
An official website of the United States government
The .gov means it’s official. Federal government websites often end in .gov or .mil. Before sharing sensitive information, make sure you’re on a federal government site.
The site is secure. The https:// ensures that you are connecting to the official website and that any information you provide is encrypted and transmitted securely.
- Publications
- Account settings
Preview improvements coming to the PMC website in October 2024. Learn More or Try it out now .
- Advanced Search
- Journal List
- Materials (Basel)


Recent Advancements in Plastic Packaging Recycling: A Mini-Review
Valentina beghetto.
1 Department of Molecular Sciences and Nanosystems, University Ca’Foscari of Venice, Via Torino 155, 30172 Mestre, Italy; [email protected] (R.S.); [email protected] (C.B.); ti.evinu.duts@900078 (M.A.-A.); [email protected] (M.F.)
2 Crossing S.r.l., Viale della Repubblica 193/b, 31100 Treviso, Italy
Roberto Sole
Chiara buranello, marco al-abkal, manuela facchin, associated data.
Not applicable.
Today, the scientific community is facing crucial challenges in delivering a healthier world for future generations. Among these, the quest for circular and sustainable approaches for plastic recycling is one of the most demanding for several reasons. Indeed, the massive use of plastic materials over the last century has generated large amounts of long-lasting waste, which, for much time, has not been object of adequate recovery and disposal politics. Most of this waste is generated by packaging materials. Nevertheless, in the last decade, a new trend imposed by environmental concerns brought this topic under the magnifying glass, as testified by the increasing number of related publications. Several methods have been proposed for the recycling of polymeric plastic materials based on chemical or mechanical methods. A panorama of the most promising studies related to the recycling of polyethylene (PE), polypropylene (PP), polyethylene terephthalate (PET), and polystyrene (PS) is given within this review.
1. Introduction
In recent years, the health of our planet has become a problem of crucial importance, with plastic recovery and disposal being of primary relevance [ 1 ].
Since the introduction of Bakelite in 1907 by Leo H. Baekeland, the first fully synthetic polymer, the plastic industry has evolved to revolutionize the way we live [ 2 , 3 , 4 , 5 ].
Polymers and plastic products own their well-known ubiquity and massive use to their excellent chemical–physical properties, which guarantee light weight, low price, and endurance [ 6 ]. Thanks to their great versatility, plastics are among the most used materials and find applications in many industrial sectors such as packaging, automotive vehicles, construction, and electronic devices [ 1 , 7 , 8 ]. Worldwide, over 360 Mt of fossil-based polymers are produced yearly, with an annual growth rate of 8.4%, two times higher than world global gross growth rate of production over the same period [ 5 ] ( Figure 1 a). The European plastic converter demand in 2018 reached 51.2 Mt, mainly to produce polyethylene (PE), polypropylene (PP), polyvinylchloride (PVC), polyethylene terephthalate (PET), and polystyrene (PS) ( Figure 1 b). These are mainly employed for packaging (39.9%), construction (19.8%), automotive vehicles (9.9%), and electronic devices (6.2%) [ 9 ] ( Figure 1 c).

( a ) World polymer production in metric tons; ( b ) distribution of main polymers produced; ( c ) 2018 European plastic converter demand and use.
A gradual switch to biobased plastics has been witnessed by the increasing use at an industrial level of alternative raw materials [ 10 , 11 ] such as polylactic acid (PLA) [ 12 ], polybutyl succinate (PBS) [ 13 , 14 ], polyhydroxyalkanoate (PHA) [ 15 , 16 , 17 ], and polyethylene furanoate (PEF) [ 18 , 19 , 20 ], together with different composite materials produced from starch [ 21 , 22 , 23 , 24 ], CMC [ 25 , 26 , 27 , 28 , 29 , 30 ], wood [ 31 , 32 ], lignin [ 33 , 34 ], and many different agro-industrial wastes [ 35 , 36 , 37 ].
Nevertheless, 99% of plastics produced today are fossil-based polymers, and they will continue to play an important role in many manufacturing compartments for a long time. In fact, according to the 2020 European Bioplastics report, the EU total production capacity of biopolymers is expected to reach 2.45 Mt by 2024 ( Figure 2 ), which is far lower than the plastic market needs [ 38 ].

Projection of world global production capacity of bioplastics by 2024.
The large gap between market demand and biobased plastics available today clearly shows the complexity of the problem and that all alternatives to approach the problem of plastic use and recycling must be pursued to reduce the environmental impact of polymers and plastic waste. In a recent article by Mendes and coworkers, the benefits of the use of bioplastics for the packaging industry were analyzed with the intent of delivering a guide for the design of more sustainable packaging to food packaging designers and producers [ 39 ]. The authors concluded that, from a climate point, the use of biobased plastics contributes to the generation of more sustainable food packaging compared to fossil-based ones; however, on the other hand, the relevance of some environmental problems originating from biobased plastics, such as eutrophication, use of water and pesticides, and effects on biodiversity, significantly reduces their environmental benefits.
Additionally, fossil-based plastics are generally scantly biodegradable and accumulate in the environment, posing serious waste management problems. Over the last 65 years, approximately 8300 Mt of fossil-based polymers were produced, 4900 Mt of which were landfilled, incinerated, or dispersed in the environment [ 5 , 40 ]. Thus, oceans, animals, and humans are inevitably exposed to different sources of contamination from plastic waste [ 41 , 42 , 43 , 44 , 45 , 46 ]. Climate changes, environmental modifications, and health pandemics are becoming more and more frequent, showing that humanity will have to rethink its unsustainable growth [ 47 , 48 ] by adopting a circular economy approach to resource consumption through eco-design, recovery, and recycling of polymeric materials with an integrated approach [ 49 , 50 , 51 , 52 , 53 ]. Circular economy is pushing toward a radical change in production and waste management to reduce water, waste, and energy consumption and to achieve zero-waste manufacturing cycles [ 10 , 54 , 55 , 56 , 57 ]. In this frame, European countries have developed different waste management systems and recycling techniques [ 58 , 59 , 60 , 61 , 62 , 63 , 64 ]. Nevertheless, a great part of post-consumer managed plastic is currently sent to incineration or landfill, while mismanaged waste is either discarded into the environment or is inadequately disposed of, potentially ending up in the ocean [ 46 ]. From 2006 to 2018, the amount of recycled post-consumer plastic waste doubled, reaching 32.5% (29.1 Mt), while 42.6% was used for energy production and 24.9% was landfilled [ 9 ] ( Figure 3 ).

Reuse of recovered post-consumer plastic waste.
In 2018, 5 Mt of plastic waste was recycled in Europe, 80% of which re-entered the EU as secondary materials, while the remaining 20% was exported outside the EU. The main industrial uses of recycled plastics in the EU are building and construction (46%), packaging (24%), agricultural applications (13%), and others (17%) [ 9 ].
Plastics may be subdivided into three categories: plastics in use, managed post-consumer plastic waste, and mismanaged plastic waste [ 65 , 66 ]. Managed plastic waste is generally disposed of by recycling, although a substantial gap exists between the quantity of plastic produced each year and the quantity of plastic thrown away since, depending on the type of product, there will be different storage and use times. Packaging products end their lifecycle generally in less than 1 year, while materials used for the construction and transport industry may last much longer. This means that the amount of waste produced each year is less than the amount of plastic in use. In 2015, 407 Mt of primary plastic entered the use phase, while only 307 Mt exited the use phase, with a consequent increase of 100 Mt of plastic in use [ 5 ].
According to the literature, it was estimated that, in 2010, between 4.8 and 12.7 Mt of plastics were leached into the ocean, predicting that, with inadequate waste management strategies, these numbers will increase by an order of magnitude by 2025 [ 46 , 67 ]. On this note, in January 2018, the European Commission issued the “European strategy for plastics in a circular economy” [ 68 ], including the ambitious target to make all plastics in EU recyclable by 2030. Soon after, in March 2018, China banned imports of plastic, generating a decrease in plastic waste export from EU of 39%, thereby overloading the EU waste management system and incinerators [ 65 , 69 ].
To reduce the amount of plastic waste disposed in landfills or incinerated, there are two main strategies: the use of biodegradable biobased plastics (as mentioned above) [ 38 , 70 ] and recycling [ 71 , 72 , 73 , 74 ]. It should be reaffirmed that not all biobased polymers are biodegradable, while some fossil-based ones are, as clearly reported in Figure 4 .

Examples of biobased and fossil-based polymers subdivided into biodegradable and not biodegradable.
Moreover, the recovery and recycling of biobased polymers is a relatively new issue and is still the object of studies compared to fossil-based polymers [ 39 , 75 ]; thus, different strategies will need to be put in place to implement the environmental sustainability of polymer manufacturing and recycling. According to the recent Circular Economy Package EU legislation and a paper by Briassoulis and coworkers, mechanical recycling is the best alternative for the valorization of both post-consumer fossil-based and biobased polymer waste, followed by chemical recycling [ 75 , 76 ].
The topic of sustainable manufacturing of plastics and packaging is so important that, from a research on Google Scholar using as key words “sustainable plastics”, “recycled plastic”, and “plastic recycling techniques”, a total of almost 95,000 papers were published between 2019 and 2021. This mini-review intends to give an outlook on different mechanical and chemical recycling techniques, giving a general panorama of the state of the art and recent innovative solutions by focusing mainly on papers published in the last 12 months relevant to plastic packaging. The scope of the work is to give a general overview of most recent technologies for the recycling of post-consumer packaging waste (PP, LDPE, HDPE, PET, and PS) to be used as secondary materials for the manufacturing of different materials. Since it is possible that the EU will implement plastic recycling up to 100% by 2050, avoiding the use of virgin naphtha for its production, the use of plastic waste as a source of energy seems bound to assume a minor importance in the future, while recycling of polymers to produce high-value products will be of strategic importance. For this reason, techniques to produce energy from plastic waste will not be discussed in this mini-review. The authors believe that a good understanding of the possible alternatives to plastic recycling and valorization, together with the difficulties encountered in sorting and reprocessing of post-consumer plastic waste, should help the industry, as well as end users, to adopt more responsible behavior and, consequently, promote the introduction of environmentally sustainable solutions.
2. Overview of Plastic Recycling Techniques
The word recycling refers to a set of modifications and transformations (mechanical treatment, chemical treatment, or heating) required to recover feedstock from a previously processed polymer which can be reused by the industry [ 73 , 77 , 78 ]. Plastic recycling methods available today are classified in primary to quaternary processes [ 79 , 80 ] ( Scheme 1 ).
Specifically, primary processes allow recovering and recycling pre-consumer or pure polymers which can be reused for the same scope. Secondary processes start from recovered post-consumer polymeric waste, which is sorted, trimmed, and re-extruded, giving a product with reduced physical–mechanical characteristics compared to the starting polymer, which in most cases cannot be reused for the same scope. Primary and secondary recycling represents physical processes that can be repeated several times. Tertiary processes adopt chemical recycling starting from polymers which may no longer undergo mechanical recycling, while quaternary ones are used for energy production. Polymers and plastics sent to landfill (end-of-life plastics) lose their value and become waste.
Different techniques adopted for plastic waste separation, processing, and possible reuse as secondary materials depend on the type of waste recovered. A first important distinction should be made between thermoplastic and thermoset polymers. Thermoplastics are usually processed by extrusion, as these polymers melt when heated and harden when cooled. A great advantage of thermoplastics is that the extrusion process can be repeated many times. The most used thermoplastics are PP, PET, LDPE, HDPE, PVC, and PS. Adversely, thermosets may not be reprocessed by extrusion since, when heated, an irreversible chemical reaction takes place. Main thermoset plastics are polyurethanes (PUR), resins (epoxy, phenol-formaldehyde, and polyester), and vulcanized rubber, widely used by the automotive and electronic industry. The most abundant polymers in post-consumer waste are polyolefins (PP, LDPE, HDPE, PET, and PS) used for packaging [ 58 , 81 , 82 ], with a consumption of over 23 Mt only in the EU in 2020.
3. Primary and Secondary Recycling
Mechanical recycling is the main and most widely used technology for plastic recycling, consisting of several steps, including collection, screening, automatic or manual sorting, washing, shredding, extrusion, and granulation [ 83 , 84 , 85 , 86 ] ( Scheme 2 ). Mechanical recycling is classified as primary or secondary according to the type of starting material being processed. Primary recycling gives the highest-quality recycled polymers and starts from closed-loop recycled products such as PET bottles or byproducts collected by manufacturing industries as pre-consumer well-separated material.
Secondary processes instead recover post-consumer plastics and, therefore, generate lower-quality polymers. It must nevertheless be considered that, from an economic standpoint, these processes have a reduced complexity and overall limited costs, generating significant income and reduced CO 2 production. According to the Ellen MacArthur foundation report, plastic production and incineration of plastic waste are estimated to produce over 400 Mt of CO 2 yearly [ 87 , 88 ]. Thus, recycled plastics can reduce fossil-fuel consumption and CO 2 emissions. According to estimates by Rahimi and coworkers [ 89 ], the adoption of plastic waste recycling worldwide would allow saving about 3.5 million barrels of oil each year.
Mechanical recycling generally includes four main steps: (i) screening and sorting; (ii) shredding; (iii) washing and drying; (iv) melting and reprocessing ( Scheme 2 ).
Screening and sorting of plastic waste is a fundamental step for the recyclability of the different plastics and the quality of the final polymer. This step is challenging, considering that the separation of mixed plastic waste often involves the combined use of different technologies [ 90 , 91 ].
To achieve an adequate separation of a specific polymer within a flow stream containing many different components (plastics, as well as metals, paper, organic residues, and dirt), characteristics of the final product must be accurately considered such as purity and destination. This will allow defining the best separation strategy to achieve high selection. Important properties commonly employed for plastic separation are magnetic or electric properties, particle size, density, and color. Relying on these properties, many different separation techniques have been developed such as dry or wet gravity separation, electronic or magnetic density separation, flotation, and sensor-based sorting together with auxiliary segregation techniques such as magnetic or eddy-current separation. These segregation methods are briefly described, mainly focusing on recently implemented technologies for PE, PP, PET, and PS recovery.
Gravity separation is a consolidated methodology that may be carried out in a dry environment (dry process) or in the presence of water (wet process) [ 63 , 92 ] ( Figure 5 a). Dry segregation techniques employ air classifiers or ballistic separators in which air is used as the medium to separate lighter materials from heavier ones. They can be positioned at the beginning of the process or at the end, to segregate end-of-life plastics from main plastic streams ( Figure 5 b). Wet gravity separation includes sink and float, jigging, and hydrocyclone techniques.

( a ) Different methodologies of gravity separation; ( b ) dry segregation; ( c ) sink and float separation; ( d ) hydrocycloning; ( e ) eddy current separator.
With sink and float separation, polymers are separated into two different streams depending on whether they have a higher or lower density than water. Materials such as PET, PVC, and PS will sink, while others such as PE, PP, and expanded polystyrene will float ( Figure 5 c). This type of separation guarantees an effective first separation, but it is not adequate to produce high-quality secondary materials and needs to be combined with other separation techniques [ 93 , 94 , 95 ].
Zhang and coworkers developed a pretreatment of PET via preliminary NaOH and ethanol hydrolysis to promote plastic flotation. Optimal conditions allowed the quantitative recovery of highly pure PET fractions [ 96 ].
Heidarpour and coworkers reported the influence of microwave irradiation in the presence of chemical additives such as PEG-400, methylcellulose, or tannic acid on the float–sink behavior of polyoxymethylene, polycarbonate, and polyvinyl alcohol. According to this study, microwave irradiation reduced the contact angle values of tested plastic surface in the presence of chemical additives (depressant) by implementing their sink–float separation capacity, thereby increasing their hydrophilicity [ 97 ]. The authors mention the possibility of using this technology for whichever plastic material.
Jigging is one of the oldest gravity separation techniques and is similar to dry gravity methods where, in most cases, water is used instead of air [ 90 , 94 ]. A water stream is pushed up and down by pistons, and plastics are separated mainly depending on their morphological and physical characteristics.
Hydrocycloning is based on centrifugal and centripetal forces together with the fluid resistance of different materials processed ( Figure 5 d). New trends in hydrocycloning separation focus especially on the recovery of precious metals from electronic device waste [ 98 , 99 ], and it seems to be a very valuable tool for more sustainable separation of plastic waste from metals.
The eddy current separator is made of a high-speed magnetic rotor which generates an electric current, the so-called eddy current, used to remove nonferrous metals (aluminum and copper) from waste plastic, glass, and paper, among others ( Figure 5 e) [ 100 ]. These separators are generally located at the beginning of the recycling process.
With a separator and drum screen, plastics are fed into a large rotating drum where materials are separated by size, thanks to holes in the drum, so that only smaller particles pass through and are separated from larger ones.
Different gravity segregation methods were analyzed by Nie and coworkers for the sustainable recovery and recycling of high-value metals from waste printed circuit board (WPCBs) [ 101 ]. This study analyzed the dynamics and statics of gravity concentration methods. The settling velocity of three kind of particles was studied, demonstrating that the stratification by density is spontaneous and can achieve the lowest potential energy. The concentration of differently sized metal particles could be effectively enriched, and the metal purity increased from 56.5% to 68.2% for decreasing particle size, albeit with a modest decrease in yield (from 86.41% to 83.04%). No recent papers were found for the use of innovative solutions for the recovery of PE, PP, PET, or PS by jigging, hydrocycloning, eddy current separation, and drum and different gravity segregation techniques, but they were reported to give a general overview of different separation technologies available.
Optical sensors are used for the characterization of plastic stream in a continuous manner where air jets allow for separation. Optical sensors may be subdivided in molecular spectroscopies and atomic spectroscopies [ 102 ], the prevalently used Raman spectroscopy (RS) [ 103 ], Fourier-transform infrared spectroscopy (FTIR) [ 96 ], near-infrared spectroscopy (NIRS) [ 104 ], and terahertz spectroscopy (THz) [ 105 ], and elemental spectroscopies such as laser-induced breakdown spectroscopy (LIBS) [ 106 ] and X-ray fluorescence spectroscopy (XRFS) [ 102 ].
Bobulski and coworkers implemented new portable devices for computer image recognition in combination with artificial intelligence for waste recognition and easy municipal waste separation. The devices were used both at home and in waste sorting plants, and they could be a very useful tool for an efficient and economically sustainable separation of plastic waste stream [ 107 ].
Most companies use a combination of different separation techniques to obtain sufficiently pure polymers from post-consumer plastic waste. The purity of the finished product depends on an adequate compromise between costs and benefits, and this leads to purities ≤95% which require further separation and purification steps. Sorting technologies reported above are generally inadequate for the separation of complex materials such as multilayered packaging or fiber-reinforced composites; therefore, these materials are generally incinerated for energy recovery or landfilled as end-of-life plastics.
Innovative recycling methods such as selective polymer dissolution were demonstrated to be efficient in extracting different polymers and fibers from multilayered films and composite materials [ 108 ]. In fact, Knappich and coworkers reported the efficient recovery and recyclability of epoxy and polyurethane resins from carbon fiber-reinforced plastics with different proprietary CreaSolv ® formulations at a laboratory scale.
Multi-material plastic waste separation technologies are also being developed to enable a proper sorting of composites, which will generate new value streams to recover and recycle plastics which are today incinerated or landfilled [ 109 ]. Many approaches have been tested, for example, for the separation of polyester from cotton fibers to recycle textile waste. Solvent-based technologies are an interesting solution, with the possibility of selecting specific solvents which may solubilize either cotton or polyesters [ 110 ]. A crucial aspect for industrial success and applicability is the nature of the solvent in terms of volatility, flammability, toxicity, and recyclability [ 111 ].
Once the mechanical separation is complete, the materials are shredded by passing them through a system of rotating blades. The obtained flakes are then sorted by size with a grid, washed and dried, made ready for reprocessing by extrusion or agglomeration, and sold.
Agglomeration is generally used to reprocess plastic films which are cut in small pieces, heated by friction and water-cooled. The agglomerates are usually combined into plastic flakes and pelletized by extrusion. Agglomeration is highly energy-consuming and, therefore, less widespread [ 90 ].
Extrusion remains the most widely used method for processing both virgin and recycled plastic. Plastic flakes are fed into the extruder and pushed by a screw into a heated cylinder, thus melting the plastic. At the end of the extruder, a pelletizer cools and cuts the final polymer into pellets.
Both shredding and extrusion may lead to partial degradation of the polymer due to chain scission and thermo-oxidative reactions, reducing the polymer chain length and, consequently, its mechanical properties [ 112 , 113 ]. Moreover, impurities deriving from other packaging components further contribute to the diminished physical–mechanical characteristics of reprocessed plastics [ 104 ].
A detailed study was published by Eriksen and coworkers on the thermal degradation, processability, and mechanical properties of re-extruded PET, PE, and PP from post-consumer waste. PET is well suited for closed-loop recycling to meet bottle and food-grade PET quality, although moisture control is a key requirement when reprocessing PET into products. For this polymer, degradation, which generally occurs during recycling by extrusion, may be avoided by careful decontamination. The quality of reprocessed PE samples from non-food bottles strongly depends on the presence of impurities from other polymers and from lids and labels. PE reprocessing by extrusion suggested that closed-loop recycling may be achieved with selected PE bags with low levels of polymer cross-contamination. Adversely, PP reprocessed by extrusion showed low mechanical properties with large variations in impact strength, reducing possible applications of reprocessed PP. Thus, the heterogeneity of PP waste, even if food packaging is managed separately, as well as polymer degradation during recycling, represents crucial limitations for PP waste recycling [ 114 ].
A possible remedy to downgrading due to extrusion was reported for the first time by Wang and coworkers. The authors reported a process to modify polyolefins from post-consumer plastic waste via a one-step radical grafting and cross-linking process, producing covalent adaptable networks or CANs [ 112 ]. This procedure relies on the functionalization of polyolefins with polar reagents, which modify the properties of the starting material, thus imparting new characteristics such as wettability, printability, and compatibility with other polymers. Upcycling of LDPE from plastic bags was achieved by free-radical reaction in a twin-screw extruder in the presence of maleic anhydride and butanediol. PE-CANs showed higher solvent resistance, tensile strength, and modulus compared to virgin PE due to the presence of cross-linking bonds generated during the extrusion process. Upcycling of post-consumer plastic waste by reactive extrusion is an interesting area of research which will surely receive much attention in the future; however, characteristics of CAN polymers must be acquired to define new possible manufacturing applications [ 115 ].
4. Chemical Depolymerization
In addition to mechanical methods, recycling can be performed via chemical depolymerization [ 111 , 116 ].
Chemical recycling has great potential in the circular economy of plastics; it can close the loop by producing starting monomers from the polymers that may be reprocessed to produce high-value-added chemicals [ 70 ]. It is estimated that, by 2050, almost 60% of plastic production can be based on recycled products [ 117 ]. Millions of euros are being invested to enhance chemical recycling and other cutting-edge technological solutions with the aim of producing 1.2 Mt of recycled plastic in EU by 2025 and 3.4 Mt by 2030 [ 9 ].
Chemical recycling methods are classified according to reaction conditions into solvolysis (hydrolysis, methanolysis, and glycolysis), catalytic depolymerization, and enzymatic depolymerization [ 83 , 84 , 118 , 119 , 120 , 121 , 122 , 123 , 124 , 125 , 126 , 127 ]. Only main innovative solutions devised in the last year for plastic packaging chemical recycling are analyzed below i.e., PE, PP, PET, and PS.
4.1. Solvolysis
Solvolysis involves the breaking of the hydrolyzable bonds of a polymer in the presence of an alcohol or water. It is rather frequent that, to improve reaction conditions, product selectivity, and yield, catalysts are used to promote solvolysis reactions [ 83 , 84 , 119 , 128 ].
4.1.1. Hydrolysis
Hydrolysis reactions perform better from an environmental point of view but require higher energy consumption compared to other solvolysis methods [ 129 ]. They may be carried out in neutral, acidic, or alkaline conditions.
Neutral hydrolysis of PET has long been known and is generally processed in the molten phase, at temperatures above 245 °C with a water/PET ( w / w ) ratio above 5.1/1. A further improvement in the rate of the reaction may be achieved via the addition of catalytic amounts of alkali metal acetates, organophosphorus compounds, or zeolites [ 128 ]. Recently, Colnik and coworkers reported hydrolytic recycling of colorless and colored PET bottles in sub- and supercritical water with temperatures between 250 and 400 °C, in 1 to 30 min. Highest yields in terephthalic acid (TPA) were achieved at 300 °C in 30 min with purities near to 100% [ 130 ] ( Scheme 3 ).
Interestingly, according to the work by Stanica-Ezeanu and coworkers, sea salt is an efficient neutral catalyst promoting PET degradation; by means of a mathematical model, it was estimated that, in tropical regions, only 72 years are necessary for spontaneous complete degradation of PET to occur [ 131 ].
Acid hydrolysis of PET proceeds by polymer dissolution in concentrated acids (H 2 SO 4 , H 3 PO 4 , and HNO 3 ) and heating, leading to chain fragmentation at high temperature.
These processes have not been, to the best of our knowledge, the object of recent studies, probably due to their low environmental sustainability; therefore, they are not further discussed in this review.
Alkali-promoted glycolysis of PET has been widely reported using both inorganic and organic bases [ 132 ]. Due to the high quantities of alkali required and consequent environmental impact of the process, in this case, no innovative solutions were found in recent publications.
4.1.2. Methanolysis
Methanol is widely used and is effective for the solvolysis of various polymers such as PET, polyamides, and polycarbonates. The majority of post-consumer recovered PET is currently reprocessed by mechanical recycling; however, this process leads to molar mass reduction and a consequent reduction in the physical–mechanical properties of the polymer, which is generally used to produce carpets (72%) [ 70 ], along with a small percentage of PET for bottle production [ 129 ]. Moreover, the commercial appeal of mechanical recycled PET depends on the price of oil; thus, when oil is available at prices below $65 per barrel, mechanically recycled PET is no longer competitive [ 70 ]. Chemical depolymerization to produce high-quality monomers and oligomers may be a solution to this problem.
The primary scope of PET chemical recycling is to regenerate TPA, dimethyl terephthalate (DMT), bis(2-hydroxyethyl) terephthalate (BHET), and ethylene glycol (EG) [ 133 ] or other chemical substances [ 134 , 135 ] ( Scheme 3 ).
Methanolysis of PET is generally a degradation process performed at high temperatures (180–280 °C) and pressures (2–4 MPa), and the major products are DMT and EG [ 70 , 129 ], with high capital and operating costs. Recently, Pham and coworkers [ 124 ] developed a low-energy catalyzed methanolysis to convert PET into DMT at room temperature in the presence of K 2 CO 3 as a catalyst. Despite the overall reaction time of 24 h, PET resins were completely decomposed into monomers with high selectivity in DMT with 93.1% yield at 25 °C. 2-Hydroxyethyl methyl terephthalate (HEMT) and monomethyl terephthalate (MMT) were the major byproducts collected after the reaction ( Scheme 4 ).
Myren and coworkers described a new method for methanolysis of post-consumer PET waste in the presence of NaOH carried out in a microwave or electrochemical reactors. Under mild reaction conditions (85 °C, 40 min) overall yields in TPA of 65% were achieved under microwave irradiation [ 136 ].
Barnard and coworkers published a review in 2021 evaluating advantages and disadvantages of chemical recycling of PET based on the energy economy coefficient and environmental energy impact. Different technologies evaluated comprised neutral, acidic, or alkaline hydrolysis, enzymatic hydrolysis, solvolysis, glycolysis, and aminolysis. From the comparison of data collected, alcoholysis was the most energetically expensive process; moreover, the low boiling point of alcohols generally requires high-pressure reactors. On the contrary, methanolysis carried out in the presence of a nanodispersion of ZnO was found to be the least energetically expensive process for PET degradation, giving high-quality DMT [ 129 , 137 ].
Additionally, Zhang and coworkers proposed a novel, simple and economic hydrophilic modification of PET by surface alcoholysis in the presence of ethanol and a sodium hydroxide water solution, which influenced the wettability of PET and promoted sink–float separation from hydrophobic PS, PVC, and PMMA [ 96 ].
Another very interesting example of the methanolysis of PET was achieved in the presence of an organocatalyst prepared from very simple reagents such as tetramethyl ammonium hydroxide and dimethyl carbonate, [NMe 4 ] + [OCO 2 Me] − , achieving good yields of DMT (≤75%) in mild reaction conditions (100 °C and 4 wt.% organocatalyst) [ 138 ]. Nevertheless, long reaction times (16 h), solvents, and product purification were necessary. Alternatively, imidazolium metal-based ionic liquids (ILs) can achieve a comparable or even better performance than [NMe 4 ] + [OCO 2 Me] − [ 139 ]. Main ILs reported in the literature are depicted in Figure 6 .

Main ILs reported in literature.
4.1.3. Glycolysis
Glycolysis was also verified to be a promising alternative with moderate energy and environmental impact [ 129 ]. Glycolysis produces the BHET monomer, which is a good starting material for PET upcycling. As reported by Lalhmangaihzuala and coworkers, glycolysis of post-consumer PET waste may be efficiently promoted by heterogenous catalysts prepared from orange peel ash. Total depolymerization of PET was detected within 90 min, producing BHET in 79% yield. The catalysts were recovered up to five times without significant deactivation. This study opens the way to a highly environmentally sustainable approach to post-consumer plastic waste recycling [ 127 ].
Organocatalyst-assisted glycolysis is considered a new frontier for a green approach to plastic recycling in comparison to conventional organometallic complexes [ 138 , 140 ]. Wang and coworkers [ 141 ] reported a very promising study on the glycolysis of PET using 1,3-dimethylimidazolium-2-carboxylate as an organocatalyst, achieving complete depolymerization in less than 1 h at 180 °C, with up to 60% yield in BHET recovered by precipitation from the reaction mixture upon cooling.
Alternatively, Fuentes and coworkers reported the glycolysis of PET bottles to BHET in the presence of catalytic amounts of different metal oxides (ZnO, CoO) obtained for the recycling of spent alkaline and lithium-ion batteries. Reactions were carried out in EG at approximately 200 °C for 2 h; in the best conditions, yields of the BHET reached 80% [ 126 ].
Functionalization of silica-coated, magnetic Fe 3 O 4 nanoparticles, with an iron-containing ionic liquid, was recently employed for the glycolysis of PET to BHET. The advantage of these catalysts is in their high recyclability and ease of recovery due to their magnetic properties, and no traces of metals were found in the final products [ 142 ].
4.1.4. Aminolysis
While aminolysis presents the best energy and environmental parameters, the use of ammonium-based ionic liquids makes the production process more expensive [ 129 , 143 , 144 ]. The high temperatures involved in aminolysis are compensated for by very low depolymerization times due to increased reaction speed. Adversely, depolymerization by aminolysis of PET produces terephthalamides which have limited industrial applications. Different amines such as monoethanolamine (MEA) have been used for the aminolysis of PET with and without catalysts such as metal salts, quaternary ammonium compounds, and ionic liquids [ 145 ] ( Scheme 5 ).
Catalyst-free, microwave-assisted aminolysis of PET proved to be an efficient method for the recovery of different terephthalamides starting from allylamine, ethanolamine, furfurylamine, or hexylamine with high selectively and yields. Terephthalamides were employed to produce good quality films [ 123 ]. Furthermore, aminolytic upcycling of PET post-consumer waste was achieved in the presence of different amino-alcohols in the presence of various organocatalysts to give diol terephthalamides, which were employed to produce poly(ester-amides) [ 146 ].
4.2. Catalytic Depolymerization
Plastic depolymerization may be carried out in the presence of different catalysts such as strong mineral acids, bases, organocatalysts, enzymes, and metal catalysts in homogeneous or heterogeneous phase [ 147 ].
4.2.1. Enzymatic Catalysis
To date, the enzymatic activity of various microbial and fungal species has been tested for the degradation of various polymers [ 148 , 149 ]. As with chemical degradation, the major difficulty in the enzyme degradation of polymers such as PE and PP derives from their high hydrophobicity, stability, and inertness, and their reactivity may be implemented by UV or thermal oxidation pretreatments [ 150 ]. While PE and PP enzymatic degradation is still a very challenging topic, numerous hydrolytic enzymes have been identified and are efficient for PET degradation [ 151 ]. PET hydrolases represent one of the most recent breakthroughs in the depolymerization of post-consumer PET, allowing the recovery of terephthalic acid and ethylene glycol at industrial relevant scale [ 120 ]. Interestingly, Sadler and coworkers developed an innovative enzyme-catalyzed post-consumer PET hydrolysis with engineered Escherichia coli to produce vanillin [ 134 ].
These new technologies once more highlight the importance of the development of specifically devised new microorganisms and enzymes for plastic depolymerization. In this connection, Santacruz Juarez and coworkers reported the use of molecular docking simulation to predict affinity, strength, and binding energy between two molecules to analyze the activity of laccase (Lac), manganese peroxidase (MnP), lignin peroxidase (LiP), and unspecific peroxygenase (UnP), thereby helping in the development of new enzymes [ 152 ]. Data achieved showed that synergic enzymatic combination, as it normally happens in nature, boosts the catalytic efficiency by promoting sequential degradation processes. The use of microorganisms and enzymes has been widely studied with the intent to find an environmentally sustainable solution to microplastic and nanoplastic contamination. Taghavi reviewed the state of the art of plastic packaging biodegradation by living microorganisms reporting mechanisms of action, advantages, limitations, and technology readiness levels (TRL). The focus of this very important research area is a reduction in plastic pollution in the environment more so than its recovery and reuse; thus, it is not further analyzed in this paper [ 148 ].
4.2.2. Hydrogenolysis
Hydrogenolysis is widely employed for the depolymerization of PET in the presence of hydrogen and homogeneous Milstein-type Ru–PNN complexes which are highly reactive toward the C=O double bonds of PET to give 4-benzenedimethanol (BDM) in 99% yield at 160 °C in 48 h ( Table 1 , entry 1), while they are ineffective in the presence of PP and PE [ 147 , 153 , 154 , 155 ]. More complex phosphine ligands have also been tested, but the economic viability on an industrial scale seems to be rather limited [ 147 ] ( Table 1 , entries 2–3).
Phosphine ligands of Milstein-type Ru–PNN complexes.
1 Selectivity to BDM. 2 Selectivity to BTX.
Two very important studies have been published on the efficient conversion of post-consumer PET to benzene, toluene, and xylenes by reportedly “unlocking hidden hydrogen in the ethylene glycol part” with Ru/Nb 2 O 5 catalyst [ 156 , 157 ]. The hydrogen is formed in situ during the reaction from ethylene glycol, and it appears that, in the presence of Ru/Nb 2 O 5 , two different pathways (decarboxylation and hydrogenolysis) compete to determine the selectivity toward alkyl-aromatic compounds ( Table 1 , entries 4–5) [ 156 ].
Solventless hydrolysis of PET bottles to TPA and ethylene has been selectively achieved by a carbon-supported single-site molybdenum-dioxo catalyst under 260 °C and 1 atmosphere of H 2 with 87% yield. The catalyst exhibits high stability and can be recycled many times without loss of activity [ 158 ].
Hydrogenolysis of PET to liquid alkanes has been carried out under mild reaction conditions using ruthenium nanoparticles supported on carbon (Ru/C). Under optimal reaction conditions (200 °C, 20 bar H 2 , 16 h), PE was converted into liquid n -alkanes with 45% yield [ 159 ]. Another SnPt/γ-Al 2 O 3 and Re 2 O 7 /γ-Al 2 O 3 heterogeneous catalyst was used to produce linear alkanes from HDPE. This type of catalyst promotes a tandem reaction via which poorly reactive aliphatic substrates are first activated through dehydrogenation and then functionalized or cleaved by a highly active olefin catalyst [ 160 ].
These technologies are particularly attractive from an industrial point of view as heterogeneous catalysts are generally easier to use and economically more sustainable than homogeneous ones.
4.2.3. Hydrosilylation
Hydrosilylation carried out in the presence of different silanes (tetramethyldisiloxane and polymethylhydrosiloxane) and borane or Ir catalysts has also been tested in the past for the depolymerization of PET, PS, and PVC [ 161 ]. Probably because of the high cost of reagents and Ir catalysts, combined with low yields in monomers recovered, no similar studies were published in the last 12 months. An interesting alternative was proposed by Fernandes and coworkers in 2020 for the depolymerization of PET by silanes and an air-stable, cost-effective dioxomolybdenum complex, MoO 2 Cl 2 (H 2 O) 2 . Although reaction conditions are rather harsh (160 °C, 4 days), very good yields in p -xylene were achieved for the reductive depolymerization of PET (65% yield) in the presence of 5 wt.% MoO 2 Cl 2 (H 2 O) 2 and six equivalents of phenylsilane. In another study, Fernandes described the first example of reductive hydrosilylation of PET and other plastic waste using an economically and environmentally sustainable Zn catalyst, Zn(OAc) 2 ·2H 2 O, to produce high-value-added compounds such as 1,2-propanediol, 1,6-hexanediol, tetrahydrofuran, and p -xylene. In the same reaction conditions, in the presence of Mo oxides, yields in p -xylene were equivalent while higher yields in EG were obtained (43%) [ 162 ]. Much work surely needs to be done to implement these technologies to industrial maturity, but the use of highly available, environmentally friendly catalysts is a great advantage and should be further pursued.
5. Thermal Recycling
Thermal recycling mainly comprises pyrolysis, hydrocracking, and gasification ( Scheme 6 ) [ 163 ]. Since there are no recent advancements for gasification, only pyrolysis and hydrocracking are reported. An outline of the main innovative solutions recently published is reported below.
5.1. Pyrolysis
Pyrolysis, or thermal cracking, is a process that occurs at high temperatures (500 °C) and in the absence of oxygen. Different kinds of catalysts can be used to improve the efficiency of the pyrolysis process since they target a specific reaction and reduce the process temperature and time [ 164 ]. Unlike other thermochemical conversion methods, pyrolysis leads to liquid or wax mixtures rich in hydrocarbons, an ideal raw material for a refinery [ 165 ]. Thermal pyrolysis is typically used for the recycling of those polymers for which depolymerization is harsh and that are not currently mechanically recyclable (PE/PP/PS mixtures, multilayer packaging, and reinforced fibers). Thanks to the high temperatures, it guarantees molecular bond breaking in the polymer chains to give, depending on the nature of the polymer, depolymerization or random fragmentation [ 122 , 166 ]. Alternatively, catalytic pyrolysis can be performed on the same polymers at lower temperatures by carbocation formation and subsequent isomerization [ 161 ]. Both thermal and catalytic pyrolysis approaches are not selective, but advantages rely on high conversions, thermal stability of the products and, in some cases, high-value enriched oil production. Pyrolysis, therefore, is an interesting recycling approach for a safe circular economy [ 161 , 166 ].
Pyrolysis must be preceded by pretreatment of the plastic waste, to ensure that it is not contaminated by non-plastic materials such as metal and wood. This step is necessary to ensure the economic feasibility of the plastic-to-fuel (PTF) plant, and it can usually be achieved by sorting, crushing, or sieving depending on the origin of the waste. Since pretreatment techniques are consolidated methodologies, no innovative methods were reported in the last year.
Another important aspect derives from different sources of plastic processed which may be different in shape and size, requiring to be uniformly sized as grains before feeding into the pyrolysis process. This step adds an extra cost to the process.
Depending on the type of reactor, the pre-sizing step can be skipped or modified. For example, rotary kilns can accommodate differently sized and shaped plastics; hence, the pre-sizing step can be avoided. Fluidized bed reactors, instead, need to have uniform thermodynamics in the reactor; therefore, plastic waste should be evenly sized. To cope with this challenge, several feeding devices have been tested [ 166 ].
Currently, the study of catalytic pyrolysis is very active, and a wide range of synthetic catalysts have been employed to enhance the overall pyrolysis process and to improve the quality of produced liquid oil.
Most PE pyrolysis approaches are promoted by heterogeneous acid catalysts (e.g., zeolites, alumina, and silica) and are usually unselective, resulting in a broad distribution of gas (C3 and C4 hydrocarbons), liquid (cycloparaffins, oligomers, and aromatics), and solid products (char, coke). This behavior is due to the radical mechanism of the C–C bond scission, leading to a complex mixture of olefinic and cross-linked compound [ 122 , 166 ].
A very recent novel study on this topic was carried out by Miandad and coworkers, in which the effect on yield and product quality of Saudi natural zeolite was investigated [ 164 ]. Saudi natural zeolite catalyst was improved via novel thermal activation (TA-NZ) at 550 °C and acid activation (AA-NZ) with HNO 3 . Pyrolysis feedstock was composed of single or mixed PS, PE, PP, and PET, in the presence of both modified natural zeolite (NZ) catalysts. The authors reported that PS produced the highest yield in liquid oil, i.e., 70% and 60% using the TA-NZ and AA-NZ catalysts, respectively, compared to PP (40% and 54%) and PE (40% and 42%).
In addition to zeolite, the research on catalytic pyrolysis has focused on other catalytic systems, always considering that the catalytic activity of the catalyst is derived from its Lewis acid sites. Most homogeneous catalysts for polyolefin degradation have been classical Lewis acids such as AlCl 3 . On the basis of these considerations, Su and coworkers [ 167 ] worked on AlCl 3 –NaCl eutectic salt as a catalyst, allowing a reduction in reaction temperature, an increase in reaction rate, a reduction in heavy oil components, and the inhibition of polyolefin formation.
Pyrolysis is most often adopted to convert plastic waste to fuels. An example of differentiation is the production of high-value-added carbon nanotubes (CNTs) [ 168 ] using a metallic Ni catalyst supported on different oxides and generated in situ. Selectivity, yield, and structural properties were tuned according to the degree of metal–support interaction in different catalysts.
5.2. Hydrocracking
Hydrocracking is a catalytic refining process for the selective recovery of useful chemical fractions in the range of heavy diesel to light naphtha. Hydrocracking requires a bifunctional catalyst with an acidic function, enhancing the cracking activity, typically provided by a high-surface-area support, such as a zeolite [ 169 ].
Recent studies have focused on the conversion of both post-consumer and laboratory polymers in mild conditions, using a metal–zeolite catalytic system.
Jumah and coworkers [ 170 ] treated low- and high-density polyethylene (LDPE, HDPE), polypropylene (PP), and polystyrene (PS) to produce liquid petrol gas (C3–C4) and naphtha. They reported the effect of both the catalyst morphology (beta zeolite impregnated with 1% Pt) and the feed stream variation, by reacting different polymers individually and post-consumer polymer mixtures.
Another recent work described the transformation of PE, PP, and PS into methane (>97% purity) at 300–350 °C using near-stoichiometric amounts of H 2 in the presence of a Ru-modified zeolite as a catalyst [ 171 ].
6. Conclusions
Ideally, the route to achieve a sustainable society is to replace synthetic plastics. A plastic-free world, however, is presently utopistic, and great effort must be applied in the pursuit of a drastic change in end-of-life plastic waste treatment and management.
In this review, we presented a highlight of the very latest technologies being developed to enhance the recycling efficiency of polymers and to generate high-value products from plastic waste.
Mechanical recycling and chemical upcycling appear to be the most promising strategies, since incineration and landfill are more pollutant and, for the latter, plastic waste completely loses its value.
Although, in the last few years, researchers have focused on chemical treatments, mechanical recycling is still the more mature and better performing technique. The lack of adequate infrastructures and technologies is limiting the industrialization of chemical upcycling, as well as the replacement of current materials with more sustainable polymers.
Future solutions will mainly focus on the development of biodegradable materials, completely recyclable polymers, and depolymerization/repolymerization pathways that allow to maximize the plastic life cycle.
Waste is a very serious problem and is intimately related to environmental and social–economic impacts. The problem of waste must be considered holistically from governments, industries, and stakeholders to preserve human health and guarantee the world survival. A deep change in mentalities at all levels is necessary to approach the impact of humanity and the industry on the environment; therefore, a high level of information is required to achieve awareness and promote sustainable processes and products. Too much information is available today; thus, that the scientific community must help give clear and well-justified indications regarding the best technologies to be adopted in the future. The authors hope that this mini-review will contribute to this consciousness and positively impact future choices.

Overview of plastic recycling techniques.

General scheme of primary and secondary recycling processes.

PET chemical recycling routes and product desired.

Low-energy catalyzed methanolysis of PET.

PET aminolysis via monoethanolamine (MEA).

General scheme of thermal recycling processes.
Author Contributions
Conceptualization, V.B.; writing—original draft preparation, V.B., M.F., R.S., C.B. and M.A.-A.; writing—review and editing, V.B., M.F., R.S., C.B. and M.A.-A.; supervision, V.B. All authors read and agreed to the published version of the manuscript.
This research was funded by POR FESR Veneto 2014–2020 Asse 1. Azione 1.1.4 (project title: Advanced waste recovery systems–ID 10057503).
Institutional Review Board Statement
Informed consent statement, data availability statement, conflicts of interest.
The authors declare no conflict of interest.
Publisher’s Note: MDPI stays neutral with regard to jurisdictional claims in published maps and institutional affiliations.
Energizing Ethical Recycling Intention Through Information Publicity: Insights from an Emerging Market Economy
- Original Paper
- Published: 15 April 2024

Cite this article
- Khalid Mehmood 1 ,
- Yaser Iftikhar 2 ,
- Fauzia Jabeen 3 ,
- Ali Nawaz Khan 1 &
- Hina Rehman 4
Plastic consumption is an important aspect of contemporary living, and studies that systematically examine consumers’ plastic waste recycling intentions from an ethical perspective are scarce. Considering the severity of plastic waste recycling problems globally based on the stimulus-organism-response paradigm, this study analyses how the information publicity influences consumers’ plastic waste recycling intentions from an ethical perspective in an emerging market economy. We investigate this link by focusing on the indirect effect of perceived social pressure and the moderating role of media disposition (i.e., media richness and content trustworthiness). Data from a three-wave field survey of 469 household consumers from China showed that social pressure mediated the positive relationship between information publicity and ethical recycling intentions. Additionally, media richness and content trustworthiness moderate the link between information publicity and consumers’ perceptions of social pressure. These findings provide encouraging evidence for applying the SOR paradigm to explain the ethical recycling intention of consumers. The findings may assist policymakers in motivating consumers to think ethically about plastic waste recycling.
This is a preview of subscription content, log in via an institution to check access.
Access this article
Price includes VAT (Russian Federation)
Instant access to the full article PDF.
Rent this article via DeepDyve
Institutional subscriptions
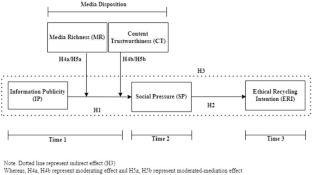
Data Availability
Data generated or analyzed during the study are available from the corresponding author by request.
To follow the established terminology in the area of business and consumer ethics the term ethics and morality are used as synonyms (Culiberg 2014 ) in this study.
Aboelmaged, M. (2021). E-waste recycling behaviour: An integration of recycling habits into the theory of planned behaviour. Journal of Cleaner Production, 278 , 124182. https://doi.org/10.1016/J.JCLEPRO.2020.124182
Article Google Scholar
Ajzen, I. (1991). The theory of planned behavior. Organizational Behavior and Human Decision Processes, 50 , 179–211.
Ajzen, I., & Fishbein, M. (1977). Belief, attitude, intention and behavior: An introduction to theory and research. Psychological Bulletin, 84 (5), 888–918. https://doi.org/10.2307/2065853
Andersch, H., Arnold, C., Seemann, A.-K., & Lindenmeier, J. (2019). Understanding ethical purchasing behavior: Validation of an enhanced stage model of ethical behavior. Journal of Retailing and Consumer Services, 48 , 50–59. https://doi.org/10.1016/j.jretconser.2019.02.004
Andrews, P., & Meyer, R. G. (2003). Marlowe-Crowne social desirability scale and short form C: Forensic norms. Journal of Clinical Psychology, 59 (4), 483–492. https://doi.org/10.1002/jclp.10136
Arjoon, S. (2007). Ethical decision-making: A case for the triple font theory. Journal of Business Ethics, 71 (4), 395–410. https://doi.org/10.1007/s10551-006-9142-1
Arli, D., Badejo, A., Carlini, J., France, C., Jebarajakirthy, C., Knox, K., Pentecost, R., Perkins, H., Thaichon, P., Sarker, T., & Wright, O. (2020). Predicting intention to recycle on the basis of the theory of planned behaviour. International Journal of Nonprofit and Voluntary Sector Marketing . https://doi.org/10.1002/nvsm.1653
Arli, D., & Pekerti, A. (2017). Who is more ethical? Cross-cultural comparison of consumer ethics between religious and non-religious consumers. Journal of Consumer Behaviour, 16 (1), 82–98. https://doi.org/10.1002/cb.1607
Attiq, S., Rasool, H., & Iqbal, S. (2017). The impact of supportive work environment, trust, and self-efficacy on organizational learning and its effectiveness: A stimulus-organism response approach. Business & Economic Review, 9 (2), 773–100. https://doi.org/10.22547/BER/9.2.4
Auger, P., Devinney, T. M., Louviere, J. J., & Burke, P. F. (2010). The importance of social product attributes in consumer purchasing decisions: A multi-country comparative study. International Business Review, 19 (2), 140–159.
Bamberg, S., & Möser, G. (2007). Twenty years after Hines, Hungerford, and Tomera: A new meta-analysis of psycho-social determinants of pro-environmental behaviour. Journal of Environmental Psychology, 27 (1), 14–25. https://doi.org/10.1016/j.jenvp.2006.12.002
Barnett, C., Cloke, P., Clarke, N., & Malpass, A. (2005). Consuming ethics: Articulating the subjects and spaces of ethical consumption. Antipode, 37 (1), 23–45. https://doi.org/10.1111/j.0066-4812.2005.00472.x
Berenguer, J. (2010). The effect of empathy in environmental moral reasoning. Environment and Behavior, 42 (1), 110–134.
Bergan, D. E., Carnahan, D., Lajevardi, N., Medeiros, M., Reckhow, S., & Thorson, K. (2021). Promoting the youth vote: The role of informational cues and social pressure. Political Behavior . https://doi.org/10.1007/s11109-021-09686-x
Bernstad, A. (2014). Household food waste separation behavior and the importance of convenience. Waste Management, 34 (7), 1317–1323. https://doi.org/10.1016/j.wasman.2014.03.013
Bigne, E., Chatzipanagiotou, K., & Ruiz, C. (2020). Pictorial content, sequence of conflicting online reviews and consumer decision-making: The stimulus-organism-response model revisited. Journal of Business Research, 115 , 403–416. https://doi.org/10.1016/j.jbusres.2019.11.031
Bortoleto, A. P., Kurisu, K. H., & Hanaki, K. (2012). Model development for household waste prevention behaviour. Waste Management, 32 (12), 2195–2207. https://doi.org/10.1016/J.WASMAN.2012.05.037
Botetzagias, I., Dima, A. F., & Malesios, C. (2015). Extending the theory of planned behavior in the context of recycling: The role of moral norms and of demographic predictors. Resources, Conservation and Recycling, 95 (99294), 58–67. https://doi.org/10.1016/j.resconrec.2014.12.004
Brislin, R. W. (1980). Translation and content analysis of oral and written material. In H. C. Triandis & J. W. Berry (Eds.), Handbook of cross-cultural psychology: Methodology (pp. 389–444). Allyn and Bacon.
Google Scholar
Cao, D., Meadows, M., Wong, D., & Xia, S. (2021). Understanding consumers’ social media engagement behaviour: An examination of the moderation effect of social media context. Journal of Business Research, 122 , 835–846. https://doi.org/10.1016/j.jbusres.2020.06.025
Center for International Environmental Law. (2020). Convention on plastic pollution: Toward a new global agreement to address plastic pollution . Thought-starter . Accessed 20 June 2021. Available at: https://www.ciel.org/reports/convention-on-plastic-pollution-toward-a-new-global-agreement-to-address-plastic-pollution/
CGTN. (2018). Reverse vending machine eats cans, paper and bottles . Accessed on 20 July 2021. Available at: https://news.cgtn.com/news/3d3d414d78416a4d30457a6333566d54/share_p.html
CGTN. (2021). China’s war on plastic waste . Accessed on 17 July 2021. Available at: https://news.cgtn.com/news/2021-01-27/China-s-war-on-plastic-waste-XmFcetAnTy/index.html
Chalak, A., Abou-Daher, C., Chaaban, J., & Abiad, M. G. (2016). The global economic and regulatory determinants of household food waste generation: A cross-country analysis. Waste Management, 48 , 418–422. https://doi.org/10.1016/j.wasman.2015.11.040
Chan, L., & Bishop, B. (2013). A moral basis for recycling: Extending the theory of planned behaviour. Journal of Environmental Psychology, 36 , 96–102. https://doi.org/10.1016/j.jenvp.2013.07.010
Chan, R. Y. K., Wong, Y. H., & Leung, T. K. P. (2008). Applying ethical concepts to the study of “green” consumer behavior: An analysis of Chinese consumers’ intentions to bring their own shopping bags. Journal of Business Ethics, 79 (4), 469–481.
Chang, C. C. (2013). Exploring the determinants of e-learning systems continuance intention in academic libraries. Library Management, 34 (1), 40–55. https://doi.org/10.1108/01435121311298261
Chen, M.-F., & Tung, P.-J. (2010). The moderating effect of perceived lack of facilities on consumers’ recycling intentions. Environment and Behavior, 42 (6), 824–844.
Chi, M., George, J. F., Huang, R., & Wang, P. (2020). Unraveling sustainable behaviors in the sharing economy: An empirical study of bicycle-sharing in China. Journal of Cleaner Production, 260 , 120962. https://doi.org/10.1016/j.jclepro.2020.120962
Chung, J., & Monroe, G. S. (2003). Exploring social desirability bias. Journal of Business Ethics, 44 (4), 291–302. https://doi.org/10.1023/A:1023648703356
Coleman, L. J., Bahnan, N., Kelkar, M., & Curry, N. (2011). Walking the walk: How the theory of reasoned action explains adult and student intentions to go green. Journal of Applied Business Research, 27 (3), 107–116.
Culiberg, B. (2014). Towards an understanding of consumer recycling from an ethical perspective. International Journal of Consumer Studies, 38 (1), 90–97. https://doi.org/10.1111/ijcs.12068
Culiberg, B., & Bajde, D. (2013). Consumer recycling: An ethical decision-making process. Journal of Consumer Behaviour, 12 (6), 449–459. https://doi.org/10.1002/cb.1446
Daft, R. L., & Lengel, R. H. (1986). Organizational information requirements, media richness and structural design. Management Science, 32 (5), 554–571.
Dermody, J., Koenig-Lewis, N., Zhao, A. L., & Hanmer-Lloyd, S. (2018). Appraising the influence of pro-environmental self-identity on sustainable consumption buying and curtailment in emerging markets: Evidence from China and Poland. Journal of Business Research, 86 , 333–343. https://doi.org/10.1016/j.jbusres.2017.09.041
Detert, J. R., & Edmondson, A. C. (2011). Implicit voice theories: Taken-for-granted rules of self-censorship at work. Academy of Management Journal, 54 (3), 461–488. https://doi.org/10.5465/amj.2011.61967925
Dhir, A., Koshta, N., Goyal, R. K., Sakashita, M., & Almotairi, M. (2021). Behavioral reasoning theory (BRT) perspectives on E-waste recycling and management. Journal of Cleaner Production, 280 , 124269. https://doi.org/10.1016/j.jclepro.2020.124269
Dickson, M. A. (2005). Identifying and profiling apparel label users. The ethical consumer (pp. 155–171). Sage.
Chapter Google Scholar
do Valle, P. O., Reis, E., Menezes, J., & Rebelo, E. (2004). Behavioral determinants of household recycling participation. Environment and Behavior, 36 (4), 505–540. https://doi.org/10.1177/0013916503260892
Dong, B., & Ge, J. (2022). What affects consumers’ intention to recycle retired EV batteries in China? Journal of Cleaner Production, 359 , 132065.
Dursun, İ., Tümer Kabadayı, E., & Tuğer, A. T. (2017). Application of Value-Belief-Norm theory to responsible post consumption behaviors: Recycling and reuse. In: International congress of the new approaches and technologies for sustainable development, proceeding book , 1 (September 2017), pp. 21–24.
Echegaray, F., & Hansstein, F. V. (2017). Assessing the intention-behavior gap in electronic waste recycling: The case of Brazil. Journal of Cleaner Production, 142 , 180–190. https://doi.org/10.1016/j.jclepro.2016.05.064
Ecker, U. K., Lewandowsky, S., Cook, J., Schmid, P., Fazio, L. K., Brashier, N., Kendeou, P., Vraga, E. K., & Amazeen, M. A. (2022). The psychological drivers of misinformation belief and its resistance to correction. Nature Reviews Psychology, 1 (1), 13–29. https://doi.org/10.1038/s44159-021-00006-y
Edwards, J. R., & Lambert, L. S. (2007). Methods for integrating moderation and mediation: A general analytical framework using moderated path analysis. Psychological Methods, 12 (1), 1–22.
European Environment Agency. (2023). EC, 2020, “2050 long-term strategy”—European Environment Agency. Policy Document .
European Commission. (2023). Waste framework directive. Environment .
Ewing, G. (2001). Altruistic, egoistic, and normative effects on curbside recycling. Environment and Behavior, 33 (6), 733–764. https://doi.org/10.1177/00139160121973223
Ferdous, W., Manalo, A., Siddique, R., Mendis, P., Zhuge, Y., Wong, H. S., Lokuge, W., Aravinthan, T., & Schubel, P. (2021). Recycling of landfill wastes (tyres, plastics and glass) in construction—A review on global waste generation, performance, application and future opportunities. Resources, Conservation and Recycling, 173 , 105745. https://doi.org/10.1016/j.resconrec.2021.105745
Fernando, M., & Chowdhury, R. M. M. I. (2010). The relationship between spiritual well-being and ethical orientations in decision making: An empirical study with business executives in Australia. Journal of Business Ethics, 95 (2), 211–225. https://doi.org/10.1007/s10551-009-0355-y
Fu, S., Chen, X., & Zheng, H. (2021). Exploring an adverse impact of smartphone overuse on academic performance via health issues: A stimulus-organism-response perspective. Behaviour & Information Technology, 40 (7), 663–675. https://doi.org/10.1080/0144929X.2020.1716848
Gillani, A., Kutaula, S., Leonidou, L. C., & Christodoulides, P. (2021). The impact of proximity on consumer fair trade engagement and purchasing behavior: The moderating role of empathic concern and hypocrisy. Journal of Business Ethics, 169 (3), 557–577. https://doi.org/10.1007/s10551-019-04278-6
Goodman, J. S., & Blum, T. C. (1996). Assessing the non-random sampling effects of subject attrition in longitudinal research. Journal of Management, 22 (4), 627–652.
Gregory-Smith, D., Manika, D., & Demirel, P. (2017). Green intentions under the blue flag: Exploring differences in EU consumers’ willingness to pay more for environmentally-friendly products. Business Ethics: A European Review, 26 (3), 205–222. https://doi.org/10.1111/beer.12151
Hair, J. F., Black, W. C., Babin, B. J., & Anderson, R. E. (2010). Multivariate data analysis: A global perspective . Prentice Hall.
Hameed, I., Khan, K., Waris, I., & Zainab, B. (2021). Factors influencing the sustainable consumer behavior concerning the recycling of plastic waste. Environmental Quality Management . https://doi.org/10.1002/tqem.21815
Han, H., Yu, J., Kim, H.-C., & Kim, W. (2018). Impact of social/personal norms and willingness to sacrifice on young vacationers’ pro-environmental intentions for waste reduction and recycling. Journal of Sustainable Tourism, 26 (12), 2117–2133. https://doi.org/10.1080/09669582.2018.1538229
Hayes, A. F. (2018). Introduction to mediation, moderation, and conditional process analysis: A regression-based approach (2nd ed.). Guilford Press.
He, X., & Zhan, W. (2018). How to activate moral norm to adopt electric vehicles in China? An empirical study based on extended norm activation theory. Journal of Cleaner Production, 172 , 3546–3556. https://doi.org/10.1016/j.jclepro.2017.05.088
Heidari, A., Kolahi, M., Behravesh, N., Ghorbanyon, M., Ehsanmansh, F., Hashemolhosini, N., & Zanganeh, F. (2018). Youth and sustainable waste management: A SEM approach and extended theory of planned behavior. Journal of Material Cycles and Waste Management, 20 (4), 2041–2053. https://doi.org/10.1007/s10163-018-0754-1
Hills, T. T. (2019). The dark side of information proliferation. Perspectives on Psychological Science, 14 (3), 323–330. https://doi.org/10.1177/1745691618803647
Hines, C., & Ames, A. (2000). Ethical consumerism. A research study conducted for the co-operative bank . Mori.
Hofstede, G. (2001). Culture’s consequences: Comparing values, behaviors, institutions and organizations across nations . Sage.
Hornik, J., Cherian, J., Madansky, M., & Narayana, C. (1995). Determinants of recycling behavior: A synthesis of research results. The Journal of Socio-Economics, 24 (1), 105–127. https://doi.org/10.1016/1053-5357(95)90032-2
Hosta, M., & Zabkar, V. (2021). Antecedents of Environmentally and socially responsible sustainable consumer behavior. Journal of Business Ethics, 171 (2), 273–293. https://doi.org/10.1007/s10551-019-04416-0
Huang, M., Law, K. M. Y., Geng, S., Niu, B., & Kettunen, P. (2022). Predictors of waste sorting and recycling behavioural intention among youths: Evidence from Shenzhen, China and Turku, Finland. Waste Management & Research, 40 (6), 721–735.
Jacoby, J. (2002). Stimulus-organism-response reconsidered: An evolutionary step in modeling (consumer) behavior. Journal of Consumer Psychology, 12 (1), 51–57. https://doi.org/10.1207/153276602753338081
Jung, H. J., Kim, H., & Oh, K. W. (2016). Green leather for ethical consumers in China and Korea: facilitating ethical consumption with value–belief–attitude logic. Journal of Business Ethics, 135 (3), 483–502. https://doi.org/10.1007/s10551-014-2475-2
Khan, F., Ahmed, W., & Najmi, A. (2019). Understanding consumers’ behavior intentions towards dealing with the plastic waste: Perspective of a developing country. Resources, Conservation and Recycling, 142 , 49–58. https://doi.org/10.1016/j.resconrec.2018.11.020
Kim, S. B., & Kim, D. Y. (2014). The effects of message framing and source credibility on green messages in hotels. Cornell Hospitality Quarterly, 55 (1), 64–75. https://doi.org/10.1177/1938965513503400
Klöckner, C. A. (2013). A comprehensive model of the psychology of environmental behaviour—A meta-analysis. Global Environmental Change, 23 (5), 1028–1038. https://doi.org/10.1016/j.gloenvcha.2013.05.014
Kredentser, M. S., Fabrigar, L. R., Smith, S. M., & Fulton, K. (2012). Following what people think we should do versus what people actually do: Elaboration as a moderator of the impact of descriptive and injunctive norms. Social Psychological and Personality Science, 3 (3), 341–347. https://doi.org/10.1177/1948550611420481
Kumar, A. (2019). Exploring young adults’ e-waste recycling behaviour using an extended theory of planned behaviour model: A cross-cultural study. Resources, Conservation and Recycling, 141 , 378–389. https://doi.org/10.1016/j.resconrec.2018.10.013
Kumar, S., Talwar, S., Murphy, M., Kaur, P., & Dhir, A. (2021). A behavioural reasoning perspective on the consumption of local food. A study on REKO, a social media-based local food distribution system. Food Quality and Preference, 93 , 104264. https://doi.org/10.1016/j.foodqual.2021.104264
Kutaula, S., Gillani, A., Leonidou, L. C., & Christodoulides, P. (2019). Personality as a driver of consumer fair trade engagement and ethically-minded behavior. Academy of Management Proceedings, 2019 (1), 13750. https://doi.org/10.5465/AMBPP.2019.13750abstract
Kwok, T. F., Yeung, C. H., & Xu, Y. (2017). Swaying public opinion on nuclear energy: A field experiment in Hong Kong. Utilities Policy . https://doi.org/10.1016/j.jup.2017.04.001
Lee, H., Kim, J., & Kim, J. (2007). Determinants of success for application service provider: An empirical test in small businesses. International Journal of Human-Computer Studies, 65 (9), 796–815. https://doi.org/10.1016/J.IJHCS.2007.04.004
Liu, Y., Zhu, J., Li, E. Y., Meng, Z., & Song, Y. (2020). Environmental regulation, green technological innovation, and eco-efficiency: The case of Yangtze river economic belt in China. Technological Forecasting and Social Change, 155 , 119993. https://doi.org/10.1016/j.techfore.2020.119993
Liu, Z., Yang, J. Z., Bloomfield, A., Clark, S. S., & Shelly, M. A. (2022b). Predicting recycling intention in New York state: The impact of cognitive and social factors. Environmental Development, 43 , 100712. https://doi.org/10.1016/j.envdev.2022.100712
Liu, Z., Yang, J. Z., Clark, S. S., & Shelly, M. A. (2022a). Recycling as a planned behavior: The moderating role of perceived behavioral control. Environment, Development and Sustainability, 24 (9), 11011–11026. https://doi.org/10.1007/s10668-021-01894-z
Lyu, Y., Wu, L.-Z., Ye, Y., Kwan, H. K., & Chen, Y. (2022). Rebellion under exploitation: How and when exploitative leadership evokes employees’ workplace deviance. Journal of Business Ethics . https://doi.org/10.1007/s10551-022-05207-w
Ma, J., Hipel, K. W., Hanson, M. L., Cai, X., & Liu, Y. (2018). An analysis of influencing factors on municipal solid waste source-separated collection behavior in Guilin, China by using the theory of planned behavior. Sustainable Cities and Society, 37 , 336–343. https://doi.org/10.1016/j.scs.2017.11.037
Manika, D., Antonetti, P., Papagiannidis, S., & Guo, X. (2021). How pride triggered by pro-environmental technology adoption spills over into conservation behaviours: A social business application. Technological Forecasting and Social Change, 172 , 121005. https://doi.org/10.1016/J.TECHFORE.2021.121005
Manika, D., Wells, V. K., Gregory-Smith, D., & Gentry, M. (2015). The impact of individual attitudinal and organisational variables on workplace environmentally friendly behaviours. Journal of Business Ethics, 126 (4), 663–684. https://doi.org/10.1007/s10551-013-1978-6
Mehmood, K., Iftikhar, Y., Khan, A. N., & Kwan, H. K. (2023). The nexus between high-involvement work practices and employees’ proactive behavior in public service organizations: A time-lagged moderated-mediation model. Psychology Research and Behavior Management, 16 , 1571–1586.
Mehmood, K., Jabeen, F., Rehman, H., Iftikhar, Y., & Khan, N. A. (2023). Understanding the boosters of employees’ voluntary pro-environmental behavior: a time-lagged investigation. Environment, development and sustainability (pp. 1–23). Springer.
Mehrabian, A., & Russell, J. A. (1974). An approach to environmental psychology . The MIT Press.
Meng, X., Tan, X., Wang, Y., Wen, Z., Tao, Y., & Qian, Y. (2019). Investigation on decision-making mechanism of residents’ household solid waste classification and recycling behaviors. Resources, Conservation and Recycling. https://doi.org/10.1016/j.resconrec.2018.09.021
Mickaël, D. (2014). The comparative effectiveness of persuasion, commitment and leader block strategies in motivating sorting. Waste Management, 34 (4), 730–737. https://doi.org/10.1016/j.wasman.2014.01.006
Muthén, L. K., & Muthén, B. O. (2012). MPlus: statistical analysis with latent variables--User’s guide .
National Information Center. (2016). The history and future trend of urbanization development in my country. http://www.sic.gov.cn/News/455/6167.htm Accessed 6 May 2022.
Nguyen, H. T. T., Hung, R. J., Lee, C. H., & Nguyen, H. T. T. (2018). Determinants of residents’ E-waste recycling behavioral intention: A case study from Vietnam. Sustainability, 11 (1), 1–24. https://doi.org/10.3390/su11010164
Niaura, A. (2013). Using the theory of planned behavior to investigate the determinants of environmental behavior among youth. Environmental Research, Engineering and Management, 63 (1), 74–81.
Nketiah, E., Song, H., Cai, X., Adjei, M., Obuobi, B., Adu-Gyamfi, G., & Cudjoe, D. (2022). Predicting citizens’ recycling intention: Incorporating natural bonding and place identity into the extended norm activation model. Journal of Cleaner Production, 377 , 134425.
OECD. (2022). Plastic pollution is growing relentlessly as waste management and recycling fall short. OECD Better Policies for Better Lives . https://www.oecd.org/environment/plastic-pollution-is-growing-relentlessly-as-waste-management-and-recycling-fall-short.htm . Accessed 25 Sept 2022.
Onwezen, M. C., Antonides, G., & Bartels, J. (2013). The norm activation model: An exploration of the functions of anticipated pride and guilt in pro-environmental behaviour. Journal of Economic Psychology, 39 , 141–153. https://doi.org/10.1016/j.joep.2013.07.005
Osburg, V.-S., Yoganathan, V., Bartikowski, B., Liu, H., & Strack, M. (2020). Effects of ethical certification and ethical eWoM on talent attraction. Journal of Business Ethics, 164 (3), 535–548. https://doi.org/10.1007/s10551-018-4018-8
Oskamp, S., Harrington, M. J., Okuda, S. M., Edwards, T. C., Sherwood, D. L., & Swanson, D. C. (1991). Factors influencing household recycling behavior. Environment and Behavior, 23 (4), 494–519. https://doi.org/10.1177/0013916591234005
Ozcaglar-Toulouse, N., Shiu, E., & Shaw, D. (2006). In search of fair trade: Ethical consumer decision making in France. International Journal of Consumer Studies, 30 (5), 502–514.
Papadopoulos, N., Cleveland, M., Bartikowski, B., & Yaprak, A. (2018). Of countries, places and product/brand place associations: An inventory of dispositions and issues relating to place image and its effects. Journal of Product & Brand Management, 27 (7), 735–753.
Park, J., & Ha, S. (2014). Understanding consumer recycling behavior: Combining the theory of planned behavior and the norm activation model. Family and Consumer Sciences Research Journal, 42 (3), 278–291. https://doi.org/10.1111/fcsr.12061
Pettigrew, K., Fidel, R., & Bruce, H. (2001). Conceptual frameworks in information behavior. Annual Review of Information Science and Technology, 35 , 43–78.
Podsakoff, P. M., MacKenzie, S. B., Lee, J.-Y., & Podsakoff, N. P. (2003). Common method biases in behavioral research: A critical review of the literature and recommended remedies. Journal of Applied Psychology, 88 (5), 879–903.
Preacher, K. J., & Hayes, A. F. (2004). SPSS and SAS procedures for estimating indirect effects in simple mediation models. Behavior Research Methods, Instruments, and Computers, 36 (4), 717–731.
Preacher, K. J., & Kelley, K. (2011). Effect size measures for mediation models: Quantitative strategies for communicating indirect effects. Psychological Methods, 16 (2), 93–115.
Randall, D. M., & Gibson, A. M. (1990). Methodology in business ethics research: A review and critical assessment. Journal of Business Ethics, 9 (6), 457–471. https://doi.org/10.1007/BF00382838
Rawwas, M. Y. A. (1996). Consumer ethics: An empirical investigation of the ethical beliefs of Austrian consumers. Journal of Business Ethics, 15 (9), 1009–1019. https://doi.org/10.1007/BF00705579
Rawwas, M. Y. A., & Singhapakdi, A. (1998). Do consumers’ ethical beliefs vary with age? A substantiation of Kohlberg’s typology in marketing. Journal of Marketing Theory and Practice, 6 (2), 26–38. https://doi.org/10.1080/10696679.1998.11501793
Saphores, J. D. M., Ogunseitan, O. A., & Shapiro, A. A. (2012). Willingness to engage in a pro-environmental behavior: An analysis of e-waste recycling based on a national survey of U.S. households. Resources, Conservation and Recycling, 60 , 49–63. https://doi.org/10.1016/J.RESCONREC.2011.12.003
Schwartz, S. H. (1977). Normative influences on altruism. Advances in Experimental Social Psychology, 10 , 221–279. https://doi.org/10.1016/S0065-2601(08)60358-5
Shang, Y., Mehmood, K., Iftikhar, Y., Aziz, A., Tao, X., & Shi, L. (2021). Energizing intention to visit rural destinations: How social media disposition and social media use boost tourism through information publicity. Frontiers in Psychology . https://doi.org/10.3389/fpsyg.2021.782461
Shang, Y., Rehman, H., Mehmood, K., Xu, A., Iftikhar, Y., Wang, Y., & Sharma, R. (2022). The nexuses between social media marketing activities and consumers’ engagement behaviour: A two-wave time-lagged study. Frontiers in Psychology . https://doi.org/10.3389/fpsyg.2022.811282
Shaw, D., & Clarke, I. (1999). Belief formation in ethical consumer groups: An exploratory study. Marketing Intelligence & Planning . https://doi.org/10.1108/02634509910260968
Shaw, D., Grehan, E., Shiu, E., Hassan, L., & Thomson, J. (2005). An exploration of values in ethical consumer decision making. Journal of Consumer Behaviour: An International Research Review, 4 (3), 185–200.
Shaw, D., & Shiu, E. (2003). Ethics in consumer choice: A multivariate modelling approach. European Journal of Marketing . https://doi.org/10.1108/03090560310487202
Shi, H., Fan, J., & Zhao, D. (2017). Predicting household PM2.5-reduction behavior in Chinese urban areas: An integrative model of theory of planned behavior and norm activation theory. Journal of Cleaner Production, 145 , 64–73. https://doi.org/10.1016/j.jclepro.2016.12.169
Sobel, M. E. (1982). Asymptotic confidence intervals for indirect effects in structural equation models. Sociological Methodology, 13 , 290–312.
Song, S. Y., & Kim, Y.-K. (2018). Theory of virtue ethics: Do consumers’ good traits predict their socially responsible consumption? Journal of Business Ethics, 152 (4), 1159–1175. https://doi.org/10.1007/s10551-016-3331-3
Spiekermann, S. (2015). Ethical IT innovation: A value-based system design approach . Auerbach Publications.
Book Google Scholar
Spink, A., & Cole, C. (2006). Human information behavior: Integrating diverse approaches and information use. Journal of the American Society for Information Science and Technology, 57 (1), 25–35. https://doi.org/10.1002/asi.20249
Stern, P. C., Kalof, L., Dietz, T., & Guagnano, G. A. (1995). Values, beliefs, and proenvironmental action: Attitude formation toward emergent attitude objects. Journal of Applied Social Psychology, 25 (18), 1611–1636. https://doi.org/10.1111/j.1559-1816.1995.tb02636.x
Sujata, M., Khor, K.-S., Ramayah, T., & Teoh, A. P. (2019). The role of social media on recycling behaviour. Sustainable Production and Consumption, 20 , 365–374. https://doi.org/10.1016/j.spc.2019.08.005
Tandon, A., Dhir, A., Kaur, P., Kushwah, S., & Salo, J. (2020). Behavioral reasoning perspectives on organic food purchase. Appetite, 154 , 104786. https://doi.org/10.1016/j.appet.2020.104786
Tang, Z., Chen, X., & Luo, J. (2011). Determining socio-psychological drivers for rural household recycling behavior in developing countries: A case study from Wugan, Hunan, China. Environment and Behavior, 43 (6), 848–877.
Tang, Z., Warkentin, M., & Wu, L. (2019). Understanding employees’ energy saving behavior from the perspective of stimulus-organism-responses. Resources, Conservation and Recycling, 140 , 216–223. https://doi.org/10.1016/j.resconrec.2018.09.030
Thøgersen, J. (1996). Recycling and morality: A critical review of the literature. Environment and Behavior, 28 (4), 536–558.
Tonglet, M., Phillips, P. S., & Read, A. D. (2004). Using the theory of planned behaviour to investigate the determinants of recycling behaviour: A case study from Brixworth, UK. Resources, Conservation and Recycling, 41 (3), 191–214.
UNEP. (2022). Visual feature | Beat plastic pollution. Accessed 7 Jan 2023. Available at: https://www.unep.org/interactives/beat-plastic-pollution/
Veissière, S. P. L., Constant, A., Ramstead, M. J. D., Friston, K. J., & Kirmayer, L. J. (2020). Thinking through other minds: A variational approach to cognition and culture. Behavioral and Brain Sciences . https://doi.org/10.1017/S0140525X19001213
Vicente, P., & Reis, E. (2008). Factors influencing households’ participation in recycling. Waste Management and Research, 26 (2), 140–146. https://doi.org/10.1177/0734242X07077371
Vitell, S. J., King, R. A., Howie, K., Toti, J.-F., Albert, L., Hidalgo, E. R., & Yacout, O. (2016). Spirituality, moral identity, and consumer ethics: A multi-cultural study. Journal of Business Ethics, 139 (1), 147–160. https://doi.org/10.1007/s10551-015-2626-0
Vitell, S. J., & Muncy, J. (2005). The Muncy-Vitell consumer ethics scale: A modification and application. Journal of Business Ethics, 62 (3), 267–275.
Wan, C., Shen, G. Q., & Yu, A. (2014). The role of perceived effectiveness of policy measures in predicting recycling behaviour in Hong Kong. Resources, Conservation and Recycling, 83 , 141–151. https://doi.org/10.1016/j.resconrec.2013.12.009
Wan, C., Shen, G. Q., & Yu, A. (2015). Key determinants of willingness to support policy measures on recycling: A case study in Hong Kong. Environmental Science & Policy, 54 , 409–418. https://doi.org/10.1016/j.envsci.2015.06.023
Wang, C., Zhu, T., Yao, H., & Sun, Q. (2020b). The impact of green information on the participation intention of consumers in online recycling: An experimental study. Sustainability . https://doi.org/10.3390/su12062498
Wang, H., Bai, R., Zhao, H., Hu, Z., & Li, Y. (2022). Why does frugality influence the recycling intention of waste materials? Frontiers in Psychology . https://doi.org/10.3389/fpsyg.2022.952010
Wang, H., Liu, X., Wang, N., Zhang, K., Wang, F., Zhang, S., Wang, R., Zheng, P., & Matsushita, M. (2020). Key factors influencing public awareness of household solid waste recycling in urban areas of China: A case study. Resources, Conservation and Recycling, 158 , 104813. https://doi.org/10.1016/j.resconrec.2020.104813
Wang, S., Lin, S., & Li, J. (2018c). Exploring the effects of non-cognitive and emotional factors on household electricity saving behavior. Energy Policy, 115 , 171–180. https://doi.org/10.1016/j.enpol.2018.01.012
Wang, S., Wang, J., Zhao, S., & Yang, S. (2019). Information publicity and resident’s waste separation behavior: An empirical study based on the norm activation model. Waste Management, 87 , 33–42. https://doi.org/10.1016/j.wasman.2019.01.038
Wang, Z., Dong, X., & Yin, J. (2018a). Antecedents of urban residents’ separate collection intentions for household solid waste and their willingness to pay: Evidence from China. Journal of Cleaner Production, 173 , 256–264. https://doi.org/10.1016/j.jclepro.2016.09.223
Wang, Z., Guo, D., Wang, X., Zhang, B., & Wang, B. (2018b). How does information publicity influence residents’ behaviour intentions around e-waste recycling? Resources, Conservation and Recycling, 133 , 1–9. https://doi.org/10.1016/j.resconrec.2018.01.014
Wang, Z., Zhang, B., Yin, J., & Zhang, X. (2011). Willingness and behavior towards e-waste recycling for residents in Beijing city, China. Journal of Cleaner Production, 19 (9–10), 977–984. https://doi.org/10.1016/J.JCLEPRO.2010.09.016
Werner, C. M., & Makela, E. (1998). Motivations and behaviors that support recycling. Journal of Environmental Psychology, 18 (4), 373–386. https://doi.org/10.1006/jevp.1998.0114
Williams, I. D., & Taylor, C. (2004). Maximising household waste recycling at civic amenity sites in Lancashire, England. Waste Management, 24 (9), 861–874. https://doi.org/10.1016/j.wasman.2004.02.002
Xiao, S., Dong, H., Geng, Y., Francisco, M.-J., Pan, H., & Wu, F. (2020). An overview of the municipal solid waste management modes and innovations in Shanghai, China. Environmental Science and Pollution Research, 27 (24), 29943–29953. https://doi.org/10.1007/s11356-020-09398-5
Xin, Z. (2012). Vending machines turn trash into treasure. Chinadaily .
Xin, Z. (2013). 'Reverse' vending machine sells idea of recycling. Chinadaily .
Ylä-Mella, J., Keiski, R. L., & Pongrácz, E. (2015). Electronic waste recovery in Finland: Consumers’ perceptions towards recycling and re-use of mobile phones. Waste Management, 45 , 374–384. https://doi.org/10.1016/j.wasman.2015.02.031
Zhang, B., Du, Z., Wang, B., & Wang, Z. (2019a). Motivation and challenges for e-commerce in e-waste recycling under “Big data” context: A perspective from household willingness in China. Technological Forecasting and Social Change, 144 , 436–444. https://doi.org/10.1016/J.TECHFORE.2018.03.001
Zhang, B., Lai, K., & hung, Wang, B., & Wang, Z. (2019b). From intention to action: How do personal attitudes, facilities accessibility, and government stimulus matter for household waste sorting? Journal of Environmental Management, 233 , 447–458. https://doi.org/10.1016/j.jenvman.2018.12.059
Zhang, H., Liu, J., Wen, Z., & guo, & Chen, Y. X. (2017). College students’ municipal solid waste source separation behavior and its influential factors: A case study in Beijing, China. Journal of Cleaner Production, 164 , 444–454. https://doi.org/10.1016/J.JCLEPRO.2017.06.224
Zhang, X., Liu, J., & Zhao, K. (2018). Antecedents of citizens’ environmental complaint intention in China: An empirical study based on norm activation model. Resources, Conservation and Recycling, 134 , 121–128. https://doi.org/10.1016/J.RESCONREC.2018.03.003
Zhong, H., & Huang, L. (2016). The empirical research on the consumers’ willingness to participate in e-waste recycling with a points reward system. Energy Procedia, 104 , 475–480. https://doi.org/10.1016/j.egypro.2016.12.080
Zorpas, A. A., & Lasaridi, K. (2013). Measuring waste prevention. Waste Management, 33 (5), 1047–1056. https://doi.org/10.1016/J.WASMAN.2012.12.017
Zou, X., & Savani, K. (2019). Descriptive norms for me, injunctive norms for you: Using norms to explain the risk gap. Judgment and Decision Making, 14 (6), 644–648.
Download references
Acknowledgements
We sincerely thank the Editor and the anonymous reviewers for their insightful evaluation of this manuscript during the revision process. Their constructive feedback and thoughtful suggestions significantly improved the quality and clarity of this paper.This study was funded by the China Postdoctoral Science Foundation (Grant Number: 2020M671236) and the Open Project of Micro & Small Enterprise Development Research Center in Hubei Province (Grant Number XWZD202205). All authors contributed equally to this manuscript.
Author information
Authors and affiliations.
Research Center of Hubei Micro and Small Enterprises Development, School of Economics and Management, Hubei Engineering University, Xiaogan, 432100, People’s Republic of China
Khalid Mehmood & Ali Nawaz Khan
AFPGMI, National University of Medical Sciences, Rawalpindi, 46000, Pakistan
Yaser Iftikhar
College of Business, Abu Dhabi University, P.O. Box 59911, Abu Dhabi, UAE
Fauzia Jabeen
Faculty of Management Sciences, National University of Modern Languages (NUML), Islamabad, 44000, Pakistan
Hina Rehman
You can also search for this author in PubMed Google Scholar
Corresponding author
Correspondence to Ali Nawaz Khan .
Ethics declarations
Conflict of interests.
The authors declare that they have no conflict of interest in submitting this paper to the Journal of Business Ethics.
Additional information
Publisher's note.
Springer Nature remains neutral with regard to jurisdictional claims in published maps and institutional affiliations.
Rights and permissions
Springer Nature or its licensor (e.g. a society or other partner) holds exclusive rights to this article under a publishing agreement with the author(s) or other rightsholder(s); author self-archiving of the accepted manuscript version of this article is solely governed by the terms of such publishing agreement and applicable law.
Reprints and permissions
About this article
Mehmood, K., Iftikhar, Y., Jabeen, F. et al. Energizing Ethical Recycling Intention Through Information Publicity: Insights from an Emerging Market Economy. J Bus Ethics (2024). https://doi.org/10.1007/s10551-024-05671-6
Download citation
Received : 02 November 2021
Accepted : 10 March 2024
Published : 15 April 2024
DOI : https://doi.org/10.1007/s10551-024-05671-6
Share this article
Anyone you share the following link with will be able to read this content:
Sorry, a shareable link is not currently available for this article.
Provided by the Springer Nature SharedIt content-sharing initiative
- Social pressure
- Media disposition
- Information publicity
- Ethical recycling intention
- Stimulus-organism-response paradigm
- Find a journal
- Publish with us
- Track your research

The Future of Plastic Recycling: Four Key Trends in 2024 for Plastic Recycling
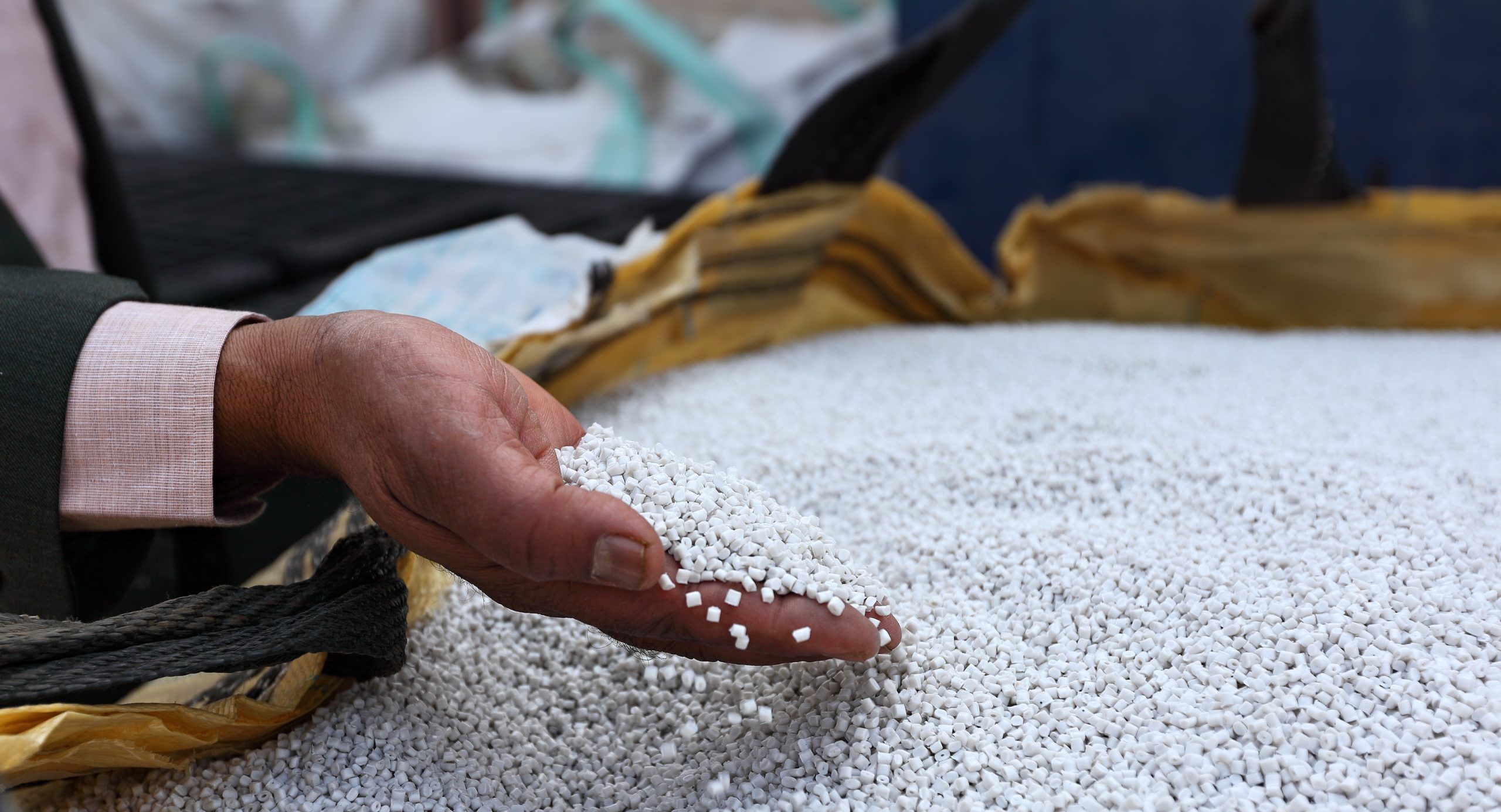
This article appeared in the January 2024 issue of Resource Recycling. Subscribe today for access to all print content.
I n the ever-evolving landscape of environmental sustainability, plastic recycling remains a cornerstone of our efforts to create a more circular economy. As we head into 2024, we stand at a pivotal moment, where the actions we take today can significantly shape the future of our planet.
I’m proud to serve as the president of the Association of Plastic Recyclers (APR). APR is the voice of plastic recyclers, with members working at every link of the recycling chain—from initial design to eventual remanufacturing.
As we look toward 2024, it’s useful to take stock of where we are today. The most important thing to know is that plastic recycling works. When plastics are collected, they are recycled. Last year, APR recycler members processed over 5 billion pounds of post-consumer plastics. That’s 5 billion pounds of plastic that did not end up in the ocean or in a landfill.
Here are some more useful numbers: The majority—approximately 80%—of rigid consumer plastic packaging is made of three types of resins: PET, HDPE and PP plastic. Over 70% of the PET and HDPE containers that people put into their curbside bins are sorted, processed and effectively recycled today. But, of course, not all of the consumer plastic out there gets put into recycling bins. As a result, the U.S. recycles PET, HDPE and PP plastics at a rate of 19.8%.
So recycling is working, but it could also be working better. APR members have the capacity today to more than double the recycling rate for PET, HDPE and PP if they could just get more supply of plastic packaging to process.
Recycling matters, and not only to reduce waste and protect our environment. Recycling is also an important economic engine for U.S. manufacturing. A strong recycling infrastructure is pivotal for building clean, resilient domestic supply chains. As we look toward the next year, four significant trends are poised to influence and drive the future of plastic recycling.
1. Inconsistent domestic demand for recycled content
Many U.S. companies have the same New Year’s resolution: to buy and use more recycled content in their products. American companies have already committed to buying three times more recycled PET by 2025 than is currently available in the domestic market. But, just as Americans sometimes waver on their January commitments to hit the gym or eat more veggies, U.S. companies sometimes back away from their recycled content commitments. Too often, brand companies cancel contracts for recycled material in favor of less expensive virgin resin or imported material. Addressing demand fluctuations would necessitate the creation of mandatory domestic recycled content minimums and long-term contracts for domestic recycled content supply.
2. Impact of high production of virgin plastic on recycling
Petrochemical companies are pumping out virgin plastic at record rates. The continued high production of virgin plastics directly impacts the recycling industry, as the proliferation of new, inexpensive plastics often undermines the market for recycled materials. Off-spec and wide-spec resin, byproducts of massive virgin production, are often sold even further below market rates, amplifying the cost differential with recycled material. When brand companies are making packaging decisions exclusively on price, it can be nearly impossible for recycled content to compete. Addressing the economic imbalance would require decoupling the value of recycled content from its price and redefining it as necessary and important with mandatory domestic content minimums.
3. Importance of verification and certification
When we think about content requirements, it will be increasingly important to also focus on verification and certification within the recycling process. In a circular economy, manufacturers are their own suppliers. Because we want brands to make more content from domestically sourced recycled material at the end of the chain, APR works to strengthen every link of the recycling chain.
The APR Design Guide offers essential guidelines for packaging designers to ensure their products are designed to be compatible with current recycling infrastructure—Circular by Design. The APR Design Guide is the gold standard, serving as the design platform for the U.S. Plastics PACT and often referenced across the globe in alignment with sister guides. When products are made for recycling, there is a significant reduction in contamination and overall improvement in the efficacy of recycling.
Similarly, the APR Sortation Potential Protocol aims to enhance the sorting efficiency of recyclable materials, a crucial step in the recycling process. Improved sortation methods can significantly increase the volume and quality of recyclables.
Finally, the APR PCR Certification Program guarantees buyers that they are truly getting post-consumer recycled material. This certification upholds the integrity of the recycled content market by maintaining transparency and trust in recycled plastics.
Establishing and adhering to the highest standardized protocols is critical for efficiency and credibility in recycling efforts.
4. Implications of changes on a local and global scale
Finally, recycling never operates in a vacuum. The industry is experiencing the pressures posed by local and global action to reduce plastic waste. One example is the U.N. Global Plastic Pollution Treaty, which aims to negotiate a global agreement to end plastic pollution. APR is an active stakeholder in both the U.N. Environment Programme and with the U.S. delegation as the group works to address important topics, including virgin plastic production, microplastics, chemicals of concern, expanding recycling, cleaning up fishing debris and much more. At the global level and here at home, APR is actively advocating for proven policies, like custom, locally appropriate extended producer responsibility (EPR) programs, and tailored, meaningful investments in recycling infrastructure.
As we move into the next year, these trends present a mix of challenges and opportunities for the plastic recycling industry. Recycling works—and it matters. Not only because it is one of the most visible opportunities to address problems caused by plastic packaging waste but also because it offers benefits, including strengthening the domestic supply chain, reducing energy consumption, lowering greenhouse gas emissions and replacing the use of virgin material derived from petrochemicals and fossil fuels.
APR remains committed to navigating these waters, advocating for a sustainable and effective recycling system. Through collaboration, innovation and policy advocacy, we are determined to make significant strides toward a more sustainable future for all.
Steve Alexander is president of the Association of Plastic Recyclers.
Subscribe today for weekly updates
- Name First Last
- Resource Recycling (weekly)
- Plastics Recycling Update (weekly)
- E-Scrap News (weekly)
- Friday Recap (weekly)
- Recycling Policy Now (monthly)
- In addition to our e-newsletters, Resource Recycling, Inc. will occasionally send emails related to the specific recycling industry sectors you've selected. As a subscriber, your information will not be sold. You may opt-out at any time with links at the bottom of our e-mails. You may review our privacy policy at any time.
- Comments This field is for validation purposes and should be left unchanged.
- Share full article

There’s an Explosion of Plastic Waste. Big Companies Say ‘We’ve Got This.’
Big brands like Procter & Gamble and Nestlé say a new generation of plants will help them meet environmental goals, but the technology is struggling to deliver.
Recycled polypropylene pellets at a PureCycle Technologies plant in Ironton, Ohio. Credit... Maddie McGarvey for The New York Times
Supported by

By Hiroko Tabuchi
- Published April 5, 2024 Updated April 8, 2024
By 2025, Nestle promises not to use any plastic in its products that isn’t recyclable. By that same year, L’Oreal says all of its packaging will be “refillable, reusable, recyclable or compostable.”
And by 2030, Procter & Gamble pledges that it will halve its use of virgin plastic resin made from petroleum.
To get there, these companies and others are promoting a new generation of recycling plants, called “advanced” or “chemical” recycling, that promise to recycle many more products than can be recycled today.
So far, advanced recycling is struggling to deliver on its promise. Nevertheless, the new technology is being hailed by the plastics industry as a solution to an exploding global waste problem.
The traditional approach to recycling is to simply grind up and melt plastic waste. The new, advanced-recycling operators say they can break down the plastic much further, into more basic molecular building blocks, and transform it into new plastic.
PureCycle Technologies, a company that features prominently in Nestlé, L’Oréal, and Procter & Gamble’s plastics commitments, runs one such facility, a $500 million plant in Ironton, Ohio. The plant was originally to start operating in 2020 , with the capacity to process as much as 182 tons of discarded polypropylene, a hard-to-recycle plastic used widely in single-use cups, yogurt tubs, coffee pods and clothing fibers, every day.

But PureCycle’s recent months have instead been filled with setbacks: technical issues at the plant, shareholder lawsuits, questions over the technology and a startling report from contrarian investors who make money when a stock price falls. They said that they had flown a drone over the facility that showed that the plant was far from being able to make much new plastic.
PureCycle, based in Orlando, Fla., said it remained on track. “We’re ramping up production,” its chief executive, Dustin Olson, said during a recent tour of the plant, a constellation of pipes, storage tanks and cooling towers in Ironton, near the Ohio River. “We believe in this technology. We’ve seen it work,” he said. “We’re making leaps and bounds.”
Nestlé, Procter & Gamble and L’Oréal have also expressed confidence in PureCycle. L’Oréal said PureCycle was one of many partners developing a range of recycling technologies. P.&G. said it hoped to use the recycled plastic for “numerous packaging applications as they scale up production.” Nestlé didn’t respond to requests for comment, but has said it is collaborating with PureCycle on “groundbreaking recycling technologies.”
PureCycle’s woes are emblematic of broad trouble faced by a new generation of recycling plants that have struggled to keep up with the growing tide of global plastic production, which scientists say could almost quadruple by midcentury .
A chemical-recycling facility in Tigard, Ore., a joint venture between Agilyx and Americas Styrenics, is in the process of shutting down after millions of dollars in losses. A plant in Ashley, Ind., that had aimed to recycle 100,000 tons of plastic a year by 2021 had processed only 2,000 tons in total as of late 2023, after fires, oil spills and worker safety complaints.
At the same time, many of the new generation of recycling facilities are turning plastic into fuel, something the Environmental Protection Agency doesn’t consider to be recycling, though industry groups say some of that fuel can be turned into new plastic .
Overall, the advanced recycling plants are struggling to make a dent in the roughly 36 million tons of plastic Americans discard each year, which is more than any other country. Even if the 10 remaining chemical-recycling plants in America were to operate at full capacity, they would together process some 456,000 tons of plastic waste, according to a recent tally by Beyond Plastics , a nonprofit group that advocates stricter controls on plastics production. That’s perhaps enough to raise the plastic recycling rate — which has languished below 10 percent for decades — by a single percentage point.
For households, that has meant that much of the plastic they put out for recycling doesn’t get recycled at all, but ends up in landfills. Figuring out which plastics are recyclable and which aren’t has turned into, essentially, a guessing game . That confusion has led to a stream of non-recyclable trash contaminating the recycling process, gumming up the system.
“The industry is trying to say they have a solution,” said Terrence J. Collins, a professor of chemistry and sustainability science at Carnegie Mellon University. “It’s a non-solution.”
‘Molecular washing machine’
It was a long-awaited day last June at PureCycle’s Ironton facility: The company had just produced its first batch of what it describes as “ultra-pure” recycled polypropylene pellets.
That milestone came several years late and with more than $350 million in cost overruns. Still, the company appeared to have finally made it. “Nobody else can do this,” Jeff Kramer, the plant manager, told a local news crew .
PureCycle had done it by licensing a game-changing method — developed by Procter & Gamble researchers in the mid-2010s, but unproven at scale — that uses solvent to dissolve and purify the plastic to make it new again. “It’s like a molecular washing machine,” Mr. Olson said.
There’s a reason Procter & Gamble, Nestlé and L’Oréal, some of the world’s biggest users of plastic, are excited about the technology. Many of their products are made from polypropylene, a plastic that they transform into a plethora of products using dyes and fillers. P.&G. has said it uses more polypropylene than any other plastic, more than a half-million tons a year.
But those additives make recycling polypropylene more difficult.
The E.P.A. estimates that 2.7 percent of polypropylene packaging is reprocessed. But PureCycle was promising to take any polypropylene — disposable beer cups, car bumpers, even campaign signs — and remove the colors, odors, and contaminants to transform it into new plastic.
Soon after the June milestone, trouble hit.
On Sept. 13, PureCycle disclosed that its plant had suffered a power failure the previous month that had halted operations and caused a vital seal to fail. That meant the company would be unable to meet key milestones, it told lenders.
Then in November, Bleecker Street Research — a New York-based short-seller, an investment strategy that involves betting that a company’s stock price will fall — published a report asserting that the white pellets that had rolled off PureCycle’s line in June weren’t recycled from plastic waste. The short-sellers instead claimed that the company had simply run virgin polypropylene through the system as part of a demonstration run.
Mr. Olson said PureCycle hadn’t used consumer waste in the June 2023 run, but it hadn’t used virgin plastic, either. Instead it had used scrap known as “post industrial,” which is what’s left over from the manufacturing process and would otherwise go to a landfill, he said.
Bleecker Street also said it had flown heat-sensing drones over the facility and said it found few signs of commercial-scale activity. The firm also raised questions about the solvent PureCycle was using to break down the plastic, calling it “a nightmare concoction” that was difficult to manage.
PureCycle is now being sued by other investors who accuse the company of making false statements and misleading investors about its setbacks.
Mr. Olson declined to describe the solvent. Regulatory filings reviewed by The New York Times indicate that it is butane, a highly flammable gas, stored under pressure. The company’s filing described the risks of explosion, citing a “worst case scenario” that could cause second-degree burns a half-mile away, and said that to mitigate the risk the plant was equipped with sprinklers, gas detectors and alarms.
Chasing the ‘circular economy’
It isn’t unusual, of course, for any new technology or facility to experience hiccups. The plastics industry says these projects, once they get going, will bring the world closer to a “circular” economy, where things are reused again and again.
Plastics-industry lobbying groups are promoting chemical recycling. At a hearing in New York late last year, industry lobbyists pointed to the promise of advanced recycling in opposing a packaging-reduction bill that would eventually mandate a 50 percent reduction in plastic packaging. And at negotiations for a global plastics treaty , lobby groups are urging nations to consider expanding chemical recycling instead of taking steps like restricting plastic production or banning plastic bags.
A spokeswoman for the American Chemistry Council, which represents plastics makers as well as oil and gas companies that produce the building blocks of plastic, said that chemical recycling potentially “complements mechanical recycling, taking the harder-to-recycle plastics that mechanical often cannot.”
Environmental groups say the companies are using a timeworn strategy of promoting recycling as a way to justify selling more plastic, even though the new recycling technology isn’t ready for prime time. Meanwhile, they say, plastic waste chokes rivers and streams, piles up in landfills or is exported .
“These large consumer brand companies, they’re out over their skis,” said Judith Enck, the president of Beyond Plastics and a former regional E.P.A. administrator. “Look behind the curtain, and these facilities aren’t operating at scale, and they aren’t environmentally sustainable,” she said.
The better solution, she said, would be, “We need to make less plastic.”
Touring the plant
Mr. Olson recently strolled through a cavernous warehouse at PureCycle’s Ironton site, built at a former Dow Chemical plant. Since January, he said, PureCycle has been processing mainly consumer plastic waste and has produced about 1.3 million pounds of recycled polypropylene, or about 1 percent of its annual production target.
“This is a bag that would hold dog food,” he said, pointing to a bale of woven plastic bags. “And these are fruit carts that you’d see in street markets. We can recycle all of that, which is pretty cool.”
The plant was dealing with a faulty valve discovered the day before, so no pellets were rolling off the line. Mr. Olson pulled out a cellphone to show a photo of a valve with a dark line ringing its interior. “It’s not supposed to look like that,” he said.
The company later sent video of Mr. Olson next to white pellets once again streaming out of its production line.
PureCycle says every kilogram of polypropylene it recycles emits about 1.54 kilograms of planet-warming carbon dioxide. That’s on par with a commonly used industry measure of emissions for virgin polypropylene. PureCycle said that it was improving on that measure.
Nestlé, L’Oréal and Procter & Gamble continue to say they’re optimistic about the technology. In November, Nestlé said it had invested in a British company that would more easily separate out polypropylene from other plastic waste.
It was “just one of the many steps we are taking on our journey to ensure our packaging doesn’t end up as waste,” the company said.
Hiroko Tabuchi covers the intersection of business and climate for The Times. She has been a journalist for more than 20 years in Tokyo and New York. More about Hiroko Tabuchi
Learn More About Climate Change
Have questions about climate change? Our F.A.Q. will tackle your climate questions, big and small .
“Buying Time,” a new series from The New York Times, looks at the risky ways humans are starting to manipulate nature to fight climate change.
Big brands like Procter & Gamble and Nestlé say a new generation of recycling plants will help them meet environmental goals, but the technology is struggling to deliver .
The Italian energy giant Eni sees future profits from collecting carbon dioxide and pumping it into natural gas fields that have been exhausted.
New satellite-based research reveals how land along the East Coast is slumping into the ocean, compounding the danger from global sea level rise . A major culprit: the overpumping of groundwater.
Did you know the ♻ symbol doesn’t mean something is actually recyclable ? Read on about how we got here, and what can be done.
Advertisement

IMAGES
VIDEO
COMMENTS
Our results show that research on the biodegradability of plastics, bioplastics, life cycle assessment, recycling of electrical and electronic equipment waste, and the use of recycled plastics in ...
Plastic materials are less recycled compared to other materials consumed in large quantities, such as glass, paper, ceramics, and aluminium [15, 16].Total plastic recycling is known as a complex technique due to its multistage processing, disposal and sorting, distribution, and use [17, 18].Plastic wastes can be recycled chemically, mechanically, or thermally (Table 1).
Scientific and effective techniques like pyrolysis can have an efficient way of plastic recycling towards the generation of fuel. The optimized physical and chemical processes are defending the merits of pyrolysis process (Sharuddin et al., 2016).There are multiple factors which affect the plastic recycling process; still recycling industries of PW face many technical challenges (MacArthur et ...
Effective recycling of mixed plastics waste is the next major challenge for the plastics recycling sector. The advantage is the ability to recycle a larger proportion of the plastic waste stream by expanding post-consumer collection of plastic packaging to cover a wider variety of materials and pack types.
Recent research points the way toward chemical recycling methods with lower energy requirements, compatibilization of mixed plastic wastes to avoid the need for sorting, and expanding recycling technologies to traditionally nonrecyclable polymers. Roughly half of the annual global production of solid plastics, or 150 million tons, is thrown ...
It is vital to recycle plastic waste, in order to conserve resources and minimize environmental impacts. Important guidelines are required for: (1) sorting, collection, transportation, and processing of plastic waste; (2) identification of different types of plastic resins and their recycling methods; (3) quality control procedures to ensure the safety and purity of recycled plastic; and (4 ...
Research into plastic recycling is rapidly increasing as ocean and land pollution and ecosystem degradation from plastic waste is becoming a serious concern. In this study, we conducted a systematic review on emerging research topics, which were selected from 35,519 studies on plastic recycling by bibliometrics analysis. Our results show that research on the biodegradability of plastics ...
Plastics have a high calorific value, with polymer energy varying from 62 to 108 MJ/kg (including feedstock energy) which is much greater than paper, wood, glass, or metals (with exception of aluminum) (Rafey and Siddiqui 2021).PW mishandling is a significant concern in developing nations like India due to its ineffective waste management collection, segregation, treatment, and disposal which ...
Plastic recycling is an important factor in the transition towards a circular economy. With recycling, countries can decouple its dependency on natural resources and work towards a more sustainable, autonomous economy. ... This Topic invites novel contributions in the form of critical reviews and research papers to address all key aspects of ...
The reported analysis enables the recommendation of closed-loop plastic recycling applications and research opportunities. The decision tree in Figure 6 is based on current technology capabilities and is designed to clarify connections between a given feedstock, its suitable recycling technique(s), and potential polymer products. The decision ...
An estimated 318 million tonnes of plastic resin is produced every year, much of it directly contributing to the 218 million tonnes of total annual solid plastic waste generation as a part of Municipal Solid Waste (year of reference: 2016). Global average plastic recycling rates are only 15%, whilst around 40% (90 million tonnes per year) is ...
The Fig. 1 represents selected recycling labels used worldwide for the packaging made of various materials. The Mobius loop composing of three arrows is the most commonly used recycling symbol in the world. This label was originally designed by Gary Anderson in 1970 as a part of a contest for the Container Corporation of America (CCA) for the description of paper recyclability.
Packaging recycling is often more economically feasible than other sectors of the plastic market due to high turnover rates of the collected post-consumer waste in Europe, 42% is recycled, 40% is sent for energy recovery and 19% is sent to landfill. [] The stability of plastics, a key performance feature that has promoted their use, also reduces their ability to degrade.
In 2019, India banned plastic waste imports. In June 2020, India introduced an extended producer responsibility framework for plastic management. There are some 100 formal and 10,000 informal plastic recycling centers in the country, with formal employment estimates of 100,000.
The topic of sustainable manufacturing of plastics and packaging is so important that, from a research on Google Scholar using as key words "sustainable plastics", "recycled plastic", and "plastic recycling techniques", a total of almost 95,000 papers were published between 2019 and 2021. This mini-review intends to give an outlook ...
This paper analyses different research approaches that employ plastic waste as binder, aggregate, fine aggregate, modifier or substitute of cement and sand in the manufacturing of bricks, tiles and concrete. ... Recycling waste plastics in developing countries: use of low-density polyethylene water sachets to form plastic bonded sand blocks ...
Up to date, primary, secondary, tertiary, and quaternary recycling methods for PSW have been developed, as shown in Fig. 2 [5].Currently, primary and secondary recycling are widely applied, due to ease of operation, which can probably obtain valuable products, but these methods can only be done with uncontaminated and single-use plastics and produce polymers which have inferior properties to ...
The main objective of the present study has been to evaluate the participants' opinion and attitudes on plastic recycling. This paper presents the results from the district of San Marcelino in the city of Valencia, the first PlastiCircle pilot to face the challenges of encouraging households to participate more in plastic waste sorting and ...
Plastic consumption is an important aspect of contemporary living, and studies that systematically examine consumers' plastic waste recycling intentions from an ethical perspective are scarce. Considering the severity of plastic waste recycling problems globally based on the stimulus-organism-response paradigm, this study analyses how the information publicity influences consumers' plastic ...
As we look toward the next year, four significant trends are poised to influence and drive the future of plastic recycling. 1. Inconsistent domestic demand for recycled content. Many U.S. companies have the same New Year's resolution: to buy and use more recycled content in their products.
A schematic representation of closed-loop recycling of a fully bio-based DGF/MBCA ERT amenable to low-temperature methanolysis. Step 1: Curing conditions: 2 equiv DGF, 1 equiv MBCA, 80°C for 2 hours, 150°C for 8 hours, 170°C for 1 hour. Step 2: Depolymerization conditions: 5 g DGF/MBCA, 40 ml methanol, 70°C for 48 hours.
Thus, advanced research is required to develop recycling and upcycling technologies for olefin-based plastics. Recycling and upcycling methods vary for each type of plastic, making it possible to recycle and upcycle plastics by mechanical and thermal means only when they are classified into analogous or homogeneous polymer groups (Cafiero et al ...
Maddie McGarvey for The New York Times. By 2025, Nestle promises not to use any plastic in its products that isn't recyclable. By that same year, L'Oreal says all of its packaging will be ...
The cost of disposing of plastic waste in developed countries is much higher than in developing countries, managing some of the plastic emissions at a cheaper cost (exporting waste plastics) and reducing pressure on recycling facilities and landfills in their own countries (Liu et al., 2018; Velis, 2014), and when oil prices are high, developed ...
Listen to article. Eriez, an Erie, Pennsylvania-based separation technologies supplier, has announced it will showcase recycling solutions at the Institute of Scrap Recycling Industries (ISRI) 2024 Convention in Las Vegas from April 15 to 18. Eriez will showcase its Eddy Current Separator and Dynamic Pulley Separator at ISRI2024 booth No. B4.
This study explored the effect of multiple recycling on the dimensional stability of wood-plastic composite. For this purpose, the injection moulding parameters effective on dimensional stability were first optimized based on the response surface method.
The research outlined in this paper shows that the basic premise of One Bin is, perhaps surprisingly, supported by both research and a broad range of stakeholders from across the supply chain. The ultimate goal of eliminating plastic leakage into the environment requires a harmonised national solution to plastic waste sorting.