 6 h, as previously described 41 , 71 . The dry nanoparticles were then used in the subsequent degradation cycle with fresh 10 mg/L rifampicin solution. The degradation efficiency was calculated, and the results were plotted as shown in Fig. 21 .
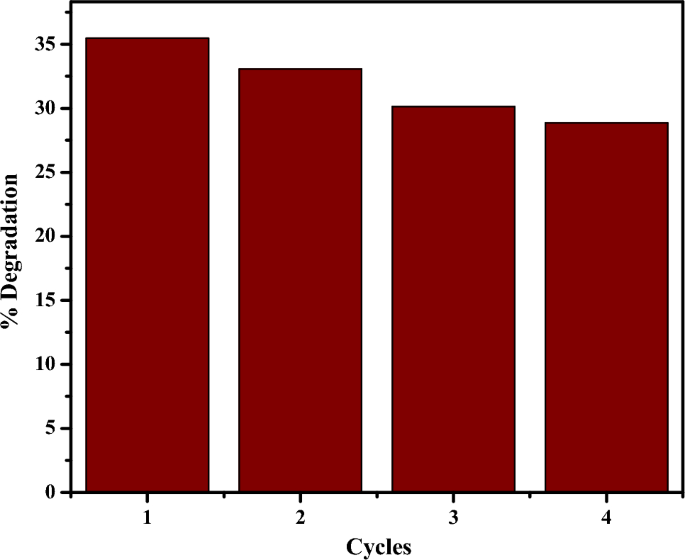
Recycle and reuse of CuO NPs in degradation of rifampicin in four cycles.
The degradation efficiency of CuO NPs on rifampicin antibiotic was observed to have declined from 35.48% (first cycle) to 28.87% (fourth cycle). The decrease in the degradation efficiency in each subsequent recycling cycle can be attributed to the adsorption of rifampicin antibiotics on the active sites of the CuO NPs, which deactivates the catalytic property of the nanoparticles 41 , 71 . In addition, the decrease in degradation efficiency of CuO NPs as the number of cycles increases can be supported by the fact that CuO NPs lose their catalytic performance during separation and drying steps 72 , 88 . This study's recyclability findings are consistent with previously reported studies 72 , 88 .
Comparison with conventional antibiotic degradation methods
The effectiveness of CuO NPs in the degradation of rifampicin antibiotic was compared with other catalysts used in the degradation of antibiotics and summarized in Table 1 . In this study, CuO NPs proved to have improved degradation efficiency in removing rifampicin, making the nanoparticles promising for application in the degradation of other antibiotics.
The present study has described a cost-effective and environmentally friendly green synthesis of CuO NPs using Parthenium hysterophorus plant aqueous extracts as reducing and capping agents. The CuO NPs were characterized using UV–Vis spectroscopy, FTIR, SEM, TEM, XRD, and DLS analytical techniques. The UV–Vis spectrum revealed an SPR band at 340 nm attributable to CuO NPs. The FTIR analysis showed the presence of secondary metabolites in the extracts, which were responsible for reducing copper ion solutions into CuO NPs with characteristic stretching vibration at 522 cm −1 . SEM and TEM micrographs confirmed the spherical shape of CuO NPs. The XRD analysis confirmed the crystallinity of CuO NPs, and crystallite size was calculated to be 31.58 nm. DLS studies revealed polydispersity of CuO NPs. CuO NPs showed to be effective in degrading rifampicin antibiotics, with a degradation efficiency of over 98% being reported at optimal conditions. The rifampicin antibiotic degradation follows a pseudo-second-order kinetic model. Therefore, an eco-friendly and cost-effective Parthenium hysterophorus -mediated green synthesis of CuO NPs can be promising in the degradation of antibiotics in wastewater treatment plants and serve to address the increasing water pollution challenges. Future research should focus on the usability of CuO NPs in large-scale wastewater treatment and identifying actual degradation products and their implications for the ecosystem. We also note that toxic degradation products on the environmental compartments can further exacerbate water pollution hence the need to prevent their occurrence through encapsulation or making magnetic nanocomposite. This way, the safety of nanoparticles can be enhanced as they address environmental pollution.
Data availability
The data associated with this research study that is enough to draw the results and conclusions have been provided within the manuscript. However, all datasets have been deposited in the public repository, Zenodo, and are accessible via the link https://doi.org/10.5281/zenodo.8116191 .
Imminent risk of a global water crisis, warns the UN world water development report 2023. UNESCO . https://www.unesco.org/en/articles/imminent-risk-global-water-crisis-warns-un-world-water-development-report-2023 (2023).
Naddaf, M. The world faces a water crisis, and 4 powerful charts show how. Sci. Am . https://www.scientificamerican.com/article/the-world-faces-a-water-crisis-and-4-powerful-charts-show-how/ (2023).
Ongaji, P. Climate change to take centre stage at Africa health agenda international conference (AHAIC) in March 2023. https://ahaic.org/climate-change-to-take-centre-stage-at-africa-health-agenda-international-conference-ahaic-in-march-2023/ (2023).
Wilkinson, J. L. et al. Pharmaceutical pollution of the world’s rivers. Proc. Natl. Acad. Sci. U.S.A. 119 , 8. https://doi.org/10.1073/pnas.2113947119 (2022).
Article CAS Google Scholar
Patel, M. et al. Pharmaceuticals of emerging concern in aquatic systems: Chemistry, occurrence, effects, and removal methods. Chem. Rev. 119 (6), 3510–3673. https://doi.org/10.1021/acs.chemrev.8b00299 (2019).
Article CAS PubMed Google Scholar
De Ilurdoz, M. S., Sadhwani, J. J. & Reboso, J. V. Antibiotic removal processes from water a wastewater for the protection of the aquatic environment—A review. J. Water Process Eng. 45 , 102474. https://doi.org/10.1016/J.JWPE.2021.102474 (2022).
Article Google Scholar
Polianciuc, S. I., Gurzău, A. E., Kiss, B., Ștefan, M. G. & Loghin, F. Antibiotics in the environment: Causes and consequences. Med. Pharm. Rep. 93 (3), 231–240. https://doi.org/10.15386/mpr-1742 (2020).
Article PubMed PubMed Central Google Scholar
Hanna, N., Tamhankar, A. J. & Stålsby Lundborg, C. Antibiotic concentrations and antibiotic resistance in aquatic environments of the WHO Western Pacific and South-East Asia regions: A systematic review and probabilistic environmental hazard assessment. Lancet Planet. Health. 7 (1), e45–e54. https://doi.org/10.1016/S2542-5196(22)00254-6 (2023).
Article PubMed Google Scholar
Kairigo, P., Ngumba, E., Sundberg, L. R., Gachanja, A. & Tuhkanen, T. Occurrence of antibiotics and risk of antibiotic resistance evolution in selected Kenyan wastewaters, surface waters and sediments. Sci. Total Environ. https://doi.org/10.1016/J.SCITOTENV.2020.137580 (2020).
Kraemer, S. A., Ramachandran, A. & Perron, G. G. Antibiotic pollution in the environment: From microbial ecology to public policy. Microorganisms. 7 (6), 1–24. https://doi.org/10.3390/microorganisms7060180 (2019).
Wanakai, I. S. et al. Kinetics of rifampicin antibiotic degradation using green synthesized iron oxide nanoparticles. Chem. Afr. https://doi.org/10.1007/s42250-022-00543-w (2022).
Kulkarni, P. et al. Antibiotic concentrations decrease during wastewater treatment but persist at low levels in reclaimed water. Int. J. Environ. Res. Public Health. 14 (6), 1–13. https://doi.org/10.3390/ijerph14060668 (2017).
Larsson, D. G. J. & Flach, C. F. Antibiotic resistance in the environment. Nat. Rev. Microbiol. 20 (5), 257–269. https://doi.org/10.1038/s41579-021-00649-x (2022).
Grobbelaar, M. et al. Evolution of rifampicin treatment for tuberculosis. Infect. Genet. Evol. 74 , 31247337. https://doi.org/10.1016/j.meegid.2019.103937 (2019).
WHO. Global Tuberculosis Report 2022. https://www.who.int/teams/global-tuberculosis-programme/tb-reports/global-tuberculosis-report-2022 (2022).
Ngigi, A. N., Magu, M. M. & Muendo, B. M. Occurrence of antibiotics residues in hospital wastewater, wastewater treatment plant, and in surface water in Nairobi County, Kenya. Environ. Monit. Assess. 192 , 1. https://doi.org/10.1007/s10661-019-7952-8 (2020).
Derakhshan, Z. et al. Removal methods of antibiotic compounds from aqueous environments—A review. J. Environ. Health Sustain. Dev. 1 (1), 43–62 (2016).
Google Scholar
Kamaz, M. et al. Investigation into micropollutant removal from wastewaters by a membrane bioreactor. Int. J. Environ. Res. Public Health. 16 , 8. https://doi.org/10.3390/IJERPH16081363 (2019).
Nam, S. W., Yoon, Y., Chae, S., Kang, J. H. & Zoh, K. D. Removal of selected micropollutants during conventional and advanced water treatment processes. Environ. Eng. Sci. 34 (10), 752–761. https://doi.org/10.1089/EES.2016.0447 (2017).
Abdullah, et al. Green synthesis and characterization of copper and nickel hybrid nanomaterials: Investigation of their biological and photocatalytic potential for the removal of organic crystal violet dye. J. Saudi Chem. Soc. 26 (4), 101486. https://doi.org/10.1016/j.jscs.2022.101486 (2022).
Faisal, S. et al. Paraclostridium benzoelyticum bacterium-mediated zinc oxide nanoparticles and their in vivo multiple biological applications. Oxid. Med. Cell. Longev. https://doi.org/10.1155/2022/5994033 (2022).
Nzilu, D. M. et al. Environmental remediation using nanomaterial as adsorbents for emerging micropollutants. Environ. Nanotechnol. Monit. Manag. 20 , 100789. https://doi.org/10.1016/j.enmm.2023.100789 (2023).
Anjum, M., Miandad, R., Waqas, M., Gehany, F. & Barakat, M. A. Remediation of wastewater using various nano-materials. Arab. J. Chem. 12 (8), 4897–4919. https://doi.org/10.1016/J.ARABJC.2016.10.004 (2019).
Kokkinos, P., Mantzavinos, D. & Venieri, D. Current trends in the application of nanomaterials for the removal of emerging micropollutants and pathogens from water. Molecules https://doi.org/10.3390/MOLECULES25092016 (2020).
Abdullah, Al-Radadi, N. S., Hussain, T., Faisal, S. & Ali Raza Shah, S. Novel biosynthesis, characterization and bio-catalytic potential of green algae ( Spirogyra hyalina ) mediated silver nanomaterials. Saudi J. Biol. Sci. 29 (1), 411–419. https://doi.org/10.1016/j.sjbs.2021.09.013 (2022).
Khan, M. I. et al. Monotheca buxifolia driven synthesis of zinc oxide nano material its c characterization and biomedical applications. Micromachines. 13 (5), 1–16. https://doi.org/10.3390/mi13050668 (2022).
Article MathSciNet Google Scholar
Jan, H. et al. The Aquilegia pubiflora (Himalayan columbine) mediated synthesis of nanoceria for diverse biomedical applications. RSC Adv. 10 (33), 19219–19231. https://doi.org/10.1039/d0ra01971b (2020).
Article CAS PubMed PubMed Central ADS Google Scholar
Kainat, et al. Exploring the therapeutic potential of Hibiscus rosa sinensis synthesized cobalt oxide (Co 3 O 4 -NPs) and magnesium oxide nanoparticles (MgO-NPs). Saudi J. Biol. Sci. 28 (9), 5157–5167. https://doi.org/10.1016/j.sjbs.2021.05.035 (2021).
Article CAS PubMed PubMed Central Google Scholar
Sridevi, H., Bhat, M. R., Kumar, P. S., Kumar, N. M. & Selvaraj, R. Structural characterization of cuboidal α-Fe 2 O 3 nanoparticles synthesized by a facile approach. Appl. Nanosci. 13 (8), 5605–5613. https://doi.org/10.1007/s13204-023-02780-y (2023).
Article CAS ADS Google Scholar
Faisal, S. et al. Green synthesis of zinc oxide (ZnO) nanoparticles using aqueous fruit extracts of Myristica fragran s: Their characterizations and biological and environmental applications. ACS Omega 6 (14), 9709–9722. https://doi.org/10.1021/acsomega.1c00310 (2021).
Faisal, S. et al. Curcuma longa mediated synthesis of copper oxide, nickel oxide and Cu–Ni bimetallic hybrid nanoparticles: Characterization and evaluation for antimicrobial, anti-parasitic and cytotoxic potentials. Coatings 11 (7), 1–22. https://doi.org/10.3390/coatings11070849 (2021).
Article MathSciNet CAS Google Scholar
Faisal, S. et al. Bio-catalytic activity of novel mentha arvensis intervened biocompatible magnesium oxide nanomaterials. Catalysts 11 (7), 1–18. https://doi.org/10.3390/catal11070780 (2021).
Shah, S. et al. Engineering novel gold nanoparticles using Sageretia thea leaf extract and evaluation of their biological activities. J. Nanostruct. Chem. 12 (1), 129–140. https://doi.org/10.1007/s40097-021-00407-8 (2022).
Andualem, W. W., Sabir, F. K., Mohammed, E. T., Belay, H. H. & Gonfa, B. A. Synthesis of copper oxide nanoparticles using plant leaf extract of catha edulis and its antibacterial activity. J. Nanotechnol. https://doi.org/10.1155/2020/2932434 (2020).
Alhalili, Z. Green synthesis of copper oxide nanoparticles CuO NPs from Eucalyptus Globulus leaf extract: Adsorption and design of experiments. Arab. J. Chem. 15 (5), 103739. https://doi.org/10.1016/j.arabjc.2022.103739 (2022).
Mali, S. C., Dhaka, A., Githala, C. K. & Trivedi, R. Green synthesis of copper nanoparticles using Celastrus paniculatus willd. leaf extract and their photocatalytic and antifungal properties. Biotechnol. Rep. https://doi.org/10.1016/j.btre.2020.e00518 (2020).
Abosede, O. O. & Obiyenwa, G. K. Green synthesis of copper oxide nanoparticles using Justicia Carnea . Int. J. Chem. Stud. 10 (2), 21–25. https://doi.org/10.22271/chemi.2022.v10.i2a.12176 (2022).
Afzal, G. et al. Copper oxide nanoparticles synthesis using Aerva Javanica and their antimicrobial activities. J. Anim. Plant Sci. 32 (5), 1403–1411. https://doi.org/10.36899/JAPS.2022.5.0547 (2022).
Olusegun, S. J., Larrea, G., Osial, M., Jackowska, K. & Krysinski, P. Photocatalytic degradation of antibiotics by superparamagnetic iron oxide nanoparticles tetracycline case. Catalysts https://doi.org/10.3390/catal11101243 (2021).
You, J., Zhang, X. & Chen, J. Degradation of antibiotics by fenton-like reaction catalyzed by iron oxide. Adv. Mater. Sci. Eng. (2022).
Zhou, L., Guo, X., Lai, C. & Wang, W. Electro-photocatalytic degradation of amoxicillin using calcium titanate. Open Chem. 16 (1), 949–955. https://doi.org/10.1515/chem-2018-0108 (2018).
Malakootian, M., Nasiri, A. & Gharaghani, M. A. Photocatalytic degradation of ciprofloxacin antibiotic by TiO 2 nanoparticles immobilized on a glass plate. Chem. Eng. Commun. 207 (1), 56–72. https://doi.org/10.1080/00986445.2019.1573168 (2020).
Parmar, N. & Srivastava, J. K. Degradation of pharmaceutical antibiotic (ciprofloxacin) by photocatalysis process using sol-gel based titanium dioxide nanoparticles. Int. J. Chem. React. Eng. 19 (9), 929–938. https://doi.org/10.1515/ijcre-2021-0076 (2021).
Pham, T. D. et al. Synthesis and characterization of novel core-shell ZnO@SiO 2 nanoparticles and application in antibiotic and bacteria removal. ACS Omega 7 (46), 42073–42082. https://doi.org/10.1021/acsomega.2c04226 (2022).
Du, J. et al. The potential hazards and ecotoxicity of CuO nanoparticles: an overview. Toxin Rev. 40 (4), 460–472. https://doi.org/10.1080/15569543.2019.1670211 (2021).
Rana, S. & Kalaichelvan, P. T. Ecotoxicity of nanoparticles. ISRN Toxicol. 1–11 (2013).
Matzrafi, M., Raz, H., Rubin, B., Yaacoby, T. & Eizenberg, H. Distribution and biology of the invasive weed Parthenium hysterophorus L. in Israel. Front. Agron. 3 , 1–12. https://doi.org/10.3389/fagro.2021.639991 (2021).
Kaur, L. et al. Comprehensive review on ethnobotanical uses, phytochemistry, biological potential and toxicology of Parthenium hysterophorus L.: A journey from noxious weed to a therapeutic medicinal plant. J. Ethnopharmacol. 281 , 114525. https://doi.org/10.1016/J.JEP.2021.114525 (2021).
Saini, A., Aggarwal, N. K., Sharma, A., Kaur, M. & Yadav, A. Utility potential of Parthenium hysterophorus for its strategic management. Adv. Agric. https://doi.org/10.1155/2014/381859 (2014).
Pati, U. K. & Chowdhury, A. Study of Parthenium hysterophorus L. extracts (first clean-up fractions) on seed germination behaviour in search of bioactive fractions for preparation of bioherbicide formulations. Int. Lett. Nat. Sci. 49 , 69–80. https://doi.org/10.18052/WWW.SCIPRESS.COM/ILNS.49.69 (2015).
Bezuneh, T. T. Phytochemistry and antimicrobial activity of Parthenium hysterophorus L.: A review. Sci. J. Anal. Chem. 3 (3), 30. https://doi.org/10.11648/J.SJAC.20150303.11 (2015).
Datta, A. et al. Green synthesis of zinc oxide nanoparticles using Parthenium hysterophorus leaf extract and evaluation of their antibacterial properties. J. Biotechnol. Biomater. 7 (03), 2–8. https://doi.org/10.4172/2155-952x.1000271 (2017).
Jayachandran, A., Aswathy, T. R. & Nair, A. S. Green synthesis and characterization of zinc oxide nanoparticles using Cayratia pedata leaf extract. Biochem. Biophys. Rep. 26 , 100995. https://doi.org/10.1016/j.bbrep.2021.100995 (2021).
Rani Verma, P. & Khan, F. Green approach for biofabrication of CuO nanoparticles from Prunus amygdalus pericarp extract and characterization. Inorg. Nano-Metal Chem. 49 (3), 69–74. https://doi.org/10.1080/24701556.2019.1601738 (2019).
Kian, L. K., Jawaid, M., Ariffin, H. & Alothman, O. Y. Isolation and characterization of microcrystalline cellulose from roselle fibers. Int. J. Biol. Macromol. 103 , 931–940. https://doi.org/10.1016/j.ijbiomac.2017.05.135 (2017).
Madivoli, E. S., Kareru, P. G., Gachanja, A. N., Mugo, S. M. & Makhanu, D. S. Synthesis and characterization of dialdehyde cellulose nanofibers from O. sativa husks. SN Appl. Sci. 1 (7), 1–7. https://doi.org/10.1007/s42452-019-0769-9 (2019).
Otenda, B. V. et al. Starch-hibiscus-cellulose nanofibrils composite films as a model antimicrobial food packaging material. J. Nat. Fibers. https://doi.org/10.1080/15440478.2022.2058674 (2022).
Madivoli, E. et al. Adsorption of selected heavy metals on modified nano cellulose. Int. Res. J. Pure Appl. Chem. 12 (3), 1–9. https://doi.org/10.9734/IRJPAC/2016/28548 (2016).
Madivoli, E. S., Kareru, P. G. & Fromm, K. Versatility of cellulose stimuli responsive membranes in the detection of dimethylamine. https://doi.org/10.5281/ZENODO.7392545 (2022).
Madivoli, E. S. et al. Synthesis of spherical titanium dioxide microspheres and its application to degrade rifampicin. Environ. Nanotechnol. Monit. Manag. https://doi.org/10.1016/j.enmm.2020.100327 (2020).
Sadia Aroob, M. S. et al. Green synthesis and photocatalytic dye degradation activity of CuO nanoparticles. Catalysis. 13 (4), 1–8. https://doi.org/10.3390/catal13030502 (2023).
Akintelu, S. A., Folorunso, A. S., Folorunso, F. A. & Oyebamiji, A. K. Green synthesis of copper oxide nanoparticles for biomedical application and environmental remediation. Heliyon. 6 (7), e04508. https://doi.org/10.1016/j.heliyon.2020.e04508 (2020).
Atri, A. et al. Green synthesis of copper oxide nanoparticles using Ephedra alata plant extract and a study of their antifungal, antibacterial activity and photocatalytic performance under sunlight. Heliyon. 9 (2), 1–16. https://doi.org/10.1016/j.heliyon.2023.e13484 (2023).
Faisal, S. et al. Green synthesis of zinc oxide (ZnO) nanoparticles using aqueous fruit extracts of Myristica fragrans : Their characterizations and biological and environmental applications. https://doi.org/10.1021/acsomega.1c00310 (2021).
Renuga, D., Jeyasundari, J., Shakthi Athithan, A. S. & Brightson Arul Jacob, Y. Synthesis and characterization of copper oxide nanoparticles using Brassica oleracea var. italic extract for its antifungal application. Mater. Res. Express https://doi.org/10.1088/2053-1591/ab7b94 (2020).
Amjad, R. et al. Green synthesis and characterization of copper nanoparticles using fortunella margarita leaves. Polymers (Basel). 13 (24), 1–12. https://doi.org/10.3390/polym13244364 (2021).
Altikatoglu, M., Attar, A., Erci, F., Cristache, C. M. & Isildak, I. Green synthesis of copper oxide nanoparticles using ocimum basilicum extract and their antibacterial activity. Fresenius Environ. Bull. 25 (12), 7832–7837 (2017).
Amin, F. et al. Green synthesis of copper oxide nanoparticles using Aerva javanica Leaf extract and their characterization and investigation of in vitro antimicrobial potential and cytotoxic activities. Evid. Based Complement. Altern. Med. https://doi.org/10.1155/2021/5589703 (2021).
Bhatnagar, S., Kobori, T., Ganesh, D., Ogawa, K. & Aoyagi, H. Biosynthesis of silver nanoparticles mediated by extracellular pigment from Talaromyces purpurogenus and their biomedical applications. Nanomaterials 9 (7), 1–27. https://doi.org/10.3390/nano9071042 (2019).
Chand Mali, S., Raj, S. & Trivedi, R. Biosynthesis of copper oxide nanoparticles using Enicostemma axillare (Lam.) leaf extract. Biochem. Biophys. Rep. 2 , 100699. https://doi.org/10.1016/j.bbrep.2019.100699 (2019).
Faisal, S. et al. In vivo analgesic, anti-Inflammatory, and anti-diabetic screening of Bacopa monnieri -synthesized copper oxide nanoparticles. ACS Omega 7 (5), 4071–4082. https://doi.org/10.1021/acsomega.1c05410 (2022).
Chowdhury, R., Khan, A. & Rashid, M. H. Green synthesis of CuO nanoparticles using: Lantana camara flower extract and their potential catalytic activity towards the aza-Michael reaction. RSC Adv. 10 (24), 14374–14385. https://doi.org/10.1039/d0ra01479f (2020).
Carvalho, P. M., Felício, M. R. & Santos, N. C., Gonçalves, S. & Domingues, M. M.,. Application of light scattering techniques to nanoparticle characterization and development. Front. Chem. 6 , 1–17. https://doi.org/10.3389/fchem.2018.00237 (2018).
Raval, N. et al. Importance of physicochemical characterization of nanoparticles in pharmaceutical product development. Basic Fundam. Drug Deliv. https://doi.org/10.1016/B978-0-12-817909-3.00010-8 (2019).
Aksu Demirezen, D., Yıldız, Y. S. & Demirezen Yılmaz, D. Amoxicillin degradation using green synthesized iron oxide nanoparticles: Kinetics and mechanism analysis. Environ. Nanotechnol. Monit. Manag. 11 , 100219. https://doi.org/10.1016/j.enmm.2019.100219 (2019).
Kutuzova, A., Dontsova, T. & Kwapinski, W. Application of TiO2-based photocatalysts to antibiotics degradation: Cases of sulfamethoxazole, trimethoprim and ciprofloxacin. Catalysts https://doi.org/10.3390/catal11060728 (2021).
El-Sayed, G. O., Dessouki, H. A. & Ibrahiem, S. S. Photocatalytic degradation of metronidazole in aqueous solutions by copper oxide nanoparticles. J. Basic Environ. Sci. 1 , 102–110 (2014).
Kumar, A. A review on the factors affecting the photocatalytic degradation of hazardous materials. Mater. Sci. Eng. Int. J. 1 (3), 106–114. https://doi.org/10.15406/mseij.2017.01.00018 (2017).
Nabilah Mohd Noor, N., Hazirah Kamaruzaman, N., Al-Gheethi, A., Maya Saphira Radin, M. & Hossain, M. S. Degradation of antibiotics in aquaculture wastewater by bio-nanoparticles: A critical review. Ain Shams Eng. J. 14 (7), 101981. https://doi.org/10.1016/j.asej.2022.101981 (2022).
Usman, M. R. et al. Ceftriaxone degradation by titanium dioxide (TiO 2 ) nanoparticles: Toxicity and degradation mechanism. J. Kim. Val. 6 (1), 82–89. https://doi.org/10.15408/jkv.v6i1.12475 (2020).
Cai, W., Weng, X. & Chen, Z. Highly efficient removal of antibiotic rifampicin from aqueous solution using green synthesis of recyclable nano-Fe 3 O 4 . Environ. Pollut. 247 , 839–846. https://doi.org/10.1016/j.envpol.2019.01.108 (2019).
Kansal, S. K. et al. Photocatalytic degradation of the antibiotic levofloxacin using highly crystalline TiO 2 nanoparticles. New J. Chem. 38 (7), 3220–3226. https://doi.org/10.1039/c3nj01619f (2014).
Hamad Hussein, M. T. M. & El-Sessy, M. E. Adsorptive removal of levofloxacin and antibiotic resistance genes from hospital wastewater by nano-zero-valent iron and nano-copper using kinetic studies and response surface methodology. Bioresour. Bioprocess. 10 (1), 1–29. https://doi.org/10.1186/s40643-022-00616-1 (2023).
Xu, Q., Owens, G. & Chen, Z. Adsorption and catalytic reduction of rifampicin in wastewaters using hybrid rGO@Fe/Pd nanoparticles. J. Clean. Prod. 264 , 121617. https://doi.org/10.1016/j.jclepro.2020.121617 (2020).
Bai, X. et al. Photocatalytic degradation of some typical antibiotics: Recent advances and future outlooks. Int. J. Mol. Sci. 23 , 15. https://doi.org/10.3390/ijms23158130 (2022).
Akhil, D., Lakshmi, D., Senthil Kumar, P., Vo, D. N. & Kartik, A. Occurrence and removal of antibiotics from industrial wastewater. Springer Int. Publ. https://doi.org/10.1007/s10311-020-01152-0 (2021).
Shelke, H. D. et al. Multifunctional Cu 2 SnS 3 nanoparticles with enhanced photocatalytic dye degradation and antibacterial activity. Materials (Basel). https://doi.org/10.3390/ma15093126 (2022).
Kaushal, S., Kumar, A., Bains, H. & Singh, P. P. Photocatalytic degradation of tetracycline antibiotic and organic dyes using biogenic synthesized CuO/Fe 2 O 3 nanocomposite: Pathways and mechanism insights. Environ. Sci. Pollut. Res. 30 (13), 37092–37104 (2023).
Download references
Acknowledgements
The authors are thankful to the Department of Chemistry, College of Pure and Applied Sciences, Jomo Kenyatta University of Agriculture and Technology, for providing laboratory facilities for conducting the study. The authors are also thankful to the Federal Commission of Scholarships (FCS) for a one-year exchange program at the University of Fribourg, Switzerland and the Swiss National Science Foundation under grant number IZSEZ0_200290 that enabled access to various analytical instruments at Prof. Katharina Fromm Laboratory, Chemistry Department, University of Fribourg.
No funding was provided during the preparation of this manuscript.
Author information
Authors and affiliations.
Chemistry Department, Jomo Kenyatta University of Agriculture and Technology, P.O. Box 62000, 00200, Nairobi, Kenya
Dennis Mwanza Nzilu, Edwin Shigwenya Madivoli, Sammy Indire Wanakai, Gideon Kirui Kiprono & Patrick Gachoki Kareru
Department of Biological and Physical Sciences, Karatina University, P.O. Box 1957-10101, Karatina, Kenya
David Sujee Makhanu
You can also search for this author in PubMed Google Scholar
Contributions
All the authors contributed to the study conception and design. Material preparation, data collection, and analysis were drafted by D.M.N. D.M.N. wrote the first draft, and all authors commented on previous versions of the manuscript. All the authors have read and approved the final manuscript.
Corresponding author
Correspondence to Dennis Mwanza Nzilu .
Ethics declarations
Competing interests.
The authors declare no competing interests.
Additional information
Publisher's note.
Springer Nature remains neutral with regard to jurisdictional claims in published maps and institutional affiliations.
Rights and permissions
Open Access This article is licensed under a Creative Commons Attribution 4.0 International License, which permits use, sharing, adaptation, distribution and reproduction in any medium or format, as long as you give appropriate credit to the original author(s) and the source, provide a link to the Creative Commons licence, and indicate if changes were made. The images or other third party material in this article are included in the article's Creative Commons licence, unless indicated otherwise in a credit line to the material. If material is not included in the article's Creative Commons licence and your intended use is not permitted by statutory regulation or exceeds the permitted use, you will need to obtain permission directly from the copyright holder. To view a copy of this licence, visit http://creativecommons.org/licenses/by/4.0/ .
Reprints and permissions
About this article
Cite this article.
Nzilu, D.M., Madivoli, E.S., Makhanu, D.S. et al. Green synthesis of copper oxide nanoparticles and its efficiency in degradation of rifampicin antibiotic. Sci Rep 13 , 14030 (2023). https://doi.org/10.1038/s41598-023-41119-z
Download citation
Received : 29 June 2023
Accepted : 22 August 2023
Published : 28 August 2023
DOI : https://doi.org/10.1038/s41598-023-41119-z
Share this article
Anyone you share the following link with will be able to read this content:
Sorry, a shareable link is not currently available for this article.
Provided by the Springer Nature SharedIt content-sharing initiative
This article is cited by
Antifungal activity of dialdehyde chitosan against aspergillus brasiliensis and candida albicans.
- Gideon Kiprono Kirui
- Edwin Shigwenya Madivoli
- Walyambillah Waudo
Biomass Conversion and Biorefinery (2024)
Influence of cobalt doping on structural and optical properties of copper oxide expected as an inorganic hole transport layer for perovskite solar cell
- Noor M. Abdulmalek
- Hussein A. Jawad
Journal of Materials Science: Materials in Electronics (2024)
Green synthesis of copper nanoparticles by using pineapple peel waste: in vitro characterizations and antibacterial potential
- Sanchita Mitra
- Tarun Kumar Dua
- Paramita Paul
Bioprocess and Biosystems Engineering (2024)
An evaluation of antimicrobial potency of silver nanoparticles synthesised from Fusarium sp.
- Ngunjiri Vincent Mwangi
- Shigwenya Edwin Madivoli
Discover Applied Sciences (2024)
Photoresist Performance: An Exploration of Synthesis, Surface Modification Techniques, Properties Tailoring, and Challenges Navigation in Copper/Copper Oxide Nanoparticle Applications
- Asishana Paul Onivefu
- Adeyemi Efunnuga
- Stanley O. Omorogbe
Biomedical Materials & Devices (2024)
By submitting a comment you agree to abide by our Terms and Community Guidelines . If you find something abusive or that does not comply with our terms or guidelines please flag it as inappropriate.
Quick links
- Explore articles by subject
- Guide to authors
- Editorial policies
Sign up for the Nature Briefing newsletter — what matters in science, free to your inbox daily.


An official website of the United States government
The .gov means it’s official. Federal government websites often end in .gov or .mil. Before sharing sensitive information, make sure you’re on a federal government site.
The site is secure. The https:// ensures that you are connecting to the official website and that any information you provide is encrypted and transmitted securely.
- Publications
- Account settings
Preview improvements coming to the PMC website in October 2024. Learn More or Try it out now .
- Advanced Search
- Journal List
- Turk J Pharm Sci
- v.17(4); 2020 Aug


Green Synthesis and Characterization of Copper Nanoparticles and Their Effects on Liver Function and Hematological Parameters in Mice
Mehrdad khatami.
1 Student Research Committee, Bam University of Medical Sciences, Bam, Iran
Katrin EBRAHIMI
2 Department of Biology, Payame Noor University, Tehran, Iran
Nasrin GALEHDAR
3 Social Determinants of Health Research Center, Lorestan University of Medical Sciences, Khorramabad, Iran
Mohammad Nabi MORADI
4 Razi Herbal Medicines Research Center, Lorestan University of Medical Sciences, Khorramabad, Iran
Alireza MOAYYEDKAZEMI
5 Department of Internal Medicine, Lorestan University of Medical Sciences, Khorramabad, Iran
Objectives:
The present investigation is based on the green synthesis of copper nanoparticles (CuNPs) from aqueous extract of Capparis spinosa L. fruit. Their effects on liver function and hematological parameters in mice were evaluated.
Materials and Methods:
The green synthesis of CuNPs by means of C. spinosa extract was achieved. Ultraviolet-visible spectroscopy, fourier transform infrared (FTIR) spectroscopy, scanning electron microscopy (SEM), and energy dispersive X-ray spectroscopy were used to identify the synthesized nanoparticles. BALB/c mice were orally administrated CuNPs at doses of 1000, 2000, and 5000 μg/kg for 2 weeks. Later, the effects of CuNPs on liver function in the treated mice were evaluated by measuring the serum levels of enzymes such as alanine aminotransferase, aspartate aminotransferase, alkaline phosphatase, and bilirubin as well as hematological parameters including hemoglobin, hematocrit, white blood cell, red blood cell, and platelet counts.
A maximum peak at wavelength 414 nm confirmed the biosynthesis of CuNPs. FTIR spectrum analysis revealed that the factor groups shaped a coating extract on the surface of the nanoparticles. SEM images demonstrated a particle size between 17 and 41 nm. Although some liver enzymes and hematological parameters increased with increasing dose of extract, there was no significant difference (p>0.05) between oral administrations of CuNPs at doses of 1000, 2000, and 5000 μg/kg and the control group.
Conclusion:
The findings revealed that CuNPs biosynthesized from aqueous extract of C. spinosa fruit have no toxic effects on the liver functions and hematological parameters of mice. However, more studies are needed for evaluation of the hepatoprotective effects of CuNPs.
INTRODUCTION
Nanotechnology is one of the most useful technologies and it can be applied in many areas including food and nutrition, biomedical science, gene transmission, energy science, electronics, and the space industry. In particular, this technology is implemented in the treatment of cancer, allergies, inflammation, diabetes, and other diseases. 1
There are various physical and chemical methods for the production of nanoparticles, and they are still being investigated for the purpose of obtaining particles with a certain size and lower toxicity. 2 Green synthesis is considered a new approach to prevent the production of undesired or unsafe by products via reliable, maintainable, and eco-favorable synthesis techniques. Between the current green procedures of synthesis of nanoparticles, use of plant extracts is an appropriate and easy method to harvest nanoparticles on a large scale relative to bacteria and/or fungi mediated synthesis. 2 , 3 Recent studies have also shown that the synthesis of metal nanoparticles using plant extracts, i.e. green synthesis, has some benefits such as low cost and low toxic effects for large-scale production. 2 , 3 , 4
Copper (Cu) is one of the most useful elements in medical science because of its numerous anti-inflammatory, anticancer, analgesic, and antimicrobial effects. 5 In recent years, it has been proven that, because of their high surface-to-volume ratio, Cu nanoparticles (CuNPs) are extremely reactive and simply interact with other particles, leading to a wide range of biological activities. 5 , 6 , 7
Previous reviews on laboratory animals have demonstrated that the liver is considered the key target tissue of drug toxicity. Hence, assessing the function of this organ is a very important method to determine drug toxicity. 8 Nowadays, one of the main criteria to determine liver damage is measuring serum levels of enzymes such as alanine aminotransferase (ALT), aspartate aminotransferase (AST), alkaline phosphatase (ALP), and bilirubin. 9 , 10 , 11 It has been reported that oral consumption of some medicinal agents may affect the hematological parameters and result in anemia, neutropenia, and thrombocytopenia; therefore, it is necessary to evaluate the effects of novel medicinal drugs by measuring hematological parameters. 10 Accordingly, the present study examined the green synthesis of CuNPs from Capparis spinosa fruit extract and evaluated their effects on liver function and hematological parameters in mice.
MATERIALS AND METHODS
Green synthesis of copper nanoparticles.
Fruits of C. spinosa were collected from rural areas in western Iran and then were extracted by percolation procedure by means of methanol (80%) for 3 days at room temperature. 12 In the next step, the green synthesis of CuNPs was performed according to a method described elsewhere. Briefly, 75 mL of the obtained extract was added to 100 mL of 0.01 M Cu sulfate solution. After stirring, it was kept at 60°C for 1 day. In the next step, to remove all impurities, it was centrifuged twice at 12.000 rpm for 20 min. Nanoparticles started to deposit when the color of the solution changed from green to amber yellow. The synthesized nanoparticles were heat treated in an oven at 60°C for further analyses.
Ultraviolet-visible (UV-Vis) spectroscopy analysis
Transformation of the Cu ions to CuNPs was shown by the surface plasmon resonance (SPR) of the CuNPs. For this purpose, 0.3 mL of the specimens was diluted with 3 mL of normal saline and studied via UV-Vis spectrum analysis by means of a spectrophotometer (JENWAY 6405) in the range of 300-700 nm. 13
Fourier transform infrared (FTIR) spectroscopy
After pouring and mixing the obtained samples and potassium bromide granules together in a ratio of 1 to 100 (1/100 ratio) and compacting them into tablets, FTIR (model Nicolet 32) analysis was carried out in the range of 400-4000 cm -1 and with the resolution of 1-4 cm -1 . 14
Scanning electron microscope (SEM)
To obtain the characteristics of the synthesized nanoparticles, electron microscopy (Mira3, Czech Republic) with 15 kV, magnification of 10x, and resolution of 1 nm was performed.
Animals and study design
A total of 32 male BALB/c mice weighing 25-30 g were provided by the Tehran Pasteur Institute and kept in light/dark cycles of 12:12 h. The room temperature was 22±2°C and the mice had ad libitum access to water and food. They were placed in laboratory conditions 30 min before the start of the experiment. The ethical approval required for this study (IR.LUMS.REC.1398.247) was issued by the Ethics Committee of Lorestan University of Medical Sciences, Lorestan, Iran. The mice tested were assigned to the following four groups:
Group i: Received normal saline orally for 14 days;
Group ii: Received CuNPs at a concentration of 1000 µg/kg orally for 14 days;
Group iii: Received CuNPs at a concentration of 2000 µg/kg orally for 14 days;
Group iv: Received CuNPs at a concentration of 5000 µg/kg orally for 14 days.
Sample collection
On day 15 of the experiments, the mice were anesthetized by ketamine-xylazine followed by collection of blood samples from each mouse after the heart was opened. The collected blood samples were put into tubes with or without anticoagulant to process their clots and then their sera were separated by centrifugation at 5000x g for 10 min.
Evaluation of the serum liver enzymes
To determine the hepatoprotective effects of CuNPs, different clinical chemistry parameters related to liver function such as AST, ALT, ALP, and bilirubin (direct and total) were assayed by commercial diagnostics kits (Roche, Germany). 10 , 15
Hematological parameters
To assess the effects of CuNPs on hematological studies, total collected blood was put into tubes containing ethylenediaminetetraacetic acid. Next, hematological parameters including hemoglobin, hematocrit, white blood cell, red blood cell, and platelet (PLT) counts were measured by Sysmex (KX-21, Japan).
Statistical analysis
SPSS version 17.0 (SPSS Inc., Chicago, IL, USA) was used to analyze the obtained data. One-Way ANOVA and Tukey’s post-hoc test were performed to assess the difference between the experimental groups and p<0.05 was considered statistically significant.
Ultraviolet-visible spectrum analysis
The results obtained showed that the highest peak of the synthesized CuNPs was found at a wavelength of 414 nm. The characteristic of the resonance band of the SP happened for CuNPs at 414 nm wavelength ( Figure 1 ).

The absorption spectrum of synthesized copper nanoparticles
Fourier transform infrared analysis
The FTIR findings revealed that the biomolecules in the extract decreased the Cu sulfate solution. The bands at 3380, 2928, 1741, 1604, 1400, 1050, and 1271 were indexed to the O-H stretching of alcohol and phenol, C-H stretching of the aliphatic group, C=O stretching of ester carbonyl, C=C stretching of the aromatic ring, and C-O stretching of ester, respectively ( Figure 2 ).

The FTIR spectrum of synthesized copper nanoparticles
FTIR: Fourier transform infrared
Scanning electron microscope analysis
Following the confirmation of the synthesized nanoparticles through color modification and Vis-UV and FTIR, the characterization of nanoparticles was determined with SEM. As shown in Figure 1 , the synthesized CuNPs represent spherical morphology, whereas the size of the particles was between 17 and 41 nm ( Figure 3 ).

Scanning electron microscope images of copper nanoparticles synthesized using aqueous extract of Capparis spinosa fruit x10000
Hepatoprotective effects of CuNPs
Table 1 shows the results of hepatoprotective effects on serum biochemical parameters in mice receiving CuNPs at doses of 1000, 2000, and 5000 µg/kg for 14 days. As can be seen, although these parameters increased with increasing doses of extract, there was no statistically significant difference (p>0.05) between oral administrations of CuNPs at doses of 1000, 2000, and 5000 µg/kg and the control group.

Effect on hematological parameters
As shown in Table 2 , following the oral administrations of CuNPs at the employed doses of 1000, 2000, and 5000 µg/kg for 14 days, there was no significant difference (p<0.05) between hematological parameters compared with the control group.

In recent years, studies in the field of nanotechnology have demonstrated that physical and chemical procedures to create nanoparticles, despite having exceptional biological activities, due to having some restrictions like toxicity, are replaced by a number of new methods such as green synthesis. 1 Today, considering that nanoparticles are broadly used by a large proportion of the world’s population to treat some diseases, it is necessary to measure the toxicity of these products by different methods. Since recent studies have shown that CuNPs possess a wide range of biological activities, 16 we decided to evaluate their toxicity and hepatoprotective effects in mice.
Recent studies have shown that liver enzyme measurement is one of the key diagnostic tests to evaluate the liver function and also inflammations and damage such as hepatitis and cirrhosis. 8 , 10 CuNPs, because of their high surface-to-volume ratio, are extremely reactive and simply interact with other particles. Therefore, they have numerous biological activities. 17 , 18 It has been proven that some medications can cause irreparable complications through reducing white and red blood cells and the number of blood PLTs; thus, it is necessary to evaluate the effects of novel medicinal drugs by measuring hematological parameters. 19
Based on the results of the present study, after oral administration of mice with CuNPs at doses of 1000, 2000, and 5000 µg/kg for 14 days, although some parameters increased with increasing dose of extract, there was no statistically significant difference (p>0.05) between oral administrations of CuNPs at these doses and the control group. Moreover, no statistically significant difference (p<0.05) was observed in hematological parameters between mice treated with CuNPs and the control group.
Considering the study of the hepatoprotective effects of nanoparticles and similar to our findings, Zhang et al. 20 demonstrated that silver nanoparticles synthesized using Rhizophora apiculata were effective in protecting the liver from harm induced by carbon tetrachloride.
Bhuvaneswari et al. 21 reported that increased enzymatic levels of AST, ALT, ALP, and bilirubin by CCl 4 returned to normal when treated with silver nanoparticles synthesized using fruit extract of Embilica officinalis , indicating potent hepatoprotective effects of these nanoparticles. In the study conducted by Eftekhari et al. 22 , quercetin nanoparticles showed remarkable hepatoprotective activity via decreasing levels of AST, ALT, and ALP. Ghosh and Gomes 23 also recently showed that gold nanoparticles synthesized by Trigonella foenum-graecum extract significantly normalized the increased enzymatic levels of AST, ALT, ALP, and bilirubin induced by CCl 4 , which indicated the hepatoprotective potential of these nanoparticles.
In the present study, we applied C. spinosa to facilitate the synthesis of CuNPs. Next, we synthesized CuNPs that were spherical and ranging from 17 and 41 nm in size. To date, a wide range of plants such as Syzygium aromaticum , Nerium oleander , Citrus medica Linn., Capparis zeylanica , Gloriosa superba L., and Vitis vinifera have been applied in the biosynthesis of CuNPs. However, identifying the plant’s capacity as biological material for the synthesis of nanoparticles in full detail requires more investigations. 5 , 6 , 17 , 24 , 25 , 26 , 27
The findings revealed that CuNPs biosynthesized from aqueous extract of C. spinosa fruit have no toxic effect on the liver of the studied mice, and no significant toxicity was observed in their hematological parameters. However, more studies need to be done for evaluation of the hepatoprotective effect of CuNPs.
Acknowledgments
The authors are thankful to the staff of Razi Herbal Medicines Research Center, Khorramabad, Iran as well as the staff of Bam University of Medical Sciences, Bam, Iran.
Conflicts of interest: No conflict of interest was declared by the authors. The authors alone are responsible for the content and writing of the paper.
Click through the PLOS taxonomy to find articles in your field.
For more information about PLOS Subject Areas, click here .
Loading metrics
Open Access
Peer-reviewed
Research Article
Green synthesis and characterization of copper nanoparticles for investigating their effect on germination and growth of wheat
Contributed equally to this work with: Humaira Kausar, Ansar Mehmood
Roles Conceptualization, Investigation
Affiliation Department of Botany, University of Poonch Rawalakot, Azad Kashmir, Pakistan
Roles Conceptualization, Supervision, Writing – original draft, Writing – review & editing
* E-mail: [email protected] , [email protected]

Roles Formal analysis
¶ ‡ RTK, KSA, SH, FN, MSI, MN and TSU also contributed equally to this work.
Affiliation Department of Botany, the University of Azad Jammu and Kashmir (UAJK), Muzaffarabad, Pakistan
Roles Methodology, Writing – review & editing
Roles Validation
Roles Software
Current address: Institute of Crop Science (340 h), University of Hohenheim, Stuttgart, Germany
Affiliation Department of Agronomy, MNS University of Agriculture Multan, Punjab, Pakistan
Affiliation Department of Botany, University of Gujrat, Punjab, Pakistan
Roles Resources
Affiliation Department of Botany, University of Kotli, Azad Jammu and Kashmir, Pakistan
- Humaira Kausar,
- Ansar Mehmood,
- Rizwan Taj Khan,
- Khawaja Shafique Ahmad,
- Sajjad Hussain,
- Fahim Nawaz,
- Muhammad Sajjad Iqbal,
- Muhammad Nasir,
- Tariq Saif Ullah
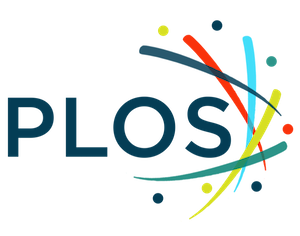
- Published: June 21, 2022
- https://doi.org/10.1371/journal.pone.0269987
- Reader Comments
Today, different types of nanoparticles (NPs) are being synthesized and used for medical and agricultural applications. In this study, copper nanoparticles (CuNPs) were synthesized using the aqueous extract of mint ( Mentha longifolia L.). For the characterization of CuNPs, UV-visible spectroscopy, scanning electron microscopy, X-ray diffraction, and Fourier transform infrared spectrometry were used. The UV-Visible absorption peak at 558 nm confirmed the formation of CuNPs. The XRD pattern confirmed the phase-centered crystalline nature of CuNPs. FTIR analysis showed the O-H, Cu-H and C-C bonds, indicating the active role of these functional groups as reducing agents of Cu ions to CuNPS. The synthesized NPs were found to have an almost spherical shape with an average size of 23 nm. When applied to wheat, a condition dependent effect of CuNPs was found. Variety 18-Elite Line 1, Elite Line 3, and 18-Elite Line 6 showed maximum germination and growth rate at 50 mg CuNPs/L, while variety 18-Elite Line 5 showed that increase at 25 mg CuNPs/L. Beyond these concentrations, the seed germination and growth of wheat declined. In conclusion, the application of CuNPs showed a beneficial effect in improving the growth of wheat at a certain concentration.
Citation: Kausar H, Mehmood A, Khan RT, Ahmad KS, Hussain S, Nawaz F, et al. (2022) Green synthesis and characterization of copper nanoparticles for investigating their effect on germination and growth of wheat. PLoS ONE 17(6): e0269987. https://doi.org/10.1371/journal.pone.0269987
Editor: Mohammad Shahid, Aligarh Muslim University, INDIA
Received: February 18, 2022; Accepted: June 1, 2022; Published: June 21, 2022
Copyright: © 2022 Kausar et al. This is an open access article distributed under the terms of the Creative Commons Attribution License , which permits unrestricted use, distribution, and reproduction in any medium, provided the original author and source are credited.
Data Availability: All relevant data are within the paper and its Supporting information files.
Funding: The author(s) received no specific funding for this work.
Competing interests: The authors have declared that no competing interests exist.
Introduction
Research in nanotechnology has evolved rapidly over the past few decades. Nanotechnology is a branch of science that deals with the atomic and molecular analysis of material objects [ 1 ]. Nanoparticles (NPs) are classified as particles up to 100 nm in size and consisting of chemical and physical processes, including unique properties [ 2 ]. They have a very significant role in various applications because of their unique properties such as optical, electrical, catalytic, electromagnetic, and mechanical, which enable them to be used in various sectors such as medical diagnostics, therapy, electronics, clothing, and agriculture [ 3 ]. Nano research is having an impact on a variety of fields, including the environment and catalysts [ 4 – 7 ]. Metal NPs production has been given great attention in the last few years, particularly to control and discover their possible applications and specific properties. The increased production of metal NPs has also increased their discharge into our environment, and the effect of their particular physical and chemical properties on the ecosystem is becoming a key concern [ 8 ]. NPs can have both negative and positive effects on the growth and development of a plant, and their influence on plants depends on the size, shape, and properties of both plant species and NPs [ 9 ].
Copper (Cu), an element in block D of the periodic table, is a microelement essential for plant improvement and growth. It is a cofactor of superoxide phenol oxidases, ascorbate oxidase, a part of regulatory proteins, and is involved in the electron transport chain during photosynthesis and respiration [ 10 , 11 ]. Cu in the form of nanomaterial gains exceptional properties like its small size and high surface area, which give it chemical reactivity, physical resistance, magnetism, and optical effects [ 12 ]. Due to these unique properties, copper nanoparticles (CuNPs) are used for a wide variety of applications, including bioactive coatings, air and liquid filtration, sensors, ceramics, films, skin products, lubricant oils, inks, wood protection, and textiles. Recent estimates suggest that the global production of CuNPs, or Cu-based NPs, has come to 200 tons/year [ 13 ]. Their extensive engineering and use have led them to enter the environment and interact with agriculture. They can cause either positive or negative effects following contact with plants, depending upon the concentration of the NPs and the type of plant. It is therefore the need of the hour to investigate the effect of CuNPs on crops, as there are only a few studies available that show the influence of CuNPs on crop plants.
CuNPs can be engineered by way of specific routes like physical, chemical, and biological techniques [ 14 – 16 ]. The main problems with the physical and chemical methods are the time-consuming nature of them and their usage of exclusive and toxic chemicals [ 17 ]. The chemical methods are eco-incompatible, costly, and also have a low yield. In contrast to physical and chemical methods, biological methods (green synthesis) are more advantageous in the sense of being eco-friendly, cost-effective, and high yielding [ 18 ]. Green synthesis is largely motivated by environmental concerns, with the goal of developing a green pathway for NPs synthesis that is also contamination-free [ 19 ].
Green synthesis involves the use of algae [ 20 ], sea cucumbers [ 6 ], marine animals [ 21 ], plants [ 16 , 22 , 23 ], and microorganisms among many others [ 24 , 25 ]. Biological techniques make it easier to reduce dissolved metal ions to a zero-valence state and produce the corresponding nanoparticles because of their inherent capabilities. NPs synthesis with the aid of using plants is beneficial over the use of microorganisms because it gets rid of the complicated procedure of keeping cellular cultures and can also be well scaled up [ 26 ]. Keeping in mind the importance and benefits of green synthesis, CuNPs were synthesized in this study by using a plant extract and their effect was evaluated on the growth and development of wheat ( T . aestivum ), an essential and widely consumed staple food. In Pakistan, wheat crops provide a living for 80 percent of farmers. It is also a very significant single commodity in Pakistan’s rural areas as a source of earnings [ 27 ]. Although some studies have reported the effect of CuNPs on wheat, they have used either very small concentrations (0.2, 0.4, 0.6, 0.8, and 1.0 ppm) of CuNPs [ 28 ] or chemically engineered CuNPs (50 nm) of large size [ 29 ]. In contrast to these, we used biologically synthesized CuNPs of 23 nm in size at a concentration of 0, 25, 50, and 100 mg/L.
Therefore, the first aim of this study is the green synthesis and characterization of CuNPs. In this study, an aqueous extract of Mentha longifolia will be used for the reduction of Cu ions to CuNPs at room temperature. The CuNPs will be characterized by UV-visible spectroscopy, scanning electron microscopy, X-ray diffraction, and Fourier transform infrared spectrometry. The second aim is the investigation of the effect of biosynthesized CuNPs on germination, growth, and biochemical attributes of the widely consumed staple food wheat.
Material and methods
Synthesis and characterization of cunps.
A biological method, i.e., the use of an aqueous extract of M . longifolia was adopted in this study for the synthesis of CuNPs. The plant material was collected from the Rawalakot region of Azad Jammu and Kashmir, Pakistan. The plant was identified with the help of the flora of Pakistan and other available literature, and a voucher specimen was submitted to the herbarium, Department of Botany, University of Poonch Rawalakot. To prepare the aqueous extract, the whole plant was dried in the shade, ground to powder, and 25 g of powder was dissolved in 400 mL of distilled water, then kept at room temperature for 24 hours. After that, it was filtered through Whatman filter paper no. 42 and the filtrate was used for the synthesis of CuNPs. The CuNPs synthesis was attained by treating 80 mL of 1 mM CuSO 4 at room temperature with 20 mL of plant extract, held in the dark, and color changes were observed. When the brown color developed (after 24 hrs), the reacting mixture was analyzed for UV-vis spectroscopy in the range of wavelengths between 300 to 800 nm using Perkin-Elmer lambda 750 spectrophotometers, indicating the formation of CuNPs. After initial indication, the reacting solution was centrifuged for 15 min at 10,000 rpm, the supernatant was discarded, the pellet was re-dispersed in distilled water and the centrifugation cycle was repeated at the same pace and duration. The final round of re-dispersion and centrifugation was accomplished with acetone to obtain cleaned and purified CuNPs. The morphology, phase, and capped functional groups of CuNPs were determined through scanning electron microscopy (MIRA 3 Tescan), x-ray diffraction analysis, and Fourier transform infrared spectrometry (Perkin Elmer Spectrum 100 FTIR).
Application of CuNPs on wheat using Petri plate assay
Seed source, treatments, and germination..
The seeds of four wheat varieties like 18-Elite Line 1, 18-Elite Line 3, 18-Elite Line 5, and 18-Elite Line 6 have been obtained from the Department of Plant Breeding Genetics, University of Poonch Rawalakot. The seeds were sterilized for 1 minute with 75 percent ethanol and 15 minutes with 2.5 percent calcium hypochlorite after being soaked in 0.6 percent HNO 3 for 10 minutes to end seed dormancy for successful germination [ 30 ]. The experiment was conducted in a completely randomized design (CRD) with 5 replicates. The four concentrations of CuNPs (0, 25, 50, and 100 mg/L) were applied in two ways, i.e., seed treatment and foliar spray. For seed treatment, the seeds were soaked in an aqueous solution of CuNPs for 3 h, and then 10 seeds of each variety were evenly placed in each Petri plate (labeled) already containing wet blotting paper. All the Petri plates were placed at room temperature and allowed to germinate with a daily supply of 5 mL of each concentration as a foliar spray. Samples were collected after 10 days of treatment and examined the effects of CuNPs on germination, growth, and biochemical attributes.
Germination indices measurements.
The germinated seeds were counted in each treatment for the measurement of germination percentage (GP). The GP was determined as GP = GN/SN × 100, where GN and SN are the total germinated seeds and tested seeds, respectively. The germination index (GI) was measured as GI = number of seeds germinated seeds/days of the first count + number of germinated seeds/days of the final count.
Growth parameters measurements.
To estimate growth, the primary root and shoot length of 10 days of seedlings were measured. For this, 5 plants were selected randomly from each treatment, the length was measured in cm, and the average was calculated. Similarly, the fresh weight of seedlings was measured in grams with the help of an analytical balance.
Biochemical contents measurements.
For the extraction of chlorophyll and carotenoid pigments, fresh leaves weighing 0.1 g were homogenized in 10 mL of 80% acetone. The homogenate was then centrifuged at 15000 rpm for 10 min, the pellet was discarded, and the absorbance of the supernatant was recorded at 666, 653, and 470 nm on the Shimadzu UV-1601 spectrophotometer. The formulae developed by [ 31 ] were used for the calculation of chlorophyll and carotenoid: Chla (mg/g) = [(12.25 x A663.2)—(2.79 x A646.8)] × ml acetone / mg leaf tissue, Chlb (mg/g) = [(21.50 x A646.8)—(5.10 x A663.2)] × ml acetone / mg leaf tissue. Total Chl = Chla + Chlb and Carotenoids (mg/g) = (1000 A470–1.8Chla– 85. 02 Chlb) /198. Total phenolic content was measured by Folin–Ciocalteu’s phenol reagent assay [ 32 ]. A 0.2 mL of plant extract mixed with 5 mL of 10-fold diluted Folin–Ciocalteu’s phenol reagent was kept for 4 minutes and then it was aided with 4 mL of sodium carbonate (7.5 percent w/v). The whole mixture was diluted up to a volume of 15 mL with distilled water and mixed well. The reaction was permitted to stand for 90 min, and the absorption of each sample was reported at 760 nm using a spectrophotometer Shimadzu UV-1601. The total phenols were measured using the calibration curve for gallic acid (GA) and presented as the equivalent of mg GA / g FW. To extract total soluble sugars, 50 mg of fresh leaves were crushed thoroughly in 5 mL of 80% ethanol and centrifuged for 10 minutes at 3000 rpm. From the supernatant, 0.1 mL was taken in a test tube and kept in a water bath for the evaporation of ethanol. After the ethanol had completely evaporated, 4 mL of 0.2 percent enthrone reagent was added to the test tube, heated in boiling water for 10 minutes, and then allowed to cool at room temperature. The absorption of each sample was reported at 760 nm using a spectrophotometer Shimadzu UV-1601. Total soluble sugars were measured using the calibration curve for glucose and presented as the equivalent of mg Gu/g FW.
Statistical analysis
The experiment was conducted in CRD using three replicates and analysis of variance (ANOVA) was performed in statistix 8.1. The statistical difference between means was evaluated, considering p < 0.05 as a significance level.
Results and discussion
Synthesis, morphology, size, and structure of cunps.
The CuNPs were synthesized from the aqueous extract of M . longifolia . The addition of aqueous extract to the aqueous solution of CuSO 4 resulted in the development of a color change from pale yellow to dark brown over the course of 24 h, the first indication of the formation of CuNPs in the solution ( Fig 1 ). This color change is developed due to the excitation of surface plasmon resonance (SPR) [ 33 ]. Previous research has also suggested that brown color can be established in the reaction mixture of plant extract and CuSO 4 [ 34 ]. This color change was further accomplished by taking a UV-vis spectrum of a brown-colored solution in the range of 400 to 800 nm ( Fig 1 ). The spectrophotometric study revealed an absorption band at 558 nm, a result of interaction between the free electrons presents on the surface of metal NPs and light of specific wavelengths. This absorption band indicates the formation of CuNPs in the colloidal solution because it is a known fact that CuNPs show characteristic absorption peaks in the range of 200–800 nm [ 35 ]. Moreover, it has been analyzed that a single SPR band represents round-shaped nanoparticles, whereas two or more SPR bands correspond to the anisotropy of nanoparticles [ 36 ]. In our study, CuNPs in the solution showed a single SPR band, which reveals the round shape of CuNPs. The morphology and size of the prepared CuNPs are shown in the images of SEM ( Fig 2a ) and their mean size distribution histogram ( Fig 2b ). The CuNPs exhibited a spherical shape, were distributed irregularly, and had an average size of 23 nm. Fig 2c represents the energy dispersive x-ray (EDX) of the powder and shows the presence of Cu in the material. The peaks belonging to elemental Cu, C, and O were clearly detected, and there were no extra peaks, demonstrating the purity of the synthesized NPs and correlating with the XRD analysis. It’s also possible that the existence of C and O is due to bioactive molecules that have been capped. Furthermore, only a few copper atoms on the surface of the NPs are likely to have been oxidized, yielding modest quantities of CuO and Cu 2 O. The XRD pattern of CuNPs is shown in Fig 3 , confirms the face-centered crystalline nature, as all the peaks match Cu with FCC symmetry and are consistent with JCPDS No: 04–0836, which reveals the crystalline nature of CuNPs [ 37 ]. With the help of FTIR ( Fig 3 ), the functional groups of all the possible biocompounds involved in the reduction and capping of CuNPs are identified. The FTIR spectrum showed peaks at 3754.36, 2054.64, 1937.67, 1587.34, 1266.30, and 1043.06 cm -1 . The peak at 3754.36 cm -1 corresponds to O-H stretching frequency of hydroxyl groups of polyphenols. The peaks at 2054.64 and 1937.67`cm -1 correspond to Cu-H (metal-hydrogen) bonds. The peaks at 1587.34, 1266.30, and 1043.06 cm -1 could be due to C-C stretching vibrations of an aromatic compound, C-O stretching of carboxylic acid, and ester bonds of phenolic compounds. These results suggest an interaction between the functional groups present in plant extract and Cu ions that results in bio-reduction, the formation, and stabilization of CuNPs [ 4 , 6 , 38 ]. CuNPs are thought to be formed from copper salts using plant extracts in three steps: production of copper ions, reduction of the ions, and lastly, oxidation of the reduced ions [ 16 , 20 ].
- PPT PowerPoint slide
- PNG larger image
- TIFF original image
a copper sulfate solution. b plant extract. c mixture of copper sulfate solution and plant extract at zero-time. d change in color of solution after 24 h of time lap. e UV-visible spectrograph of CuNPs.
https://doi.org/10.1371/journal.pone.0269987.g001
(a) FESEM micrograph of CuNPs, showing spherical shape of CuNPs; (b) Size distribution histogram of CuNPs, showing average size of 23 nm. c EDX spectrum of CuNPs.
https://doi.org/10.1371/journal.pone.0269987.g002
(a) XRD pattern of CuNPs, predicting crystalline nature of CuNPs; (b) FTIR spectrum of CuNPs, confirming functional groups from plant extract capping the CuNPs.
https://doi.org/10.1371/journal.pone.0269987.g003
Effect of CuNPs on seed germination of wheat
Seed germination is the beginning of a physiological process of a plant that needs water imbibition and is directly linked to the yield of the plant. In this study, seed germination and germination index of the tested wheat varieties exhibited an almost similar trend, except for the variety 18-Elite line-5. Fig 4 shows the GP and GI of wheat varieties after the 10 th day of treatment with different concentrations of an aqueous solution of CuNPs. When exposed to 50 mg CuNPs/L, GP and GI increased considerably in varieties 18-Elite line-1, 18-Elite line-3, and 18-Elite line-6, while the variety 18-Elite line-5 showed increased GP and GI under 25 mg CuNPs/L. After 50 mg CuNPs/L exposure, the GP increased to approximately 67, 31, 33, and 11 percent in 18-Elite line-1, 18-Elite line-3, 18-Elite line-5, and 18-Elite line-6, respectively, of the GP observed in control. However, in 18-Elite line-5, a higher increase was found at 25 mg CuNPs/L (100%) as compared to control. A similar trend was found for GI, it increased to approximately 76, 37, 41, and 17 percent in 18-Elite line-1, 18-Elite line-3, 18-Elite line-5, and 18-Elite line-6, respectively, of the GI, recorded in control after the application of 50 mg CuNPs/L. More than double the increase in GI was apparent in 18-Elite line-5 after treatment with 25 mg CuNPs/L. When varieties were exposed to 100 mg CuNPs/L, no statistical difference was found in GP compared to control, except in variety 18-Elite line-6, which showed a reduced GP and GI as compared to control. Similar results were reported by [ 39 ], where lettuce seeds showed better germination when treated with CuNPs. It is found that NPs tend to come into contact with seed coats, penetrate the seeds, and improve seed germination and seedling growth of the plants [ 40 ]. They found that CuNPs penetrated the cell wall and formed new pores, so water absorption was improved, and as a result, favorable seed germination was started. Another study demonstrated that high seed germination rates might be due to the photo-generation of active oxygen like hydroxide anions and superoxide, which causes re-activation of aged seeds. In the nanocomposite, activated carbon provides moisture and a large surface area for seed germination [ 41 ].
Different letters in the same sub-group of columns indicate significant difference at p < 0.05 level.
https://doi.org/10.1371/journal.pone.0269987.g004
Effect of CuNPs on seedling growth of wheat
RL, SL, and FW were measured for all the tested varieties after 10 days of seedling ( Fig 5 ) to investigate the effect of CuNPs on seedling growth of wheat. All the concentrations of CuNPs posed a positive effect on the RL of all wheat varieties, but the most prominent increase was found at 50 mg CuNPs/L in 18-Elite line-1, 18-Elite line -3, and 18-Elite line-6 and 25 mg CuNPs/L in 18-Elite line-5. When treated with 50 mg CuNPs/L, the RL increased by 50, 153, and 33% in 18-Elite line-1, 18-Elite line-3, and 18-Elite line-6, respectively, and by 134 percent in 18-Elite line-5 when treated with 25 mg CuNPs/L. When compared to the control, the SL in 18-Elite line-1, 18-Elite line-3, and 18-Elite line-6 increased by 19, 20, and 37 percent, respectively, under 50 mg CuNPs/L, and by 54 percent in 18-Elite line-5 under 25 mg CuNPs/L. The FW also displayed the same results as for RL and SL, where a CuNPs concentration of 50 mg/L exhibited the most prominent effect on the FW of three wheat varieties. The maximum increase of 75, 95, and 35 in FW was observed for 18-Elite line-1, 18-Elite line -3, and 18-Elite line-6, respectively, treated with 50 mg CuNPs/L, while a maximum increase of 18% in FW was observed for 18-Elite line-5, treated with 25 mg CuNPs/L.
https://doi.org/10.1371/journal.pone.0269987.g005
After 10 days of exposure, the CuNPs treatments modified the RL, SL, and FW of wheat. RL, SL, and FW were significantly increased, especially for varieties exposed to 50 mg CuNPs/L. These changes in the root and shoot system might be due to hormonal imbalances, like auxin and cytokinin imbalances [ 42 ]. Our findings are supported by the study [ 33 ], where CuNPs, at low concentration, increased the fresh weight of wheat. Moreover, we have observed that at 100 mg/L treatment of CuNPs, the seedling growth is significantly reduced compared to the treatment of 50 mg CuNPs/L, suggesting that a high dose of CuNPs can be lethal to plants. At high concentrations, CuNPs may release cupric ions that change the physiological processes of the plant. These modifications increase the capacity of particles that cross the cell membrane of a plant cell [ 3 ]. Previous studies also supported this fact that a higher concentration of CuNPs produces toxic effects, e.g., in chickpea and soybean, CuONPs increased root and shoot growth at 100 ppm, but beyond this concentration (200 ppm), the root and shoot growth was found to decrease [ 43 ]. Other studies also suggest the same result, e.g., CuNPs reduced the seedling length by 65 and 75% in Phaseolus radiatus and Triticum aestivum , respectively [ 44 ]. In a similar way, CuONPs decreased the RL in Cucurbita pepo when applied at a 1000 mg/L concentration [ 45 ].
Effect of CuNPs on photosynthetic pigments of wheat seedling
The effect of CuNPs on photosynthetic pigments of wheat varieties was measured in terms of total chlorophyll and carotenoid ( Fig 6 ). Chlorophylls are located in the chloroplast where they play a crucial role in the photosynthesis system, which is highly related to plant productivity and biomass [ 46 ]. As a result, the total content of Chl and carotenoid in wheat varieties was measured in all treatments in the current study. The total chl content started increasing upon exposure to CuNPs and a higher level was found at 50 mg CuNPs/L in 18-Elite line-1, 18-Elite line -3, and 18-Elite line-6, while in 18-Elite line-5, 25 mg CuNPs/L evidenced stimulation of chl up to 48% as compared with no application of CuNPs (control). Carotenoid content in test plants also followed the same trend as total Chl. A maximum increase of 61, 40, and 42% in carotenoid content was found in 18-Elite line-1, 18-Elite line -3, and 18-Elite line-6, respectively, at 50 mg CuNPs/L. Some authors also noticed changes in the content of photosynthetic pigments as a result of nanoparticle application. For example, a concentration-dependent effect of CuNPs on chlorophyll and carotenoid pigments was studied by [ 47 ], where CuNPs increased the chlorophyll and carotenoid content. Similarly, Vigna radiata has shown increased chlorophyll and carotenoid content under the treatment of CuNPs [ 48 ]. We also observed a significant inhibitory effect at higher concentrations (100 mg CuNPs/L). This could be attributed directly to oxidative stress or the interaction of RuBP carboxylase, due to copper interaction with SH groups. Additionally, the Chl content decrease might also be due to the lowered shoot biomass upon contact with higher concentrations of CuONPs or to the membrane damage as a result of excess lipid peroxidation of chloroplast membranes under oxidative stress [ 49 ].
https://doi.org/10.1371/journal.pone.0269987.g006
Effect of CuNPs on phenolic and sugar content of wheat seedling
Treatment with CuNPs significantly influenced the content of phenol and soluble sugar in the seedlings of wheat ( Fig 7 ). A maximum increase in total phenols was found at 100 mg CuNPs/L. It has been identified that plants induce the production of phenolic compounds in response to NPs [ 50 ]. In some other studies, an increase in phenolic content was also observed under a high concentration of nanoparticles. For example, fruits of Jalapeno pepper showed an increase in the total phenols under the application of CuNPs + Chitosan-PVA [ 51 ]. Similarly, the application of zinc nano fertilizer also increased the total phenols in pomegranate fruits [ 52 ]. This increase in phenolic content at higher concentrations may be related to the plant’s response to stress, as we observed 100 mg CuNPs/L caused toxic effects on wheat. Phenols are antioxidant compounds that trigger the synthesis of a series of secondary metabolites from the shikimic acid pathway or through phenylpropanoids under conditions of abiotic stress [ 53 ]. Therefore, the observed response may be related to ROS formation due to CuNPs. However, unlike the phenolic content, the soluble sugar content was found to be higher at 50 mg CuNPs/L treatment, as was the case with all other factors investigated. Overall, we found a positive effect of CuNPs on the growth and development of wheat. These results were supported by previous studies that showed Cu plays an important role in plant growth and development, and plant productivity [ 54 ].
https://doi.org/10.1371/journal.pone.0269987.g007
Conclusions
This study used an eco-friendly and appropriate method for the synthesis of CuNPs using Mentha longifolia extract . There were no chemical reagents or surfactant templates required, allowing green bioprocesses for a range of biomedical applications. During the application of CuNPs on wheat, we found in our study that with an increase in the concentration of CuNPs, the seed germination and growth of wheat plants were also increased. However, after a certain concentration (50 mg CuNPs/L), the seed germination and seedling growth were found to decrease. Overall results showed that application of CuNPs influences the seed germination and seedling growth of wheat at different concentrations. The higher germination and growth were found at 50 mg CuNPs/L treatment for 18-Elite line-1, 18-Elite line -3, and 18-Elite line-6 wheat varieties and at 25 mg CuNPs/L for 18-Elite line-5. Beyond this treatment, germination and growth were inhibited. Effective germination and growth at a certain optimum concentration and decreased germination and growth beyond this concentration may be attributed to the varieties of wheat and the uptake and accumulation of CuNPs by the roots, because the germination and growth were dependent on the exposure concentration of CuNPs. In particular, the contact of plants with NPs and the impact of such contact on plant growth could spur new research focus on nanobiotechnology. Further studies are needed to understand the mechanism of accumulation and uptake of CuNPs in plants and the way they act during seed germination and growth.
Supporting information
https://doi.org/10.1371/journal.pone.0269987.s001
Acknowledgments
We are thankful to Department of Plant Breeding and Genetics, University of Poonch Rawalakot for providing seeds of wheat varieties. We are also thankful to IST Islamabad for providing scanning electron microscopy and X-ray diffraction facilities.
- View Article
- PubMed/NCBI
- Google Scholar
- 26. Kim BS, Song JY. Biological synthesis of metal nanoparticles. In: Hou CT, Shaw JF, editors. Biocatalysis and Agricultural Biotechnology. CRC Press, Boca Raton; 2009. pp. 399–407.
- 49. Halliwell B, Gutteridge JMC. Free radicals in biology and medicine. Clarendon Press, Oxford; 1989.

Maintenance work is planned for Wednesday 1st May 2024 from 9:00am to 11:00am (BST).
During this time, the performance of our website may be affected - searches may run slowly and some pages may be temporarily unavailable. If this happens, please try refreshing your web browser or try waiting two to three minutes before trying again.
We apologise for any inconvenience this might cause and thank you for your patience.
RSC Advances
Green synthesis of copper nanoparticles and conducting nanobiocomposites using plant and animal sources †.
* Corresponding authors
a Advanced Materials Laboratory, Centre for Leather Apparel & Accessories Development, Central Leather Research Institute (Council of Scientific and Industrial Research), Adyar, Chennai 600020, India E-mail: [email protected]
We report large-scale biosynthesis of copper nanoparticles using an extract of henna leaves as reductant. Due to the substantial electrical conductivity of the calcined copper nanoparticles, we used them to prepare conductive nanobiocomposites utilizing collagen waste. We demonstrate that the nanobiocomposites, when inserted between batteries, illuminate a light emitting diode lamp.
Supplementary files
- Supplementary information PDF (966K)
Article information
Download citation, permissions.

Green synthesis of copper nanoparticles and conducting nanobiocomposites using plant and animal sources
K. Cheirmadurai, S. Biswas, R. Murali and P. Thanikaivelan, RSC Adv. , 2014, 4 , 19507 DOI: 10.1039/C4RA01414F
To request permission to reproduce material from this article, please go to the Copyright Clearance Center request page .
If you are an author contributing to an RSC publication, you do not need to request permission provided correct acknowledgement is given.
If you are the author of this article, you do not need to request permission to reproduce figures and diagrams provided correct acknowledgement is given. If you want to reproduce the whole article in a third-party publication (excluding your thesis/dissertation for which permission is not required) please go to the Copyright Clearance Center request page .
Read more about how to correctly acknowledge RSC content .
Social activity
Search articles by author, advertisements.

COMMENTS
Green synthesis of copper nanoparticles. The copper nanoparticles were synthesized using the method described by Ullah, N. et al., 2020 with minor modifications show in Fig. 1 [15]. In brief, the copper nanoparticles were created by dissolving 5 g of CuSO4·.5H2O in 50 mL of distilled water. A 250-mL beaker was filled with an equal volume of ...
The green synthesis of copper nanoparticles (CuNPs) using a leaf extract from Jatropha curcas (JC) has been documented in our present research work. The existence of flavonoids, tannins, glycosides, and alkaloids was confirmed by the phytochemical analysis of the plant extract and these chemicals can be used as reducing, stabilizing and capping agents.
Green synthesis of copper nanoparticles was initially confirmed by the position of SPR band at 340 nm in UV-Vis spectra. The particle size of about 20 nm with a spherical shape. XRD spectrum shows crystalline nanostructured copper particles and AFM techniques illustrate the spherical shape of nanoparticles with aggregation. TEM image shows poly ...
The synthesis of copper nanoparticles is a difficult task for a researcher to find the best valuable points, such as pH, temperature, concentration ratio and time. Time plays an important role in the synthesis of nanoparticles. In some cases, a color change occurs within 30 min, while sometimes it takes up to 48 h.
The use of biomaterials in the synthesis of nanoparticles is one of the most up-to-date focuses in modern nanotechnologies and nanosciences. More and more research on green methods of producing metal oxide nanoparticles (NP) is taking place, with the goal to overcome the possible dangers of toxic chemicals for a safe and innocuous environment. In this study, we synthesized copper nanoparticles ...
2. Green synthesis of nanoparticles. Green synthesis can be defined as the derivation of materials from green or eco-friendly resources by the use of solvent, good reducing agent, and harmless material for stabilization (Citation 37).Additionally, this synthesis route is straightforward, cost-effective, dependable, sustainable, and relatively repeatable, and results in more stable compounds.
Green synthesis is largely motivated by environmental concerns, with the goal of developing a green pathway for NPs synthesis that is also contamination-free . Green synthesis involves the use of algae , sea cucumbers , marine animals , plants [16, 22, 23], and microorganisms among many others [24, 25]. Biological techniques make it easier to ...
1. Introduction. Nanoparticles have attracted many researchers because of their unique properties compared to their bulk material [1, 2].They have unique characteristics due to the size, shape, distribution, and morphology [1, 3].One of the applicable nanoparticles in several fields is copper nanoparticles (CuNPs).
This is why its use as a stabilizing, reducing, and capping agent in the synthesis of copper nanoparticles (CuNPs) represents an option viable in the short term. ... Green Synthesis of Copper Nanoparticles. The Sargassum Extract (SE) was prepared with 10 g of sargassum dust in 100 mL of deionized water; the solution was warmed until it reached ...
Green synthesis of copper oxide nanoparticles using parthenium aqueous extract. Synthesis of copper oxide nanoparticles utilized copper (II) sulphate as a metal precursor. 0.01 M CuSO 4
Characterization of silver nanoparticles by green synthesis method using Pedalium murex leaf extract and their antibacterial activity. Appl Nanosci 6, 399-408 Ansilin S., J. Kavya Nair, C. Aswathy, V. Rama, J. Peter & J. Jeyachynthaya Persis. (2016). Green Synthesis and Characterisation of Copper Oxide Nanoparticles using
It is also known that the dietary allowance of copper (Cu) is 0.9 mg/day and a 70 kg healthy human body contains 110 mg Cu. The union of nanoscience and nanotechnology with physics, chemistry, and biology has emerged as a foremost approach for the synthesis and biomedical application of Cu and Cu-based NPs.
In recent ages, green nanotechnology has gained attraction in the synthesis of metallic nanoparticles due to their cost-effectiveness, simple preparation steps, and environmentally-friendly. In ...
green was observed, indicating the fo rmation of. copper nanoparticles, (CuNPs). This colour. change is as a result of interaction between. conduction electrons of metal NPs and incident. photons ...
This study employed green chemistry to synthesize copper nanoparticles (CuNPs) using fresh root extract of <i>Withania somnifera</i>. The size, shape, morphology, and stability of resultant CuNPs were investigated by UV-Vis spectroscopy, FTIR, transmission electron microscopy (TEM), and energy-dispersive spectrophotometer. Crude extracts were assessed phytochemically to determine the presence ...
Indigenous medicinal plant of Ethiopia has been applied for the first time to investigate the synergistic influence of phytoconstituents in green copper nanoparticles (g-Cu NPs) towards the enhancement of antimicrobial properties of NPs. We report the green synthesis of Cu NPs using <i>Hagenia abyssinica (Brace) JF. Gmel.</i> leaf extract. The synthesized g-Cu NPs were characterized by UV ...
Green synthesis of copper nanoparticles. Fruits of C. spinosa were collected from rural areas in western Iran and then were extracted by percolation procedure by means of methanol (80%) for 3 days at room temperature. 12 In the next step, the green synthesis of CuNPs was performed according to a method described elsewhere. Briefly, 75 mL of the ...
Today, different types of nanoparticles (NPs) are being synthesized and used for medical and agricultural applications. In this study, copper nanoparticles (CuNPs) were synthesized using the aqueous extract of mint (Mentha longifolia L.). For the characterization of CuNPs, UV-visible spectroscopy, scanning electron microscopy, X-ray diffraction, and Fourier transform infrared spectrometry were ...
We report large-scale biosynthesis of copper nanoparticles using an extract of henna leaves as reductant. Due to the substantial electrical conductivity of the calcined copper nanoparticles, we used them to prepare conductive nanobiocomposites utilizing collagen waste. We demonstrate that the nanobiocomposites, whe