Advertisement

G6PD deficiency
Concluding remarks, acknowledgments, glucose-6-phosphate dehydrogenase deficiency.

- Split-Screen
- Request Permissions
- Cite Icon Cite
- Search Site
- Open the PDF for in another window
Lucio Luzzatto , Mwashungi Ally , Rosario Notaro; Glucose-6-phosphate dehydrogenase deficiency. Blood 2020; 136 (11): 1225–1240. doi: https://doi.org/10.1182/blood.2019000944
Download citation file:
- Ris (Zotero)
- Reference Manager
Visual Abstract

Glucose 6-phosphate dehydrogenase (G6PD) deficiency is 1 of the commonest human enzymopathies, caused by inherited mutations of the X-linked gene G6PD . G6PD deficiency makes red cells highly vulnerable to oxidative damage, and therefore susceptible to hemolysis. Over 200 G6PD mutations are known: approximately one-half are polymorphic and therefore common in various populations. Some 500 million persons with any of these mutations are mostly asymptomatic throughout their lifetime; however, any of them may develop acute and sometimes very severe hemolytic anemia when triggered by ingestion of fava beans, by any of a number of drugs (for example, primaquine, rasburicase), or, more rarely, by infection. Approximately one-half of the G6PD mutations are instead sporadic: rare patients with these mutations present with chronic nonspherocytic hemolytic anemia. Almost all G6PD mutations are missense mutations, causing amino acid replacements that entail deficiency of G6PD enzyme activity: they compromise the stability of the protein, the catalytic activity is decreased, or a combination of both mechanisms occurs. Thus, genotype-phenotype correlations have been reasonably well clarified in many cases. G6PD deficiency correlates remarkably, in its geographic distribution, with past/present malaria endemicity: indeed, it is a unique example of an X-linked human polymorphism balanced through protection of heterozygotes from malaria mortality. Acute hemolytic anemia can be managed effectively provided it is promptly diagnosed. Reliable diagnostic procedures are available, with point-of-care tests becoming increasingly important where primaquine and its recently introduced analog tafenoquine are required for the elimination of malaria.
1932. Otto Warburg and Walter Christian in Berlin, Germany, identified in yeast and in red cells an enzyme of carbohydrate metabolism that oxidized glucose-6-phosphate. 1 Because the oxidation was not carried out by O 2 itself, but required as an intermediary the coenzyme NADP (then called TPN), they named the enzyme Zwischenferment : we now know it was G6PD.
1956. Paul Carson in Alf Alving’s laboratory in Chicago, IL, discovered that men who had developed AHA following administration of the antimalarial primaquine had severe deficiency of G6PD in their red cells. 2
1957. Gennaro Sansone and Giuseppe Segni in Genoa, Italy, found G6PD deficiency in all patients who had a history of favism. 3
1958. Arieh Szeinberg and colleagues in Tel Aviv, Israel, found that the inheritance of G6PD deficiency was consistent with the gene being on the X chromosome. 4
1961. Franco Panizon in Sassari, Italy, 5 and Spyros Doxiadis in Athens, Greece, 6 identified G6PD deficiency as a cause of severe neonatal jaundice.
1962. Shortly after the formulation of the “Lyon hypothesis” regarding inactivation of 1 of the 2 X chromosomes in somatic cells of female mice, Ernest Beutler at City of Hope, Duarte, CA, demonstrated that this created mosaicism in human blood cells in heterozygotes for G6PD deficiency. 7
1965. Lucio Luzzatto and Normal Allan in Ibadan, Nigeria, found that G6PD A − was structurally different from G6PD A, rather than being a lower amount of the same. 8
1966. The World Health Organization in Geneva, Switzerland, held an international Study Group in the aim to standardize methodologies for investigating G6PD deficiency and its genetic polymorphism. 9
1967. Frank Livingstone in Michigan compiled in a book hundreds of data on the frequency of G6PD deficiency in human populations. 10
1969. Philip Cohen and Michael Rosemeyer 11 show that the active form of human G6PD is either a dimer or a tetramer of identical subunits.
1980. Barbara Migeon in Baltimore, MD, maps the G6PD gene to the tip of the long arm of the X chromosome (band Xq28), near the hemophilia A gene. 12
1981. Maria Grazia Persico in Naples, Italy, clones a G6PD-related cDNA 13 ; by 1986, through a collaboration between Naples and London, we had the entire cDNA sequence and the complete structure of the G6PD genomic gene. 14
1995-2002. Embryonic stem cells in which G6PD had been knocked out by targeted homologous recombination have normal pentose synthesis but exquisite sensitivity to oxidative stress 15 ; and G6PD inactivation is lethal early in embryo development. 16
1999. Through work pioneered by Margaret Adams in Oxford, United Kingdom, 17 with coworkers in London 18 and in Hong Kong (SWN Au and Veronica Lam), the 3D structure of tetrameric human G6PD was solved by X-ray crystallography. 19 Veronica Lam sadly died in the tsunami of 2004.

Epidemiology of G6PD deficiency. The map was constructed by compiling, from the world literature, data on the population frequency of polymorphic G6PD alleles that cause enzyme deficiency. Country-specific frequency ranges, full references, and additional details are given in supplemental Table 1, available on the Blood Web site. Although frequencies may vary widely within the same country, each country was assigned here a color based on the best estimate of the average frequency of G6PD deficiency, probably the most important figure from the point of view of public health. This map represents a thorough update of one first published in 1989 and widely reproduced. 20 A similar map was published in 2009, 21 and one based on a sophisticated geostatistical model in 2012. 22
Biochemistry of G6PD and G6PD deficiency
G6PD is an enzyme very ancient in evolution, found in all organisms except for Archaea, which are mostly anaerobic, and a few obligate surface parasites (for example, Mycoplasma genitalium ) and intracellular parasites (for example, Rickettsia prowazekii ). 24 G6PD is an oxidoreductase that catalyzes the oxidation of glucose-6-phosphate to 6-phosphoglucono-lactone coupled to the reduction of NAD phosphate (NADP) to reduced NADP (NADPH) ( Figure 2 ). In all animals, G6PD is ubiquitously expressed, suggesting that it has an essential housekeeping function: indeed, knockout of the G6PD gene is lethal early in embryonic life. 16 G6PD is often referred to as the first enzyme of the pentose phosphate pathway, 28 underscoring its role in the production of pentose sugars required for nucleic acid synthesis (but pentose can also be produced through the alternative transketolase-transaldolase pathway). NADPH, produced by G6PD and called its coenzyme, is the electron donor in reactions required for the biosynthesis of deoxyribonucleotides, fatty acids, and steroids; it is also the coenzyme of cytochrome P450, central to the metabolism of many drugs and other xenobiotics. The reducing power of NADPH is required in what is commonly referred to as defense against oxidative challenges. 28

The red cell has several lines of defense against oxidative stress. Hydrogen peroxide (H 2 O 2 : in red) at the center, is a powerful oxidizing agent arising from superoxide (O 2 − ) via superoxide dismutase (SOD). O 2 − (representing in this figure, in red, ROS in general) is a by-product of auto-oxidation of Hb within the red cells, and also of the oxidative burst in neutrophils (endogenous sources, in orange). In red cells, H 2 O 2 can be detoxified to H 2 O (in green) by 3 different enzyme-mediated mechanisms. (i) Catalase directly degrades H 2 O 2 to H 2 O: it has 2 to 4 molecules of NADPH in its structure. 25 (ii) Glutathione peroxidase (GPX) catalyzes the same reaction coupled to the oxidation of reduced glutathione (GSH): it relies on the NADPH-linked glutathione reductase (GR) for regeneration of GSH. (iii) Peroxiredoxin-2 (Prx2) also degrades H 2 O 2 at the expense of its own sulhydryl groups that become disulphides, and can be regenerated by thioredoxin (Trx) through thioredoxin reductase (TrxR). 26 We do not know in quantitative terms the relative contributions of these 3 mechanisms to the degradation of H 2 O 2 in human red cells under different conditions. However, we do know that acatalasemia is not associated with hemolytic anemia, whereas GR deficiency is associated with favism, 27 supporting the importance of mechanism (ii); Prx2 deficiency in humans is not known, but it does cause hemolytic anemia in mice. Most important, all 3 mechanisms depend on NADPH, a steady supply of which can be provided in red cells only by G6PD (and 6PGD, which, however, depends on G6PD for supply of its own substrate; therefore, the crucial role of G 6 PD is highlighted in blue). Exogenous agents (in purple) can impose severe oxidative challenge by producing ROS or H 2 O 2 directly; divicine, the aglycone of vicine (together with isouramil), is the chemical responsible for favism.
There is no nucleus and therefore no DNA or RNA synthesis, no fatty acid synthesis, no endoplasmic reticulum, and therefore no cytochrome P450 29 in mature red cells: therefore, in their physiology, all of these factors are irrelevant except for the last function, which is paramount. Indeed, red cells are constantly exposed to an endogenous oxidative challenge because the concentration of hemoglobin (Hb) in red cells is no less than 5 mM. The reversible binding of O 2 to the 4 heme residues within Hb is a masterpiece of physical chemistry, but no physical device can be perfect. Spontaneous conformational fluctuations in the heme pocket of HbO 2 occasionally allow water or a small anion to enter, resulting in transfer of an electron from the iron to oxygen to produce methemoglobin and superoxide radicals: this auto-oxidation process affects 2% to 3% of total Hb each day (Low et al 30 ). Methemoglobin (Fe3+) is rapidly reduced back to Hb (Fe2+) by NADH cytochrome b5 reductase 3 (also known as methemoglobin reductase); whereas superoxide radicals, via superoxide dismutase, produce hydrogen peroxide (H 2 O 2 ), a powerful oxidizing agent. It is here that the provision of NADPH is critical: indeed, 3 enzymatic processes in the red cell can remove H 2 O 2 , and NADPH is involved in all 3 ( Figure 2 ). In red cells, G6PD is the 1 key to facing oxidative challenge.
Because mature red cells have no protein synthesis, G6PD activity physiologically decreases as red cells age. 31 Reticulocytes have a high level of G6PD activity, whereas only approximately one-tenth of that remains in the oldest red cells; however, in G6PD normal red cells, this amount is still sufficient for their needs.
Clinical manifestations
The commonest trigger of hemolysis in G6PD-deficient persons is a meal of fava beans: therefore, we briefly describe here an attack of favism ( Figure 3A ), a topic recently reviewed elsewhere. 32 The patient is usually a boy in the 2- to 10-year age group (but girls and adults are not exempt as discussed in “Genetics and molecular genetics”) who appears pale, jaundiced, and quite ill with abdominal pain and sometimes fever. On examination, the spleen may be enlarged, and the urine is usually dark (“like red wine” or “like Coca-Cola,” depending on cultural preferences). Blood examination shows moderate to very severe anemia, and a rather spectacular blood smear ( Figure 3B ). Supravital staining with methyl violet reveals Heinz bodies; there is often a neutrophil leukocytosis with shift to the left; the platelets are usually normal. The unconjugated bilirubin and lactate dehydrogrenase are elevated; haptoglobin is low or undetectable.

Clinical course of AHA in G6PD-deficient persons. (A) Attack of severe favism in a 5-year-old Sardinian boy (courtesy of Gianfranco Meloni). (B) Blood smears on day 1 of patients with favism: (i) May-Grünwald-Giemsa staining, original magnification ×1000; (ii) Heinz body supravital staining, original magnification ×1000. (C) Time course of Hb levels in a large cohort of children with P falciparum malaria who received antimalarial treatment with a drug combination (Lapdap) containing dapsone. Eleven percent of G6PD-deficient hemizygous boys and 0.5% of heterozygous girls required blood transfusion. All of these children were in clinical trials under appropriate medical supervision; there were no deaths (there might have been outside of clinical trials). B, bite cell; nc, nucleated red cell; S, spherocyte. Modified from (A) Luzzatto and Arese, 32 (B) Luzzatto and Poggi, 63 and (C) Pamba et al 33 with permission.
Since the discovery of G6PD deficiency the standard spectrophotometric assay of G6PD enzyme activity (Box 1), used on hemolysates, has provided a highly reliable diagnostic test. 40
Brewer 41 observed that after Hb (Fe 2+ ) in intact red cells is converted to methemoglobin (Fe 3+ ) by the addition of sodium nitrite, reversal to Hb (Fe 2+ ) depends on the G6PD activity within the red cells themselves: G6PD normal samples will return to bright red, whereas G6PD-deficient samples will stay brown.
Motulsky and Campbell-Kraut 42 observed that the time for brilliant cresyl blue (BCB) added to hemolysates to become colorless was roughly proportional to G6PD activity.
Both the BCB and the methemoglobin reduction tests lent themselves to processing large numbers of samples: they were the first G6PD screening tests, and they were widely used, for instance, to determine the frequency of G6PD deficiency in various human populations (Livingstone 10 ).
Based on the screening method carried out on intact red cells (no. 2 in this list), a cytochemical test was developed in 1965. 43 Under certain precise conditions methemoglobin is eluted from red cells but Hb is not: thus, after smearing red cells on a slide, elution and staining, G6PD deficiency can be quantitatively assessed by a differential count of G6PD normal and G6PD-deficient red cells.
From 1966 to 1968, Beutler’s laboratory developed a “spot test” for the diagnosis of G6PD deficiency that could be carried out by just applying to a piece of filter paper 1 reagent mixture and 10 μL of blood: after 15 minutes the spot will show bright fluorescence only if the sample is G6PD normal. 44 This fluorescent spot test (FST) became popular not only for work in the field but also in diagnostic laboratories because it is reliable, inexpensive, and easy (the only equipment required is a UV lamp).
A new cytochemical test, based on the use of tetrazolium dyes, was introduced in 1968 by Fairbanks and Lampe 45 in 1968; subsequently the test was perfected by Van Noorden. 46,47
Both cytochemical methods (nos. 5 and 7 in this list) have been used particularly in testing women heterozygous for G6PD deficiency, who have therefore a mosaic composition of blood cells; the method mentioned under item 7 has proven to be technically easier.
Although screening methods were already available [nos. 2, 3, and 6 in this list], the resurgence of primaquine and the introduction of tafenoquine, 48 for the administration of which G6PD testing is label-mandatory, have been a strong stimulus to the development of point of care (POC) tests. These are becoming available as commercial kits: some are suitable for identifying not only G6PD-deficient hemizygotes and homozygotes, but also heterozygous females at risk of AHA. 49
Given that many G6PD mutations are known ( Table 2 ; Figure 5 ), a G6PD-deficiency status can be inferred reliably by mutation analysis (genotypic testing). This approach has the advantage that it can be carried out on stored DNA, and it circumvents the risk of misclassification that arises when reticulocytosis produces in a G6PD-deficient person an increase in G6PD enzyme activity that may, though rarely, be so marked as to reach the normal range. However, there are 2 caveats: (a) one needs to know which mutations are prevalent in the population to which the patient belongs; and one may still miss an unusual or unknown mutation; (b) in heterozygous females, on account of the wide range of the ratio between G6PD normal and G6PD-deficient red cells generated by X-inactivation, the genotype does not predict the degree of G6PD deficiency: from this point of view, in heterozygotes, a phenotypic test is superior to a genotypic test.
Drugs that may trigger AHA in G6PD-deficient patients
Modified from Table 4 of Luzzatto et al, 38 where sources and additional references are given.
Evidence-based according to Youngster et al. 39
Acetylsalicylic acid. Like for all drugs, hemolysis is dose-related. The regimen of 75 to 100 mg per day, widely used for the prophylaxis of cardiovascular events, is safe for G6PD-deficient persons.
Methylene blue.
Toluidine blue.
Furadantin.
Infection is the third potential trigger of AHA, but it is more erratic. Broadly speaking, bacterial infection must be severe to cause AHA: it has been reported with pneumonia, 50 brucellosis, 51 rickettsiosis, 52 maxillary abscesses caused by Streptococcus , 53 or Staphylococcus , 54 and even with Clostridium difficile 55 infection. AHA also occurs in the course of hepatitis, 56 including hepatitis A, B, 57 and E. 58,59 With viral hepatitis in G6PD-deficient patients, hyperbilirubinemia is sometimes excessive even when there is little or no evidence of AHA, 56-60 and acute renal failure can be a further complication. 60 AHA has also been reported occasionally in the course of cytomegalovirus infection 61 and in 1 instance of dengue fever. 62 AHA triggered by infection can be quite severe: if it subsides when the infection is controlled, then we can be confident that infection was the trigger. In some cases, AHA has been mistakenly blamed, rather than on the infection, on an antibiotic used to treat it; but the reverse could also happen.
With both fava beans and drugs, G6PD deficiency-related hemolysis is characteristically dose-dependent: the cases that come to the emergency room are the “tip of the iceberg.” When the anemia is mild, the patient may not be seen in hospital and may often be undiagnosed; in many cases, there may not even be anemia, but only compensated hemolysis.
Diagnosis and management
If AHA is severe, blood transfusion may be urgent 63 ; otherwise, only fluid support and analgesics may be needed. In most cases, the patient had been previously asymptomatic: AHA will prompt testing for G6PD deficiency, which should be done by a quantitative test ( Box 2 ). In most cases, the result will be clear cut, that is, below the normal range of 7 to 11 IU/g Hb. 64 Reference ranges vary, and unfortunately those stated by some laboratories are too wide. A result below 80% of the lower limit of normal must be regarded as G6PD deficient. Sometimes, during or immediately after a hemolytic attack, G6PD activity from a G6PD-deficient patient may be within the “normal range” (as a result of both destruction of the oldest cells and reticulocytosis). In such cases, the test must be repeated after a few weeks, or a known mutation can be revealed by DNA testing.
In areas with high prevalence, donor blood may be G6PD deficient: in general, this can be used safely 65 and universal screening of donors is not practical. Ideally, it would of course be preferable to use G6PD normal blood when needed for AHA in a G6PD-deficient patient, when doing an exchange transfusion for neonatal jaundice (NNJ; see “NNJ” later in text), or in patients requiring regular blood transfusion.
Clinical course
Unlike with autoimmune AHA, which often poses considerable therapeutic challenges, with G6PD-related AHA, rapid recovery is the rule. A most striking feature is the speed of the reticulocyte response ( Figure 3A ). It is hard to imagine that a hypoxia-erythropoietin–mediated response can take place within 48 hours: there must be a rush order (mechanism not yet known) that prompts the exiting from the marrow of existing reticulocytes.
Pathophysiology
This type of AHA seems almost like experimental hematology (reminiscent of a phenylhydrazine-treated rabbit). 66 The zero time is exposure to the trigger; from then on, we witness what happens when, in a person with a previously normal blood count, up to two-thirds of red cells are suddenly destroyed by a process referred to as oxidative damage. The culprit is a chemical (primaquine, or any of the other drugs listed in Table 1 , or divicine and isouramil from fava beans) that can generate reactive oxygen radicals (ROS) and H 2 O 2 ( Figure 2 ): these can also be produced directly in the course of bacterial infection. As noted in the previous section, ROS are generated all the time through auto-oxidation of Hb; however, the rate at which they are thus produced is low, so that even G6PD-deficient red cells can provide enough NADPH to cope with them. When the levels of ROS are instead higher, red cells with normal G6PD respond by recycling NADPH at an increased rate, thereby preventing damage. G6PD-deficient red cells are unable to do so, which results in formation of ferryl hemoglobin and of hemichromes (partially denatured hemoglobin); at the same time, lipids and thiol groups in cytoplasmic and membrane proteins are oxidized. Binding of hemichromes to the membrane cytoskeleton leads to formation of Heinz bodies; aggregation of membrane proteins leads to formation of cross-bonded rigid hemighosts. 32,67 Glutathione (GSH) plays a special role in defense against oxidative damage because, in addition to being the substrate of GSH peroxidase, it can also regenerate thiol groups in proteins that had been oxidized to disulphides. 68 The more severely damaged red cells will undergo intravascular hemolysis, with consequent hemoglobinuria. In other red cells, clusters of oxidized band 3 in the membrane will bind immunoglobulin G and complement factor C3c 69 ; thus, opsonized, they will undergo erythrophagocytosis, that is, extravascular hemolysis. Intravascular hemolysis accounts, of course, for hemoglobinuria, and also for abdominal pain, because plasma Hb will bind nitric oxide causing smooth muscle dystonia; extravascular hemolysis accounts for jaundice and for splenomegaly. In G6PD-deficient red cells, like in normal red cells, there is a downward gradient in G6PD activity as they age 70 : therefore, the oldest red cells will be the first to suffer damage; whereas the youngest red cells, including reticulocytes, are relatively resistant to hemolysis.
Chronic nonspherocytic hemolytic anemia
This rather cumbersome heading originates from history. Hereditary spherocytosis (HS) has been, for over a century, a prototype of congenital hemolytic anemias (other than hemoglobinopathies), but in some patients with this kind of condition, spherocytes may not be prominent on a blood smear: after G6PD deficiency was discovered, some of them were found to be G6PD deficient.
In contrast to the high prevalence of G6PD deficiency that entails the risk of AHA, chronic nonspherocytic hemolytic anemia (CNSHA) is a rare disease (estimated frequency, <10 per million). The clinical picture is generally similar to that of HS, including jaundice and gallstones, with wide-ranging severity (see examples in Table 2 ): from mild (for example, diagnosed incidentally in an adult) to severe enough (in a minority of patients) to require recurrent blood transfusion. Unlike in HS, a history of NNJ (see next section), often severe, is the rule. Needless to say, all agents capable of causing AHA in any G6PD-deficient person will cause acute on chronic hemolysis in patients with CNSHA. In some transfusion-dependent patients, splenectomy has proven highly beneficial ( Figure 4 ).
Different G6PD mutations produce a wide range of biochemical phenotypes
From the full list (supplemental Table 2) of G6PD variants we have selected, among those for which enzymatic properties are known more extensively, some polymorphic (classes II-IV) and some rare (class I) variants. These properties were reported either in Betke et al 9 or when the variant was originally described (references in supplemental Table 2), or in subsequent papers (references in this table). Class I variants are, by definition, associated with CNSHA: but within this diagnosis there is a wide spectrum of severity.
—, no change; 3D, 3-dimensional; BT, blood transfusion; CNSHA, chronic nonspherocytic hemolytic anemia; exp, expected.
Thermostability studies must be carried out on purified or recombinant enzyme, and they have been carried out in different ways, making comparisons problematic: hence, we have adopted in this table a semiquantitative terminology. Rather than the time it takes to inactivate the enzyme at a fixed temperature, it is probably more informative to determine T 1/2 (temperature at which 50% activity is lost after a fixed exposure time, eg, 7 minutes): this parameter was first introduced in 1965 8 and found to be highly sensitive to the concentration of NADP. The properties of this arbitrary set of variants illustrate the following: (1) Almost all class I variants show evidence of markedly impaired stability; several of the others are also unstable, and in some this has been found to correlate with accelerated decay in vivo; (2) K m G6P , when increased, affects performance in the steady state; therefore, it is not surprising to find this feature in some class I variants (for example, Portici, Volendam). G6PD Orissa is not in class I (despite high KmG6P) probably thanks to its relatively high residual activity; (3) To determine k cat , pure enzyme (usually obtained by recombinant DNA technology) is required (hence some data are missing): it is moderately decreased in many variants; drastically decreased in G6PD Nilgiri and G6PD Santiago; (4) There is overlap in residual enzyme activity values between class II and class I; however, as a rule, all class I variants have very low activity (1% or less: sometimes undetectable);(5) Comparing variants where the same amino acid is replaced: G6PD Plymouth (class I) differs from G6PD Mahidol (class II) only in reduced thermostability; interestingly, the amino acid replacement in G6PD Plymouth entails a change in charge, whereas that in G6PD Mahidol does not. G6PD Santiago (class I) and G6PD Nilgiri (class II) again differ only in thermostability; and again there is a charge change in the former but not in the latter.
Calculated from ratio of activity/cross reacting material compared with G6PD B.
Enzyme activity reported as undetectable.

Clinical course of a patient with CNSHA. The patient presented with severe NNJ requiring exchange blood transfusion; he then had moderate to severe anemia requiring frequent blood tranfusions (arrows at the top). At 9 years of age, he was splenectomized, whereupon he became transfusion-independent. Note the persistent reticulocytosis, that remitted after splenectomy. The underlying unique variant was G6PD Harilaou (class I: F216L). Reproduced from Luzzatto and Poggi 63 with permission.
The clinical picture of CNSHA is so different from asymptomatic G6PD deficiency that it prompted early on 9 a classification of underlying variants ( Table 3 ). It is now clear that different mutations underlie these 2 phenotypes, as discussed later in text.
Molecular basis of G6PD variants
Details and references regarding all 230 G6PD protein variants with known mutation are given in supplemental Table 2.
According to a classification proposed in 1971 87 and universally used since, class IV variants have normal activity; class III variants have enzyme deficiency with residual activity 10% to 60%; class II variants have enzyme deficiency with residual activity <10%; class I variants are those that cause CNSHA. We prefer to consider class II and class III variants as 1 group 38 because their clinical manifestations are the same: even though, when they occur, they tend to be more severe, on average and with wide overlap, with class II variants than with class III variants. In view of this, the WHO is currently considering a revision of this classification.
There is 1 example of 2 different mutations resulting in the same amino acid change (F173L); there is 1 example of 2 contiguous base replacements within the same codon (G6PD Palermo); in 2 variants, 2 mutations occur in the same codon; in 1 case, this is the only mutation; in another case, it is associated with another 2 mutations in the nearby codons (G6PD Crispim). Two variants (G6PD Tokyo Glu416Lys, G6PD Herlev Arg198Ser) are reported as class I/II.
Until recently, the only class IV variant was G6PD A (originally characterized because electrophoretically fast compared with the “wild-type” G6PD B). Interestingly, 4 additional class IV variants have been recently discovered in a normal subjects database 81 ).
Two variants with 3-aa replacements
One variant with 3-aa replacements
Fifteen variants with 2 and 3 variants with 3-aa replacements. Only 8 of the variants with multiple amino acid replacements carry an amino acid replacement not found as a single amino acid replacement: these specific amino acid replacements are 11.
Two variants with splicing site mutation and 1 with a nonsense mutation.
In newborns, G6PD deficiency entails the risk of NNJ ( Box 1 ). The vast pertinent literature (see Luzzatto and Poggi 63 and Kaplan and Hammerman 88 ) cannot be fully reviewed here, but some facts must be noted. First, NNJ in G6PD-deficient babies can be severe enough to cause kernicterus, therefore, prompt management, which may require exchange blood transfusion, is imperative if permanent neurologic damage is to be avoided. Second, unlike with severe NNJ caused, for example, by Rhesus incompatibility, in most cases there is little if any evidence of hemolysis: indeed, we have to admit that the mechanism is not yet clear. 89 Third, although not all G6PD-deficient babies develop NNJ, in numerous studies from different parts of the world (for example, in Africa), the frequency of NNJ has been always higher in G6PD-deficient babies compared with G6PD normal controls. 90,91 The coexistence of a UGT1A1 promoter mutation further increases this frequency. Fourth, the peak incidence of hyperbilirubinemia is on day 2 to day 3 after birth: this is of great practical importance because mother and baby may have been discharged already, and therefore there may be a delay or even a failure of a diagnosis that requires urgent management by phototherapy or exchange blood transfusion.
Genetics and molecular genetics
The G6PD gene (which maps to Xq28) has 13 exons (exon 1 being noncoding: Figure 5 ), and it encodes a polypeptide chain of 514 amino acids, the dimer and the tetramer of which ( Figure 6 ) are the enzymatically active forms of G6PD (the monomer has no enzyme activity).

Known mutations in the G6PD gene. The full list of known mutations in the G6PD gene can be found in supplemental Table 2. The G6PD genomic gene spans ∼18 kb. Exons are shown as thick numbered blocks and introns as thin lines. Exon 1 (noncoding) and the 3′ untranslated region of exon 13 are in gray. The long intron 2 is indicated by a diagonal double line. Mutations with normal enzyme activity (class IV) are in green. Mutations that cause susceptibility to AHA (class II/III) are in blue. Mutations that cause CNSHA (class I) are in red. Mutations that have been observed only in association with another mutation are in purple. Mutations with unknown clinical features are in in black italics. The amino acid replacements caused by mutations are shown using the amino acid 1-letter symbols. Note the predominance of class I variants in exons 10 and 13. Additional notes: (a) This variant has, in addition to the mutation shown, also the mutation (454 Arg>Cys) of G6PD Union. (b) These variants have, in addition to the mutation shown, also the mutation (126 Asn>Asp) of G6PD A. (c) This variant has been reported to have 3 different mutations: 2 are unique (106 Ser>Lys and 182 Arg>Trp), whereas 1 is the mutation (198 Arg>Cys) of G6PD Coimbra. (d) This variant has been reported to have 3 different mutations: 2 are unique (125 Met>Ile and 127 Ala>Pro), whereas 1 is the mutation (128 Leu>Pro) of G6PD Vanua Lava. (f) This mutation has been found also associated with the mutation (126 Asn>Asp) of G6PD A. (g) These variants have, in addition to the mutation shown, also the mutation (291 Val>Met) of G6PD Viachang. (h) This variant is generated by 2 mutations within the same codon. (i) This variant has 2 unique mutations: 213 Val>Leu and 346 Asn>Ile. (j) This variant has 2 point mutations within the same codon. Δ indicates deletion. ∫, abnormal splicing; Ins, insertion.

3-Dimensional structure of the human G6PD protein. Both the dimer and the tetramer are enzymatically active. The dimer/tetramer ratio within different cells or under different conditions is still not known, but it affects the pH-dependence of G6P binding. 92 It is still not known what proportion of G6PD, within cells, is in dimer form. (A) The G6PD dimer, showing the binding sites of the 2 substrates G6P (yellow) and NADP (purple), and the site of the “structural” NADP (blue) (from Gómez-Manzo et al 78 ). (B) The G6PD tetramer, showing the positions of a small selection of G6PD variants. Note (on top) the relatively large in-frame deletion of G6PD Nara: this is compatible with residual G6PD activity because the 8 aa involved are in a nonstructured region. Modified from Mason et al 93 with permission.
The consequences of X-linkage are very important. First, because a male has only 1 G6PD allele, he can only be either hemizygous G6PD normal or hemizygous G6PD deficient; a female instead, having 2 G6PD alleles, can be homozygous G6PD normal, or homozygous G6PD deficient, or heterozygous for G6PD deficiency. Second, in keeping with the laws of population genetics, in any given population, heterozygotes are more frequent than hemizygous G6PD-deficient males, whereas homozygous G6PD-deficient females are much more rare (see examples in Luzzatto et al 38 ). Third, because G6PD , like most X-linked genes, is subject to the phenomenon of X-chromosome inactivation ( Box 1 ), heterozygous females are epigenetic mosaics: in their blood, there is a mixture of G6PD normal and G6PD-deficient red cells. The ratio between the 2 types has a modal value of 1 (ie, 50% of each type), but this ratio is highly variable from 1 person to another: therefore, the phenotype of heterozygous females ranges from G6PD normal to G6PD, just as deficient as that of a hemizygous male. Some of these facts are sometimes misunderstood. At the population level, many studies report the frequency of G6PD deficiency in a sex-pooled population sample. This is unfortunate because only a subset of G6PD-deficient heterozygous females will have been classified as G6PD deficient, and therefore, in that population, we will not know the true frequency of the G6PD-deficient allele(s): instead, this could have been exactly ascertained from the frequency of G6PD-deficient males alone, which is identical to the frequency of the respective allele. It is also often stated that G6PD deficiency “is more frequent in males,” or “more expressed in males”: both statements are not correct. It is true, instead, that whereas G6PD deficiency is regularly expressed in G6PD-deficient hemizygous males and homozygous females, in heterozygous females, the expression, and therefore the potential severity of hemolysis, is highly variable. Surprisingly, G6PD deficiency is often qualified, even in textbooks, as “X-linked recessive”: of course this is wrong because G6PD deficiency is frequently expressed in heterozygotes, both biochemically and clinically.
There are now 230 G6PD variants with known mutations ( Table 3 ; Figure 5 ; supplemental Table 2). All but 2 of them are either missense mutations (each 1 causing a single amino acid replacement) or small in-frame deletions (causing loss of 1 or a few amino acids): these will produce a qualitatively abnormal G6PD protein, which still has some residual enzyme activity. Frame-shift mutations, which would result in zero enzyme activity, are conspicuously absent; and the only nonsense mutation (G6PD Georgia; supplemental Table 2), which would do the same, has been found in a heterozygote. These data support the notion that complete loss of G6PD activity is lethal. Some variants have 2 mutations in cis : the best known is G6PD A - , in which a mutation in codon 126 (N126D, not causing G6PD deficiency), coexists with 1 of 3 other mutations (M68V, R227L, L323P). It is the combination of the 2 mutations that causes G6PD deficiency. 94 Note: the term variant is currently often used to designate proteins resulting from mutations that may or may not have pathological implications. Algorithms predicting pathogenicity of variants have become popular, but, in the case of G6PD, the finding of enzyme deficiency is the best test because every enzyme-deficient variant has the potential to cause clinical manifestation.
Genotype-phenotype correlations
Given the large number of point mutations identified within the G6PD gene, we should try to understand why and how they give a particular phenotype. First, we must consider what will cause G6PD enzyme activity to be deficient. A low rate of G6PD protein synthesis is unlikely, as no regulatory mutations have been found. In principle, we might be dealing therefore with decreased stability or decreased catalytic activity ( k cat ). Either or both occur in different cases (see examples in Table 2 ), but decreased stability (that may imply impaired folding or impaired stability of dimer or tetramer) is more prominent in more cases; this is not surprising, in view of the long lifespan of red cells, whereby the physiological decrease in G6PD activity as red cells age is greatly accelerated. If instability is moderate, there will be enough G6PD for the steady-state requirements of red cells, and these will fail (ie, undergo hemolysis) only when an exogenous oxidative stress is applied. If instability is severe, G6PD activity will be insufficient to enable normal red cell survival even in the absence of oxidative stress: this will give the CNSHA (class I) phenotype.
From the evolutionary point of view, G6PD-deficient variants are enriched in amino acid residues of intermediate conservation (supplemental Figure 1 and Notaro et al 24 ). We presume that mutations in more highly conserved residues are more likely to be lethal, and mutations in nonconserved residues are less likely to cause G6PD deficiency. The next question is why, once a mutation gives G6PD deficiency, the phenotype is mild (class II or III; Table 3 ) or severe (class I; CNSHA). The most obvious factor must be the level of residual G6PD activity: in this respect, there is a striking density of class I mutations in exon 10 ( Figure 5 ), which comprises many of the amino acids involved in the dimer interface (failure of dimerization entails the maximum of instability); amino acid replacements near the structural NADP site ( Figure 6A ) or the dimer-dimer interface 95 may also affect stability. Another factor is substrate affinity, because the intraerythrocytic concentrations of G6P and NADP are much below saturation 96 . Therefore, a high K m G6P will be generally an adverse feature ( Table 2 ), whereas a low K m G6P will be an advantage in the steady state (less so under oxidative stress: it might be the reason why G6PD Mediterranean, for instance, is in class II rather than in class I). The relative roles of k cat and of enzyme instability in G6PD variants have been recently explored 78 and subjected to rigorous principal component analysis. 81
The regulation of G6PD transcription has been investigated in some detail. The G6PD core promoter region includes 7 guanine-cytosine (GC) boxes, 2 of which, through binding of the Sp1 and AP-2 transcription factors, are essential. 97 G6PD expression requires a specific chromatin conformation, as histone deacetylase inhibitors, through enhanced recruitment of Sp1 and of RNA polymerase II, enhance G6PD transcription. 98
Animal models of G6PD deficiency have been reviewed elsewhere. 38 Although models exist in mouse and in zebrafish, they have not yet been adopted for routine safety testing of new drugs.
Correction of G6PD deficiency
By transduction of hematopoietic stem cells with a retroviral vector harboring the human G6PD complementary DNA (cDNA), stable expression of human G6PD was obtained in primary and secondary recipient syngeneic mice. 99 A similar vector was also competent for human G6PD expression in Macaque monkeys. 100 In principle, severe CNSHA due to G6PD deficiency could be treated by allogeneic bone marrow transplantation or by gene therapy, but this has not yet been attempted.
In view of the fact that histone deacetylase inhibitors selectively increase the synthesis of G6PD (but not of 16 other red cell enzymes that have been tested 98 ), butyrate or valproate might correct G6PD deficiency, but chronic administration would be required (or, in theory, one might contemplate using 1 of these agents to prevent or curb hemolysis under special circumstances). Very recently, high-throughput screening has been used to identify a small molecule, AG1 (2,2′-disulfanedylbis- N -(2-(1 H -indol-3-yl)ethyl)ethan-1-amine), which is able to activate G6PD 101 . Although the activation is less than twofold, AG1 might be a lead compound for finding more effective activators.
G6PD deficiency and malaria
1960. Based on the close geographic correlation between the frequency of G6PD deficiency and the endemicity of P falciparum malaria, Allison 102 and Motulsky 103 independently suggested that the latter had been a factor in Darwinian selection of the former.
1966. By micromapping the frequency of G6PD deficiency within the island of Siniscalco et al 104 found a direct correlation with former malaria prevalence (by that time malaria had been eradicated in that island).
1967. By studying children with high P falciparum parasitemia in Nigeria Gilles et al 105 provided evidence that G6PD deficiency may be protective against severe malaria.
1969. In patients with P falciparum malaria it is found by cytochemical analysis that parasites are present preferentially, within the same patient, in G6PD normal vs G6PD-deficient red cells. 106
1972. In a study of 699 children admitted to hospital with fever it was found that the distribution of parasitemia was significantly shifted to lower levels in girls heterozygous for G6PD deficiency but not in G6PD-deficient males. 107
1983-98. From in vitro cultures of P falciparum it was initially reported that parasite growth was decreased in G6PD-deficient host red cells compared with G6PD normal host red cells 108 ; later it was found that under optimal conditions the growth was very similar. However, when exposed to autologous monocytes, P falciparum– infected red cells were phagocytosed at a much earlier stage of parasite development when they were G6PD-deficient than when they were G6PD normal. 109
1994. Identification and cloning of the G6PD gene from P falciparum . 110 This gene has sequence homology to all other known G6PD genes from other organisms, 24 but it is unique by being some 300 codons longer. It was subsequently discovered that this longer gene encodes a bifunctional enzyme that has not only G6PD activity but also the metabolically closely related 6-phosphoglucono-lactonase activity. 111
1995. From the combined data of a study carried out in children in Gambia and in Kenya, Ruwende et al 112 reported protection against severe malaria of both G6PD-deficient males and females heterozygous for G6PD deficiency. This paper had a huge impact in this field (over 600 citations): however, years later it was invalidated by the finding that the DNA methodology used for G6PD typing must have misclassified a large proportion of the samples from the Gambia. 113,114
2013-2018. Several studies have confirmed the original finding 107 that protection against severe malaria (a proxy for malaria mortality) is a prerogative of heterozygotes. Considering different forms of severe malaria, protection may be greater against severe malarial anemia than against cerebral malaria.
Darwinian selection is not the only link between malaria and G6PD deficiency, as the latter was originally discovered through the study of AHA caused by exposure to primaquine ( Table 1 ), the only drug, until recently, that effectively eliminates the dormant liver forms (hypnozoites) of Plasmodium vivax . This requires a 14 day-course of primaquine (0.75 mg/kg per day), which will regularly cause hemolysis in a G6PD-deficient person, including the majority of heterozygous females. A much lower dose (0.25 mg/kg once only) is recommended to eliminate gametocytes (after a P falciparum malaria attack has been treated with a standard course of an artemisinin combination): this is safe for G6PD-deficient persons. 121 The primaquine analog tafenoquine ( Table 1 ) also causes AHA in G6PD-deficient persons 122 : we may surmise that damage to the parasite and damage to G6PD-deficient red cells go hand in hand because they are both mediated by the ability of these 4-aminoquinolines to produce ROS. Tafenoquine is attractive because, due to its longer in vivo half-life, a single dose is sufficient; however, this becomes a liability in a G6PD-deficient person, as the drug cannot be discontinued if and when signs and symptoms of AHA develop. In view of this, tafenoquine 123 has been licensed with a label that positively prescribes testing for G6PD deficiency: this in turn has been a strong stimulus to the development of simple and reliable point-of-care tests for G6PD deficiency, including heterozygous females ( Box 2 ).
Extraerythrocytic manifestations of G6PD deficiency
Because G6PD is ubiquitously expressed, one might expect manifestations of G6PD deficiency not to be limited to red cells, but rather to occur in other cells or tissues as well. Overall, these are not very prominent, mainly because, as stated herein, in most cases the molecular basis of G6PD deficiency is enzyme instability, and presumably nucleated cells can compensate for instability, if need be, through increased G6PD synthesis. The consequence of G6PD deficiency in granulocytes has been best characterized in some of the patients who have CNSHA (for example, G6PD Barcelona 124 ), who suffered severe bacterial infections. The likely explanation is that granulocytes with severe G6PD deficiency are unable to produce enough NADPH to provide an effective oxidative burst (so much so that, a long time ago, G6PD deficiency had been confused with X-linked chronic granulomatous disease). A possibly related finding is the increased frequency of severe sepsis in G6PD-deficient patients after major trauma 125 : interestingly, this was observed in patients with the common variant G6PD A − . There have been isolated case reports in G6PD-deficient persons of acute rhabdomyolysis with myoglobinuria and sometimes acute renal failure. 126 This is a very rare occurrence when compared with AHA, with which it is usually but not always associated. 127
The cells of the eye lens share with red cells loss of organelles, including the nucleus. There has been controversy as to whether G6PD deficiency favors cataract formation, but this does not seem to be the case. 128 There is extensive literature on G6PD and cancer, and the results have not been uniform. A recent study has found that adenomatous polyps and colon cancer are less frequent in persons with G6PD deficiency/ 129 we think it is preferable to await confirmation before speculating on how G6PD deficiency might be protective. The risk of cardiovascular disease has been reported to be somewhat higher (odds ratio, 1.39; confidence interval, 1.04-1.87) in G6PD-deficient males compared with controls. 130
G6PD deficiency is not a disease (except for the very small minority of patients who have CNSHA): it is a widespread genetic trait that can protect heterozygotes from dying of malaria. Yet, AHA in a G6PD-deficient child or adult is a medical emergency that, if not promptly and appropriately treated, can be fatal. On a worldwide basis, the commonest trigger of AHA is the ingestion of fava beans: favism is seen in at least 35 countries, and there are probably thousands of cases every year. 32 The next common trigger, in the same and in other countries, is iatrogenic: deaths have been reported with both primaquine 131 and rasburicase, 132 and these deaths are preventable. Favism is preventable by population screening and health education 133 ; possibly also by the introduction of fava bean cultivars with absent or very low levels of vicine and convicine. 134
As hematologists, we have learnt much from G6PD-deficient patients. At the same time, the biology of G6PD is eminently interdisciplinary, having been a model system in biochemical genetics and in understanding how the red cell responds to oxidative attack; a tool for studying X-chromosome inactivation (the most spectacular epigenetic event in human development); a tool, for years, for studying clonal populations; a pioneer in the molecular genetics of enzymopathies; and the best characterized example in humans of an X-linked genetic polymorphism balanced by Darwinian selection exerted by malaria.
The online version of this article contains a data supplement.
The authors thank all of their collaborators in Ibadan, Naples, London, New York, Genova, Firenze, Bangkok, Ouagadougou, Dar es Salaam, and elsewhere, and particularly José Bautista for careful review of the manuscript.
This paper is dedicated to the memory of Olaniyi Babalola and Giorgio Battistuzzi, who did early studies on the biochemistry and genetics of G6PD variants, and of Graziella Persico, who cloned the human G6PD gene.
Contribution: All authors contributed to writing the paper and to assembling the large amount of supplementary materials.
Conflict-of-interest disclosure: The authors declare no competing financial interests.
Correspondence: Lucio Luzzatto, Hematology, Muhimbili University of Health and Allied Sciences, PO Box 65001, Dar es Salaam, United Republic of Tanzania; e-mail: [email protected] .
Supplemental data
This feature is available to subscribers only.
- Previous Article
- Next Article
Email alerts
Affiliations.
- Current Issue
- First edition
- Collections
- Submit to Blood
- About Blood
- Subscriptions
- Public Access
- Permissions
- Blood Classifieds
- Advertising in Blood
- Terms and Conditions
American Society of Hematology
- 2021 L Street NW, Suite 900
- Washington, DC 20036
- TEL +1 202-776-0544
- FAX +1 202-776-0545
ASH Publications
- Blood Advances
- Hematology, ASH Education Program
- ASH Clinical News
- The Hematologist
- Publications
- Privacy Policy
- Cookie Policy
- Terms of Use
This Feature Is Available To Subscribers Only
Sign In or Create an Account
ISSN: 0041-4301 Online ISSN: 2791-6421
The Turkish Journal of Pediatrics
- About the journal
- Author Guidelines
- Review Process
- Publication Ethics
- Privacy statement
- Editorial Board
- Advisory Board
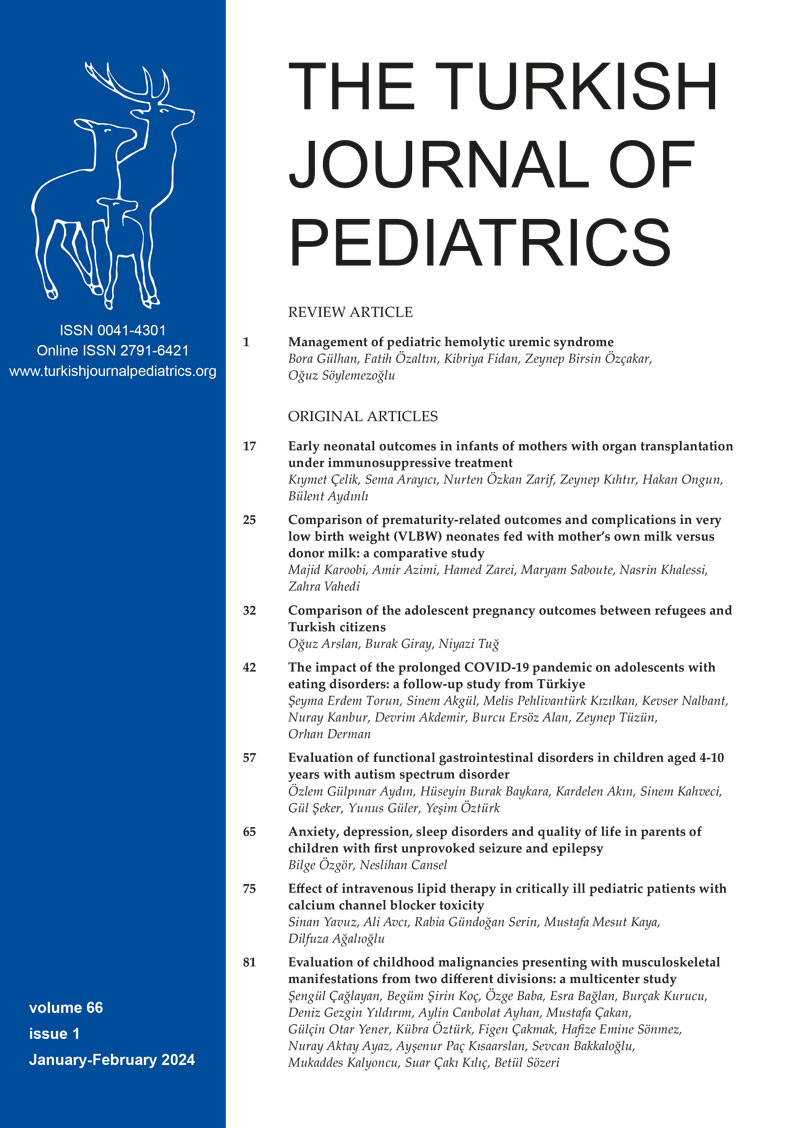
Volume 66 Issue 1 (2024)
Review article, original articles, short communications, case reports, letters to the editor.
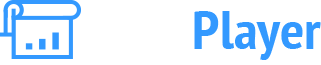
- My presentations
Auth with social network:
Download presentation
We think you have liked this presentation. If you wish to download it, please recommend it to your friends in any social system. Share buttons are a little bit lower. Thank you!
Presentation is loading. Please wait.
Case Study 1: G6PD David Grkovicious Andrew Wattals Tim Cryal.
Published by Holly Owens Modified over 8 years ago
Similar presentations
Presentation on theme: "Case Study 1: G6PD David Grkovicious Andrew Wattals Tim Cryal."— Presentation transcript:
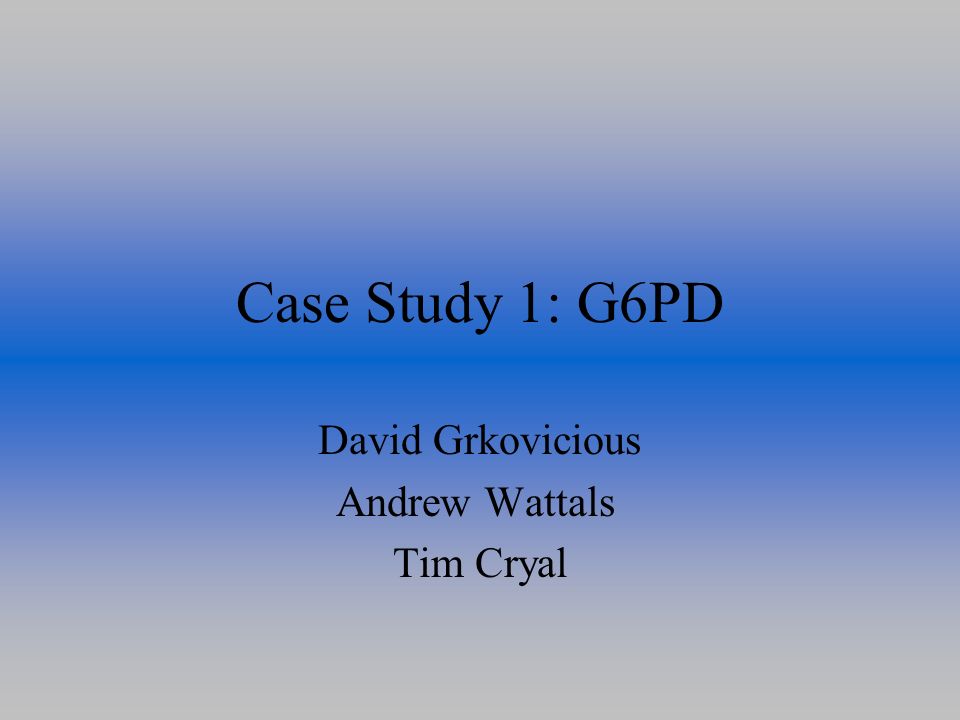
Practical Hematology Lab Glucose 6 Phosphate Dehydrogenase
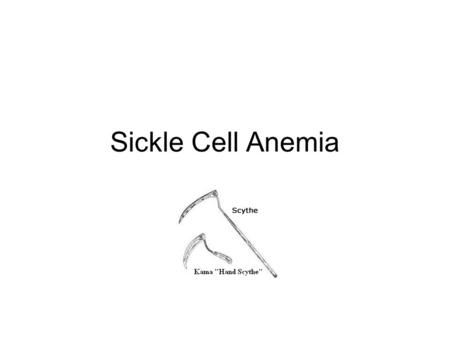
Sickle Cell Anemia. Sickle cell anemia - abnormally shaped red blood cells that are shaped like a sickle (or crescent). Hard and sticky, sickle cells.
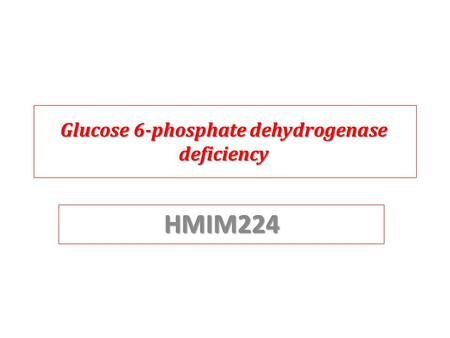
Glucose 6-phosphate dehydrogenase deficiency
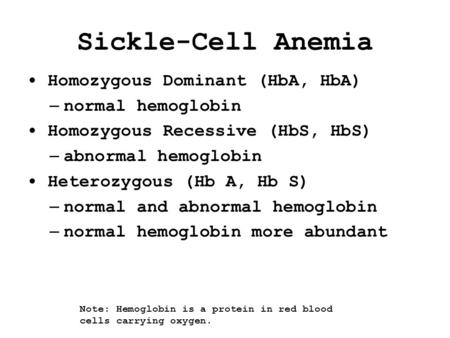
Sickle-Cell Anemia Homozygous Dominant (HbA, HbA) – normal hemoglobin Homozygous Recessive (HbS, HbS) – abnormal hemoglobin Heterozygous (Hb A, Hb S) –
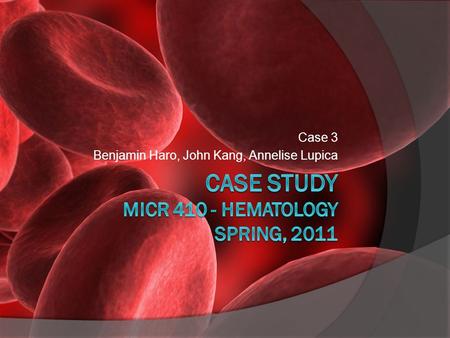
Case Study MICR Hematology Spring, 2011
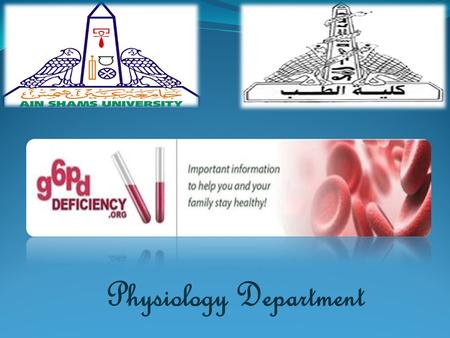
Physiology Department Esraa Raafat Ahmed Ghanem 92 - Esraa Reda Hashem Tawfik 93 -Esraa samy Farid Abd Elghaffar 94 -Esraa Saad Abbas Hamed 95 -Esraa.
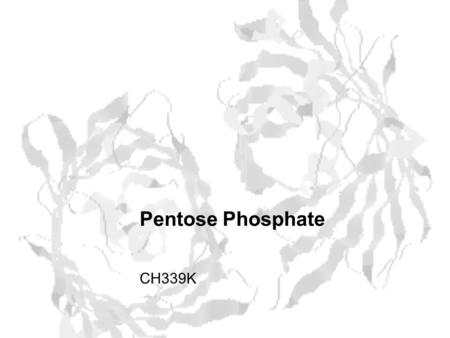
Pentose Phosphate CH339K. Pentose Phosphate An example of a pathway that can be both: Anabolic –Generates ribose-5-phosphate for nucleotide synthesis.
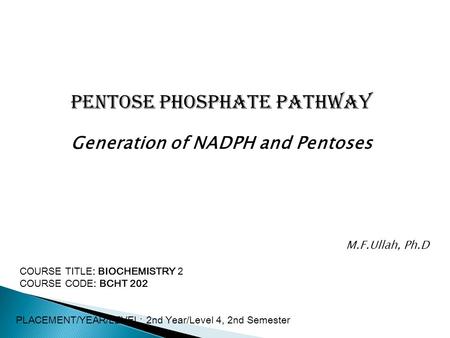
Pentose Phosphate Pathway Generation of NADPH and Pentoses COURSE TITLE: BIOCHEMISTRY 2 COURSE CODE: BCHT 202 PLACEMENT/YEAR/LEVEL: 2nd Year/Level 4, 2nd.
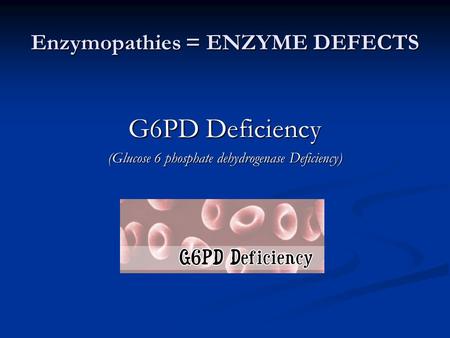
Enzymopathies = ENZYME DEFECTS
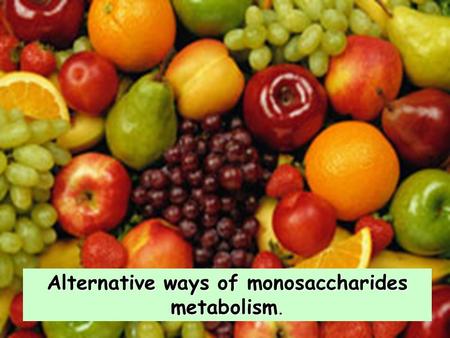
Alternative ways of monosaccharides metabolism.. Glucose The fate of glucose molecule in the cell Glucose-6- phosphate Pyruvate Glycogen Ribose, NADPH.
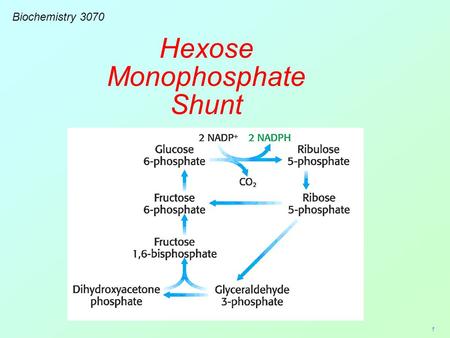
1 Biochemistry 3070 Hexose Monophosphate Shunt. 2 Biological systems utilize a variety of simple sugars which must be synthesized by the cell. These sugars.
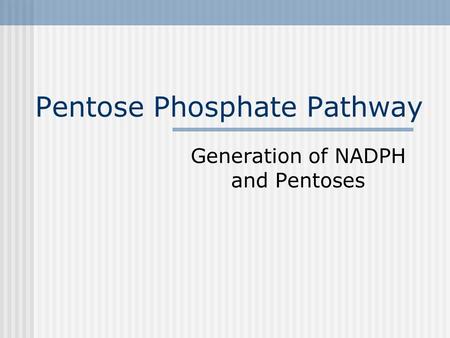
Pentose Phosphate Pathway Generation of NADPH and Pentoses.
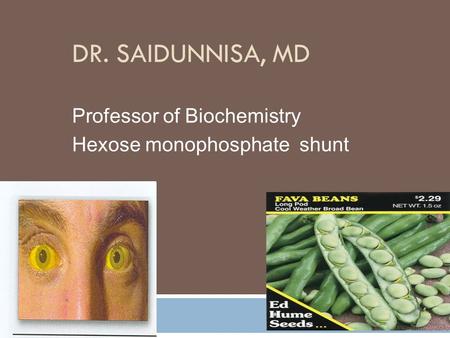
DR. SAIDUNNISA, MD Professor of Biochemistry Hexose monophosphate shunt.
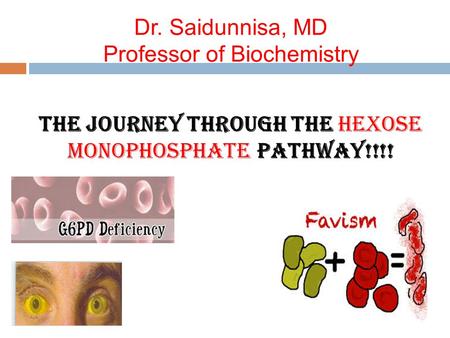
Dr. Saidunnisa, MD Professor of Biochemistry
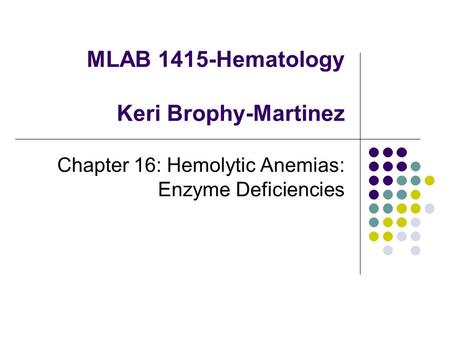
MLAB 1415-Hematology Keri Brophy-Martinez
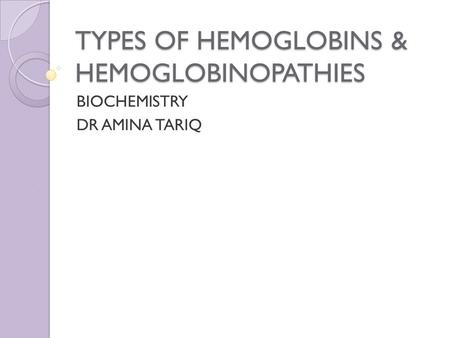
TYPES OF HEMOGLOBINS & HEMOGLOBINOPATHIES
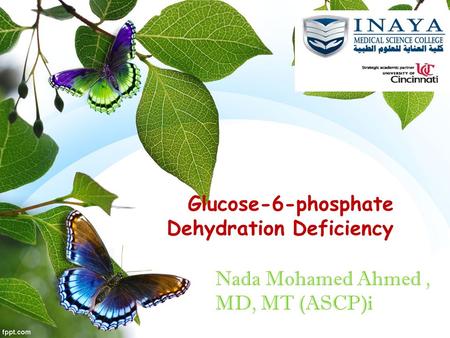
Glucose-6-phosphate Dehydration Deficiency Nada Mohamed Ahmed, MD, MT (ASCP)i.
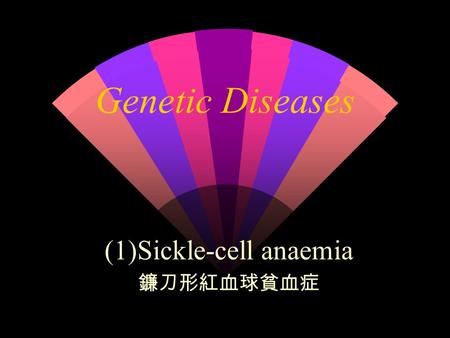
Genetic Diseases (1)Sickle-cell anaemia 鐮刀形紅血球貧血症.
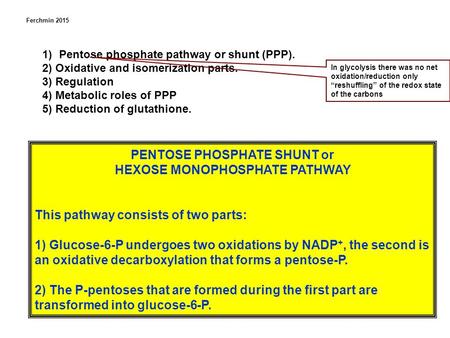
PENTOSE PHOSPHATE SHUNT or HEXOSE MONOPHOSPHATE PATHWAY This pathway consists of two parts: 1) Glucose-6-P undergoes two oxidations by NADP +, the second.
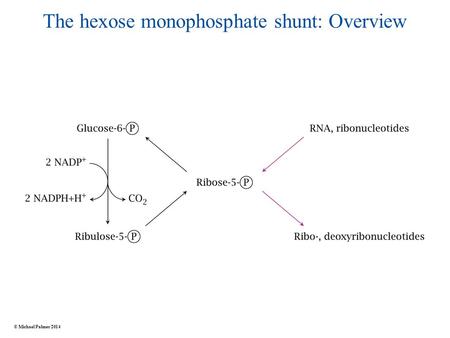
The hexose monophosphate shunt: Overview © Michael Palmer 2014.
About project
© 2024 SlidePlayer.com Inc. All rights reserved.

JENNIFER E. FRANK, MAJ, MC, USA
Am Fam Physician. 2005;72(7):1277-1282
Author disclosure: Nothing to disclose.
Glucose-6-phosphate dehydrogenase deficiency, the most common enzyme deficiency worldwide, causes a spectrum of disease including neonatal hyperbilirubinemia, acute hemolysis, and chronic hemolysis. Persons with this condition also may be asymptomatic. This X-linked inherited disorder most commonly affects persons of African, Asian, Mediterranean, or Middle-Eastern descent. Approximately 400 million people are affected worldwide. Homozygotes and heterozygotes can be symptomatic, although the disease typically is more severe in persons who are homozygous for the deficiency. The conversion of nicotinamide adenine dinucleotide phosphate to its reduced form in erythrocytes is the basis of diagnostic testing for the deficiency. This usually is done by fluorescent spot test. Different gene mutations cause different levels of enzyme deficiency, with classes assigned to various degrees of deficiency and disease manifestation. Because acute hemolysis is caused by exposure to an oxidative stressor in the form of an infection, oxidative drug, or fava beans, treatment is geared toward avoidance of these and other stressors. Acute hemolysis is self-limited, but in rare instances it can be severe enough to warrant a blood transfusion. Neonatal hyperbilirubinemia may require treatment with phototherapy or exchange transfusion to prevent kernicterus. The variant that causes chronic hemolysis is uncommon because it is related to sporadic gene mutation rather than the more common inherited gene mutation.
Glucose-6-phosphate dehydrogenase (G6PD) deficiency increases the vulnerability of erythrocytes to oxidative stress. Clinical presentations include acute hemolytic anemia, chronic hemolytic anemia, neonatal hyperbilirubinemia, and an absence of clinical symptoms. The disease is rarely fatal.
Epidemiology
G6PD deficiency occurs with increased frequency throughout Africa, Asia, the Mediterranean, and the Middle East. In the United States, black males are most commonly affected, with a prevalence of approximately 10 percent. Prevalence of the deficiency is correlated with the geographic distribution of malaria, which has led to the theory that carriers of G6PD deficiency may incur partial protection against malarial infection. 1 – 3 Cases of sporadic gene mutation occur in all populations.
Pathophysiology
G6PD catalyzes nicotinamide adenine dinucleotide phosphate (NADP) to its reduced form, NADPH, in the pentose phosphate pathway ( Figure 1 4 ). NADPH protects cells from oxidative damage. Because erythrocytes do not generate NADPH in any other way, 3 they are more susceptible than other cells to destruction from oxidative stress. The level of G6PD activity in affected erythrocytes generally is lower than in other cells. 5 Normal red blood cells that are not under oxidative stress generally exhibit G6PD activity at approximately 2 percent of total capacity. 1 Even with enzyme activity that is substantially reduced, there may be few or no clinical symptoms. A total deficiency of G6PD is incompatible with life. 6 The G6PD-deficient variants are grouped into different classes corresponding with disease severity( Table 1 1 , 7 ).
The gene mutations affecting encoding of G6PD are found on the distal long arm of the X chromosome. More than 400 mutations have been identified, most being missense mutations. 6 Most of the variants occur sporadically, although the G6PD Mediterranean and the G6PD A–variants occur with increased frequency in certain populations ( Table 2 3 , 6 , 7 ).
The diagnosis of G6PD deficiency is made by a quantitative spectrophotometric analysis or, more commonly, by a rapid fluorescent spot test detecting the generation of NADPH from NADP. 7 The test is positive if the blood spot fails to fluoresce under ultraviolet light. 8 In field research, where quick screening of a large number of patients is needed, other tests have been used; however, they require definitive testing to confirm an abnormal result. 9 , 10 Tests based on polymerase chain reaction detect specific mutations and are used for population screening, family studies, or prenatal diagnosis. 6
In patients with acute hemolysis, testing for G6PD deficiency may be falsely negative because older erythrocytes with a higher enzyme deficiency have been hemolyzed. Young erythrocytes and reticulocytes have normal or near-normal enzyme activity. Female heterozygotes may be hard to diagnose because of X-chromosome mosaicism leading to a partial deficiency that will not be detected reliably with screening tests. 7 , 11 , 12
G6PD deficiency is one of a group of congenital hemolytic anemias, and its diagnosis should be considered in children with a family history of jaundice, anemia, splenomegaly, or cholelithiasis, especially in those of Mediterranean or African ancestry. 13
Testing should be considered in children and adults (especially males of African, Mediterranean, or Asian descent) with an acute hemolytic reaction caused by infection, exposure to a known oxidative drug, or ingestion of fava beans.
Although rare, G6PD deficiency should be considered as a cause of any chronic nonspherocytic hemolytic anemia across all population groups.
Newborn screening for G6PD deficiency is not performed routinely in the United States, although it is done in countries with high disease prevalence. The World Health Organization recommends screening all newborns in populations with a prevalence of 3 to 5 percent or more in males. 3
Neonatal Hyperbilirubinemia
The prevalence of neonatal hyperbilirubinemia is twice that of the general population 14 in males who carry the defective gene and in homozygous females. It rarely occurs in heterozygous females. 15 , 16
The mechanism by which G6PD deficiency causes neonatal hyperbilirubinemia is not completely understood. Although hemolysis may be observed in neonates who have G6PD deficiency and are jaundiced, 17 other mechanisms appear to play a more important role in the development of hyperbilirubinemia. 6 , 18 , 19 Hyperbilirubinemia is likely secondary to impairment of bilirubin conjugation and clearance by the liver leading to indirect hyperbilirubinemia. 6 , 20 Infants with G6PD deficiency and a mutation of uridine diphosphoglucuronate glucuronosyltransferase-1 gene promoter (UDPGT-1) are particularly susceptible to hyperbilirubinemia secondary to decreased liver clearance of bilirubin. 21 UDPGT-1 is the enzyme affected in Gilbert disease.
G6PD deficiency should be considered in neonates who develop hyperbilirubinemia within the first 24 hours of life, a history of jaundice in a sibling, bilirubin levels greater than the 95th percentile, and in Asian males. 22 , 23
G6PD deficiency can lead to an increased risk and earlier onset of hyperbilirubinemia, 24 , 25 which may require phototherapy or exchange transfusion. 6 , 25 In certain populations, hyperbilirubinemia secondary to G6PD deficiency results in an increased rate of kernicterus and death, 26 , 27 whereas in other populations this has not been observed. 18 This may reflect genetic mutations specific to different ethnic groups. 18 , 19
Acute Hemolysis
Acute hemolysis is caused by infection, ingestion of fava beans, or exposure to an oxidative drug. 3 Medications that should be avoided in patients with G6PD deficiency are listed in Table 3 , 6 and drugs that can be used safely in these patients are listed in Table 4 . 6 Hemolysis occurs after exposure to the stressor but does not continue despite continued infection or ingestion. This is thought to be a result of older erythrocytes having the greatest enzyme deficiency and undergoing hemolysis first. Once the population of deficient erythrocytes has been hemolyzed, younger erythrocytes and reticulocytes that typically have higher levels of enzyme activity are able to sustain the oxidative damage without hemolysis. 7 Clinically, acute hemolysis can cause back or abdominal pain and jaundice secondary to a rise in unconjugated bilirubi n ( Table 5 21 ). Jaundice, in the setting of normal liver function, typically does not occur until greater than 50 percent of the erythrocytes have been hemolyzed. 21
Drugs that cause hemolysis in G6PD-deficient persons inf lict oxidative damage to erythrocytes leading to erythrocyte destruction. Hemolysis typically occurs 24 to 72 hours after ingestion, with resolution within four to seven days. 21 Oxidative drugs ingested by a woman who is breast-feeding may be transmitted in breast milk and can cause acute hemolysis in a G6PD-deficient child. 16 , 28
Although persons who experience hemolysis after the ingestion of fava beans can be presumed to have G6PD deficiency, not all of them will exhibit hemolysis. 6 , 7 Favism is most common in persons with G6PD class II variants, but rarely it can occur in patients with the G6PD A–variant. 5 Fava beans ( Table 6 ) are presumed to cause oxidative damage by an unknown component, possibly vicine, convicine, or isouramil. 6 , 7
Infection is the most common cause of acute hemolysis in G6PD-deficient persons, 6 although the exact mechanism by which this occurs is unknown. Leukocytes may release oxidants during phagocytosis that cause oxidative stress to the erythrocytes; however, this explanation alone would not account for the variety of infections associated with hemolysis in G6PD-deficient persons. The most common infectious agents causing hemolysis include Salmonella, Escherichia coli, beta-hemolytic streptococci, rickettsial infections, viral hepatitis, and influenza A.
A peripheral smear taken during an acute hemolytic reaction in a G6PD-deficient person may demonstrate Heinz bodies, although this rarely is seen in clinical practice. 7
Chronic Hemolysis
In chronic nonspherocytic hemolytic anemia, which usually is caused by a sporadic gene mutation, hemolysis occurs during normal erythrocyte metabolism. 5 , 6 The severity of the hemolysis varies, causing mild hemolysis to transfusion-dependent anemia. Exposure to oxidative stress can cause acute hemolysis in these persons.
Other Clinical Considerations
G6PD-deficient persons are predisposed to the development of sepsis and complications related to sepsis after a severe injury. 29 Although research has failed to consistently show a clinically significant risk to patients receiving G6PD-deficient donor blood, blood banks generally do not accept G6PD-deficient blood donors. 30
The main treatment for G6PD deficiency is avoidance of oxidative stressors. Rarely, anemia may be severe enough to warrant a blood transfusion. Splenectomy generally is not recommended. Folic acid and iron potentially are useful in hemolysis, although G6PD deficiency usually is asymptomatic and the associated hemolysis usually is short-lived. Antioxidants such as vitamin E and selenium have no proven benefit for the treatment of G6PD deficiency. 6 , 31 Research is being done to identify medications that may inhibit oxidative-induced hemolysis of G6PD-deficient red blood cells. 32
Ruwende C, Hill A. Glucose-6-phosphate dehydrogenase deficiency and malaria. J Mol Med. 1998;76:581-8.
Mockenhaupt FP, Mandelkow J, Till H, Ehrhardt S, Eggelte TA, Bienzle U. Reduced prevalence of Plasmodium falciparum infection and of concomitant anaemia in pregnant women with heterozygous G6PD deficiency. Trop Med Int Health. 2003;8:118-24.
WHO Working Group. Glucose-6-phosphate dehydrogenase deficiency. Bull World Health Organ. 1989;67:601-11.
Glucose 6 phosphate dehydrogenase deficiency. Accessed July 20, 2005, at:http://www.malariasite.com/malaria/g6pd.htm.
Mason PJ. New insights into G6PD deficiency. Br J Haematol. 1996;94:585-91.
Beutler E. G6PD deficiency. Blood. 1994;84:3613-36.
Gregg XT, Prchal JT. Red cell enzymopathies. In: Hoffman R, ed. Hematology: basic principles and practice. 4th ed. Philadelphia: Churchill Livingstone, 2000:657–60.
Glucose-6-phosphate dehyrdogenase deficiency. Accessed online July 20, 2005, at: http://www.answers.com/glucose-6-phosphate-dehydrogenase-deficiency .
Jalloh A, Tantular IS, Pusarawati S, Kawilarang AP, Kerong H, Lin K, et al. Rapid epidemiologic assessment of glucose-6-phosphate dehydrogenase deficiency in malaria-endemic areas in Southeast Asia using a novel diagnostic kit. Trop Med Int Health. 2004;9:615-23.
Iwai K, Matsuoka H, Kawamoto F, Arai M, Yoshida S, Hirai M, et al. A rapid single-step screening method for glucose-6-phosphate dehydrogenase deficiency in field applications. Japanese Journal of Tropical Medicine and Hygiene. 2003;31:93-7.
Ainoon O, Alawiyah A, Yu YH, Cheong SK, Hamidah NH, Boo NY, et al. Semiquantitative screening test for G6PD deficiency detects severe deficiency but misses a substantial proportion of partially-deficient females. Southeast Asian J Trop Med Public Health. 2003;34:405-14.
Reclos GJ, Hatzidakis CJ, Schulpis KH. Glucose-6-phosphate dehydrogenase deficiency neonatal screening: preliminary evidence that a high percentage of partially deficient female neonates are missed during routine screening. J Med Screen. 2000;7:46-51.
Hermiston ML, Mentzer WC. A practical approach to the evaluation of the anemic child. Pediatr Clin North Am. 2002;49:877-91.
Glucose-6-phosphate dehydrogenase; G6PD. Accessed online July 20, 2005, at: http://www3.ncbi.nlm.nih.gov/entrez/dispomim.cgi?id=305900 .
Kaplan M, Hammerman C, Vreman HJ, Stevenson DK, Beutler E. Acute hemolysis and severe neonatal hyper-bilirubinemia in glucose-6-phosphate dehydrogenase-deficient heterozygotes. J Pediatr. 2001;139:137-40.
Corchia C, Balata A, Meloni GF, Meloni T. Favism in a female newborn infant whose mother ingested fava beans before delivery. J Pediatr. 1995;127:807-8.
Bizzarro MJ, Colson E, Ehrenkranz RA. Differential diagnosis and management of anemia in the newborn. Pediatr Clin North Am. 2004;51:1087-107.
Kaplan M, Vreman HJ, Hammerman C, Leiter C, Abramov A, Stevenson DK. Contribution of haemolysis to jaundice in Sephardic Jewish glucose-6-phosphate dehydrogenase deficient neonates. Br J Haematol. 1996;93:822-7.
Seidman DS, Shiloh M, Stevenson DK, Vreman HJ, Gale R. Role of hemolysis in neonatal jaundice associated with glucose-6-phosphate dehydrogenase deficiency. J Pediatr. 1995;127:804-6.
Kaplan M, Rubaltelli FF, Hammerman C, Vilei MT, Leiter C, Abramov A, et al. Conjugated bilirubin in neonates with glucose-6-phospate dehydrogenase deficiency. J Pediatr. 1996;128(5 pt 1):695-7.
Edwards CQ. Anemia and the liver. Hepatobiliary manifestations of anemia. Clin Liver Dis. 2002;6:891-907.
Bhutani VK, Johnson LH, Keren R. Diagnosis and management of hyperbilirubinemia in the term neonate: for a safer first week. Pediatr Clin North Am. 2004;51:843-61.
American Academy of Pediatrics Subcommittee on Hyperbilirubinemia. Management of hyperbilirubinemia in the newborn infant 35 or more weeks of gestation [published correction appears in Pediatrics 2004;114:1138]. Pediatrics. 2004;114:297-316.
Valaes T. Fractionation of serum bilirubin conjugates in the exploration of the pathogenesis of significant neonatal bilirubinemia associated with glucose-6-phosphate dehydrogenase deficiency. J Pediatr. 1997;130:678-9.
Kaplan M, Abramov A. Neonatal hyperbilirubinemia associated with glucose-6-phosphate dehydrogenase deficiency in Sephardic-Jewish neonates: incidence, severity, and the effect of phototherapy. Pediatrics. 1992;90:401-5.
Slusher TM, Vreman HJ, McLaren DW, Lewison LJ, Brown AK, Stevenson DK. Glucose-6-phosphate dehydrogenase deficiency and carboxyhemoglobin concentrations associated with bilirubin-related morbidity and death in Nigerian infants. J Pediatr. 1995;126:102-8.
Nair PA, Al Khusaiby SM. Kernicterus and G6PD deficiency—a case series from Oman. J Trop Pediatr. 2003;49:74-7.
American Academy of Pediatrics Committee on Drugs. Transfer of drugs and chemicals into human milk. Pediatrics. 2001;108:776-89.
Spolarics Z, Siddiqi M, Siegel JH, Garcia ZC, Stein DS, Ong H, et al. Increased incidence of sepsis and altered monocyte functions in severely injured type A- glucose-6-phosphate dehydrogenase-deficient African American trauma patients. Crit Care Med. 2001;29:728-36.
CBBS e-Network Forums. Eligibility of prospective blood donors known to have G6PD deficiency. Accessed online July 20, 2005, at:http://www.cbbsweb.org/enf/donor_g6pd.html.
Glucose-6-phosphate dehydrogenase deficiency. Accessed online July 20, 2005, at:http://www.thedoctorslounge.net/clinlounge/diseases/hematology/g6pd.htm.
Sharma SC, Sharma S, Gulati OP. Pycnogenol prevents haemolytic injury in G6PD deficient human erythrocytes. Phytother Res. 2003;17:671-4.
Continue Reading
More in afp, more in pubmed.
Copyright © 2005 by the American Academy of Family Physicians.
This content is owned by the AAFP. A person viewing it online may make one printout of the material and may use that printout only for his or her personal, non-commercial reference. This material may not otherwise be downloaded, copied, printed, stored, transmitted or reproduced in any medium, whether now known or later invented, except as authorized in writing by the AAFP. See permissions for copyright questions and/or permission requests.
Copyright © 2024 American Academy of Family Physicians. All Rights Reserved.
Learn how UpToDate can help you.
Select the option that best describes you
- Medical Professional
- Resident, Fellow, or Student
- Hospital or Institution
- Group Practice
- Patient or Caregiver
- Find in topic
RELATED TOPICS
INTRODUCTION
The severity of hemolytic anemia varies among individuals with G6PD deficiency, making diagnosis more challenging in some cases. Identification of G6PD deficiency and patient education regarding safe and unsafe medications and foods is critical to preventing future episodes of hemolysis.
This topic review discusses the clinical manifestations, diagnosis, and management of G6PD deficiency. Separate topic reviews discuss the genetic testing and pathogenesis of G6PD deficiency and an overall approach to the patient with unexplained hemolytic anemia.
● G6PD deficiency
• Genetic testing – (See "Gene test interpretation: G6PD " .)
Select a Community
- MB 1 Preclinical Medical Students
- MB 2/3 Clinical Medical Students
- ORTHO Orthopaedic Surgery
Are you sure you want to trigger topic in your Anconeus AI algorithm?
You are done for today with this topic.
Would you like to start learning session with this topic items scheduled for future?
G6PD Deficiency
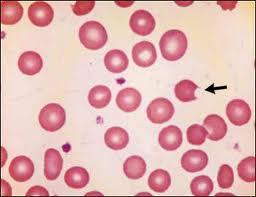
- glucose-6-phosphate dehydrogenase (G6PD) deficiency is an X-linked genetic disorder that causes an intrinsic hemolytic anemia
- 7.1% worldwide
- most common enzyme disorder of erythrocytes
- more severe in males than females
- sub-Saharan Africa
- Middle East
- southeast Asia
- Mediterranean regions
- Pacific islands
- this pathway generates nicotinamide dinucleotide phosphate (NADPH), which protects red blood cells against oxidative stress
- in red blood cells (without mitochondria), this pathway is the only source of NADPH
- sulfa drugs
- fava bean ingestion
- precipitation of hemoglobin (manifested as Heinz bodies)
- erythrocyte membrane damage, both extravascular and intravascular hemolysis
- G6PD deficiency is thought to decrease risk of severe malaria
- G6PD gene encoding the G6PD enzyme
- typically asymptomatic until exposed to oxidative stressors
- neonatal hyperbilirubinemia on day 2-4
- complete blood count and reticulocyte count
- Heinz bodies
- ↑ indirect bilirubin
- ↓ haptoglobin
- ↑ lactate dehydrogenase
- hemoglobinuria
- most sensitive
- methemoglobin reduction test
- a normal G6PD level immediately after hemolysis does not rule out G6PD deficiency
- spectrophotometry analysis
- jaundice at birth (rather than delayed onset of jaundice)
- normal G6PD enzyme activity
- spherocytosis seen on peripheral blood smear
- avoid oxidative stressors
- Recurrence of acute hemolysis
- - G6PD Deficiency
Please Login to add comment

Enter search terms to find related medical topics, multimedia and more.
Advanced Search:
- Use “ “ for exact phrases.
- For example: “pediatric abdominal pain”
- Use – to remove results with certain keywords.
- For example: abdominal pain -pediatric
- Use OR to account for alternate keywords.
- For example: teenager OR adolescent
Glucose-6-Phosphate Dehydrogenase (G6PD) Deficiency
, MD, PhD, Johns Hopkins University School of Medicine
- Pathophysiology
- Symptoms and Signs
- 3D Models (0)
- Calculators (0)

Glucose-6-phosphate dehydrogenase (G6PD) deficiency is an X-linked enzymatic defect common in people with African ancestry that can result in hemolysis after acute illnesses or intake of oxidant drugs (including salicylates and sulfonamides). Diagnosis is based on assay for G6PD, although test results are often falsely negative during acute hemolysis due to the presence of reticulocytes, which are richer in G6PD than older cells. Treatment is supportive.

G6PD deficiency, a defect in the hexose monophosphate shunt pathway, is the most common disorder of red blood cell (RBC) metabolism. The G6PD gene is located on the X chromosome and exhibits a high amount of variation (polymorphism), resulting in a range of G6PD activity from normal to severely deficient. Variants are classified I through V by the amount of activity of the G6PD enzyme. Because the gene is X-linked, males are more likely to present with clinically significant hemolysis. Females who are homozygous, or who are heterozygous with skewed X inactivation that results in a high proportion of affected X chromosomes may also be affected.
In the US, this defect is most common in people with African ancestry, occurring in about 10% of male African Americans and in 10% of female African Americans. It occurs in lower frequencies among people from the Mediterranean basin (eg, Italian, Greek, Arab, or Sephardic Jewish ancestry) and people with Asian ancestry.
Pathophysiology of G6PD Deficiency
G6PD deficiency renders RBCs susceptible to oxidative stress, which shortens RBC survival. Hemolysis occurs following an oxidative challenge, commonly after fever, acute viral or bacterial infections, and diabetic ketoacidosis Diabetic Ketoacidosis (DKA) Diabetic ketoacidosis (DKA) is an acute metabolic complication of diabetes characterized by hyperglycemia, hyperketonemia, and metabolic acidosis. Hyperglycemia causes an osmotic diuresis with... read more . Hemolysis is episodic and self-limited, although rare patients have chronic, ongoing hemolysis in the absence of oxidative challenge.
Less commonly, hemolysis occurs after exposure to drugs or to other substances that produce peroxide and cause oxidation of hemoglobin and RBC membranes. These drugs and substances include primaquine , salicylates, sulfonamides, nitrofurans, phenacetin, naphthalene, some vitamin K derivatives, dapsone , phenazopyridine , nalidixic acid, methylene blue , and, in some cases, fava beans. The amount of hemolysis depends on the degree of G6PD deficiency and the oxidant potential of the drug.
Symptoms and Signs of G6PD Deficiency

Diagnosis of G6PD Deficiency
Peripheral smear

The peripheral smear may reveal RBCs that appear to have a blister (blister cells) or have one or more "bites" (1-micron wide) taken from the cell periphery (bite cells) and RBCs with inclusions termed Heinz bodies, which are particles of denatured hemoglobin, which can be recognized only by special stains. These cells may be visible early during the hemolytic episode but do not persist in patients with an intact spleen, which removes them.
Testing for G6PD activity is available. However, during and immediately after a hemolytic episode, tests may yield false-negative results because of destruction of the older, more deficient RBCs and the production of reticulocytes, which are rich in G6PD. Thus, testing may need to be repeated several weeks after the acute event. Several screening tests are available, including point-of-care tests; positive results should be confirmed with a quantitative test.
Treatment of G6PD Deficiency
Avoidance of triggers, removal of offending drug or agent, and supportive care
During acute hemolysis, treatment is supportive; transfusions are rarely needed. Patients are advised to avoid drugs or substances that initiate hemolysis.
Glucose-6-phosphate dehydrogenase (G6PD) deficiency is the most common inherited disorder of red blood cell metabolism and can cause hemolysis in the presence of triggers.
Incidence is higher in certain ethnic groups (eg, people with African, Mediterranean, or Asian ancestry).
Triggers include acute illnesses (eg, infections), drugs (eg, salicylates) and other substances (eg, fava beans) that cause oxidative stress.
Diagnose using peripheral smear and G6PD assay; false negative G6PD assays are possible during acute hemolysis so repeat testing after several weeks if initial G6PD assay is negative.
Avoid triggers to limit hemolytic episodes.

Was This Page Helpful?

Test your knowledge
Brought to you by Merck & Co, Inc., Rahway, NJ, USA (known as MSD outside the US and Canada) — dedicated to using leading-edge science to save and improve lives around the world. Learn more about the MSD Manuals and our commitment to Global Medical Knowledge.
- Permissions
- Cookie Settings
- Terms of use
- Veterinary Manual

- IN THIS TOPIC
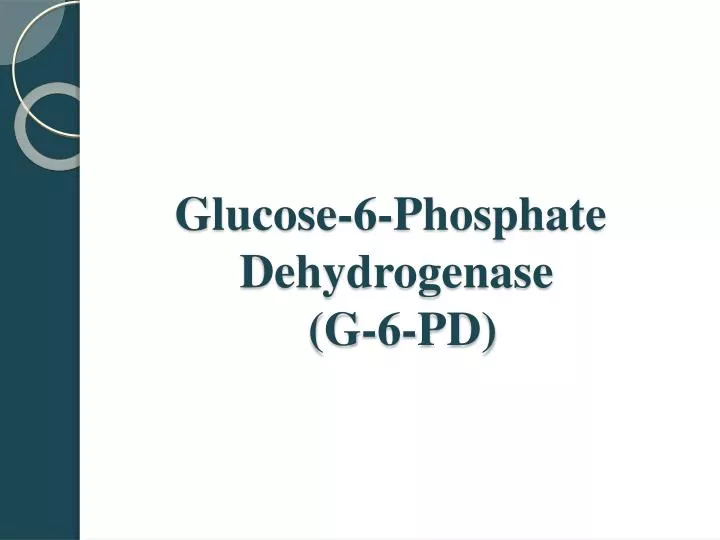
Glucose-6-Phosphate Dehydrogenase (G-6-PD)
Aug 10, 2014
390 likes | 1.46k Views
Glucose-6-Phosphate Dehydrogenase (G-6-PD). Introduction. G6PD deficiency is an allelic abnormality which is inherited in an X-linked recessive fashion. G6PD deficiency is also known as "favism" since G6PD deficient individuals are also sometimes allergic to fava beans .
Share Presentation
- constant temperature cuvete compartment
- red cell count
- dichlorophenol indophenols
- whole blood
- g-6-pdh substrate
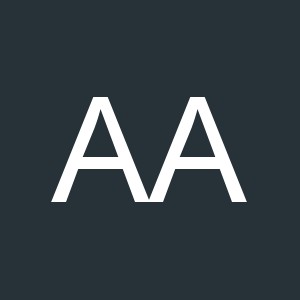
Presentation Transcript
Glucose-6-Phosphate Dehydrogenase(G-6-PD)
Introduction • G6PD deficiency is an allelic abnormality which is inherited in an X-linked recessive fashion. • G6PD deficiency is also known as "favism" since G6PD deficient individuals are also sometimes allergic to fava beans. • Glucose-6-Phosphate Dehydrogenase (G6PD) deficiency is the most common human enzyme deficiency in the world; it affects an estimated 400 million people.
When someone has G6PD deficiency, complications can arise; hemolytic anemia • In addition to being susceptible to hemolytic anemia, G6PD deficient individuals are also predisposed to prolonged neonatal jaundice, this can be a potentially serious problem as it can cause severe neurological complications and even death.. • Both of these conditions are directly related to the inability of specific cell types to regenerate reduced nicotinamide adenine dinucleotide phosphate (NADPH); this reaction is normally catalyzed by the G6PD enzyme.
In G6PD deficient individuals, anemia is usually caused by certain oxidative drugs, infections, or fava beans. • When any one of these agents, or their metabolites, enters a G6PD deficient red blood cell, hemoglobin becomes denatured, thus destroying its function as the principal oxygen carrying molecule.
Principle: • Glucose-6-phosphate dehydrogenase (G6PDH, D-glucose-6-phosphate) catalyzes the first step in the pentose phosphate shunt, oxidizing glucose-6-phosphate (G-6-P)to 6-phosphogluconate(6-PG) and reducing NADP to NADPH, which illustrated by the following equation: G-6-P + NADP+ G-6PDH 6-PG + NADPH +H+ • NADP is reduced by G-6-PDH in the presence of G-6-P. The rate of formation of NADPH is directly proportional to the G-6-PDH activity and is measured spectrophotometrically as an increased in absorbance at 340nm.
Principle Cont. • Production of a second molar equivalent of NADPH by erythrocyte 6-phosphogluconate dehydrogenase (6-PGDH) according to the reaction : 6-PG + NADP+G-6PDH Ribulose-5- phosphate + NADPH + H+ + CO2 SpecimenWhole blood collected with EDTA, or acid citrate dextrose .
Stability and Storage: • Red cell G-6-PDH is stable in whole blood for one week refrigerated (2-8ºc), but is unstable in red cell hemolysates. • Since activity is reported in term of number of red blood cell or gram hemoglobin, the red cell count or hemoglobin concentration should be determined prior to performing the G6PDH assay.
Procedure • The temperature of the reaction mixture should be maintained at 30ºc or some other constant temperature. • prepare reaction mixture: • Add 0.01ml blood directly to vial containing G-6-PDH assay solution and mix thoroughly to completely suspend erythrocytes, let stand at room temperature(18-25ºc) for 5-10min. • Add 2.0ml G-6-PDH substrate solution directly to vial and mix gently by inverting several times. • Transfer contents of vial to cuvete.
Procedure Cont. • Place cuvete in constant temperature cuvete compartment or water bath and incubate for approximately 5min to attain thermal equilibrium. • Read and record absorbance (A1) of test at 340nm against water or potassium dichromate solution. This is initial A (if using a water bath or incubator , return cuvete to it). • Exactly 5min later, again read and record (A2), this is final A.
Calculation: To determine G-6-PDH activity, do the following calculations: • ΔA per min =( A2 - A1 )/5 • G-6-PDH activity is expressed as U/1012 erythrocyte (RBC)or as U/g hemoglobin (Hb). • G-6-PDH (U/1012 RBC) = ( ΔA per min X 3.01 X 1012 X TCF) / 0.01 X 6.22 X (N X 10*6) X 1000
Where: • 3.01 = total reaction volume(ml). • 1012= factor for expressing activity in1012 cells. • 0.01 = sample volume (ml) • 6.22 = millimolar absorptive of NADPH at 340 nm. • N X 106 = red cell count (red cells/mm³ determined for each specimen. • 1000 = conversion of red cell count from mm³ to ml. • TCF = temperature correction factor (1at 30ºc).
This equation reduced to: G-6-PDH ( U/1012 RBC)= ΔA/min X (48,390/N) X TCF Where: • N = red cell count divided by 106 • TCF = temperature correction factor (1at 30ºc) • G-6-PDH(U/g Hb) = ΔA per min X 100 X 3.01 / ((0.01 X6.22 X Hb (g/dl)) X TCF = ΔA per min X 4839 / Hb (g/dl) X TCF
Where: • 100 = factor to convert activity to 100ml • 3.01 = total reaction volume (ml) • 0.01 = sample volume (ml) • 6.22 = mill molar absorptive of NADPH at 340 nm • Hb (g/dl) = hemoglobin concentration determined for each specimen • TCF = temperature correction factor (1 at 30ºc)
Note: • If anemia and/or leukocytosis is present: Use Buffy coat free blood sample for assay (platelets and WBCs marked activity in this enzyme) • Normal range: G-6-PDH (U/1012 RBC): (146-376) G-6-PDH (U/g Hb): (4.6-13.5)
Qualitative Method in G-6-DP Determination
Principle: Glucose -6-phosphate dehydrogenase present in the red blood cell hemolysate, act on glucose -6-phosphate and reduces NADP to NADPH which, with the help of PMS, reduces blue colored 2,6 Dichlorophenol Indophenols into a colorless form. The reaction can be represented as: G-6-phosphate + NADP 6-phosphogluconic acid + NADPH NADPH + 2,6 Dichlorophenol indophenols (DCPIP)(Blue color) NADP + Reduced DCPIP (colorless) • Rate of declorization is directly proportional to the activity of G-6-PD.
Note: • Fresh blood sample should be use since refrigeration reduces the enzyme activity. • Heparin sample should not be use as interfere with enzyme reaction. • Avoid exposure of substrate vial to the light (it is photosensitive).
Procedure: Step1: Preparation of red cell hemolysate: • Purified water : 2.5ml • Fresh blood : 0.05ml • Mix well and allow standing for 5min at R.T. Step2: Assay of the enzyme: • Add 1mi of the hemolysate (step 1) to the vial of solution 1 and mix gently. • Add immediately about 1ml of reagent 3. • Seal the vial with aluminum foil and incubate in water bath at 37ºc.
Procedure Cont. Observe: • the time taken for the color change from initial deep blue to reddish purple. Follow up to a maximum of 6 hours with 30 min intervals. Results: • Normal : 30-60 min. • G-6-PD deficient (heterozygous males, homozygous female): 140min-24hr • G-6-Pdcarriers (heterozygous females): 90min-several hours.
- More by User
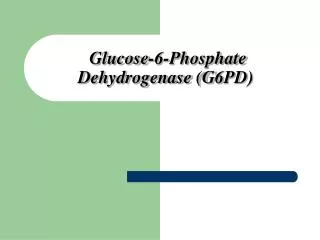
Glucose-6-Phosphate Dehydrogenase (G6PD)
Glucose-6-Phosphate Dehydrogenase (G6PD). Glucose-6-Phosphate Dehydrogenase (G6PD) deficiency.
1.98k views • 13 slides
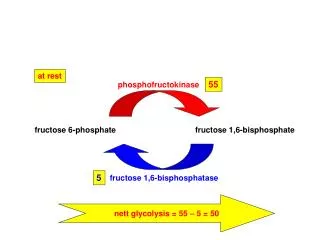
fructose 6-phosphate
at rest. 55. 5. phosphofructokinase. fructose 1,6-bisphosphate. fructose 6-phosphate. fructose 1,6-bisphosphatase. nett glycolysis = 55 – 5 = 50. anticipation. 40 x 55 = 2200. 430 x 5 = 2150. phosphofructokinase. fructose 1,6-bisphosphate. fructose 6-phosphate.
208 views • 3 slides
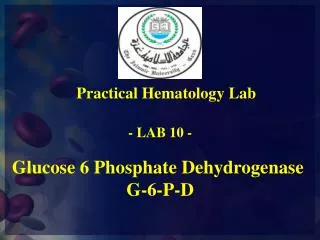
Glucose 6 Phosphate Dehydrogenase G-6-P-D
Practical Hematology Lab. - LAB 10 -. Glucose 6 Phosphate Dehydrogenase G-6-P-D. Introduction. G6PD deficiency is an allelic abnormality which is inherited in an X-linked recessive fashion.
504 views • 20 slides
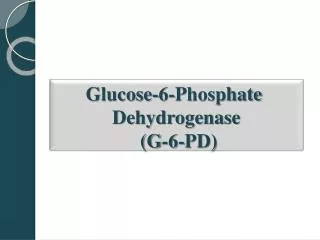
1.11k views • 19 slides
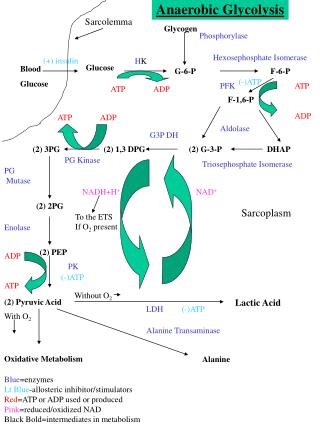
Blood Glucose
Anaerobic Glycolysis. Sarcolemma. Glycogen. Phosphorylase. Hexosephosphate Isomerase. (+) insulin. H K. Glucose. Blood Glucose. G-6-P. F-6-P. (-)ATP. PFK. ATP ADP. ATP ADP. F-1,6-P. ATP ADP. Aldolase. G3P DH. (2) 3PG. (2) 1,3 DPG. (2) G-3-P. DHAP. PG Kinase.
263 views • 4 slides
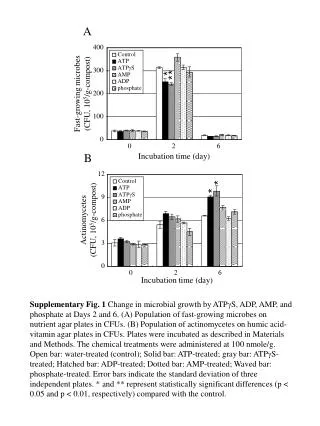
Control ATP ATP g S AMP ADP phosphate
A. 400 300 200 100 0. Control ATP ATP g S AMP ADP phosphate. *. **. Fast-growing microbes (CFU, 10 5 /g-compost). 0. 2. 6. B. Incubation time (day). 12 9 6 3 0. *. Control ATP ATP g S AMP ADP phosphate. *. Actinomycetes (CFU, 10 5 /g-compost). 0. 2. 6.
134 views • 1 slides
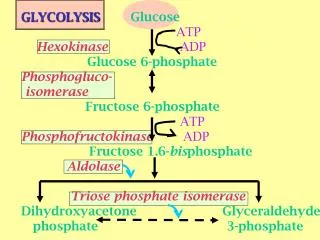
GLYCOLYSIS Glucose ATP Hexokinase ADP Glucose 6-phosphate P hosphogluco- i somerase
GLYCOLYSIS Glucose ATP Hexokinase ADP Glucose 6-phosphate P hosphogluco- i somerase Fructose 6-phosphate ATP Phosphofructokinase ADP Fructose 1.6- bis phosphate Aldolase Triose phosphate isomerase Dihydroxyacetone Glyceraldehyde phosphate 3-phosphate.
565 views • 21 slides
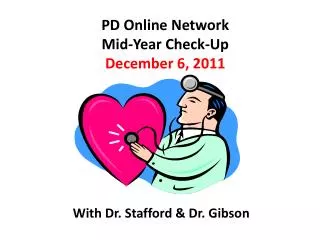
PD Online Network Mid-Year Check-Up December 6, 2011
PD Online Network Mid-Year Check-Up December 6, 2011. With Dr. Stafford & Dr. Gibson. Doctors’ Checklist. Check components are open Check implementation progress Check evaluation phase progress Marzano PD Resources 6 Topics for District PD Training Overall PD Health of Your School.
272 views • 15 slides
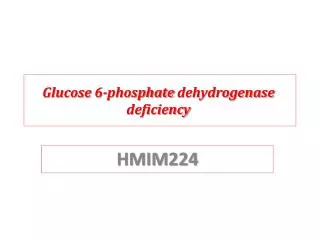
Glucose 6-phosphate dehydrogenase deficiency
Glucose 6-phosphate dehydrogenase deficiency. HMIM224. Objectives of the Lecture. 1- Understanding the main functional concepts for pentose phosphate pathway (also called hexose monophosphate pathway). 2- Identify the main general uses of NADPH for normal cellular
1.15k views • 25 slides
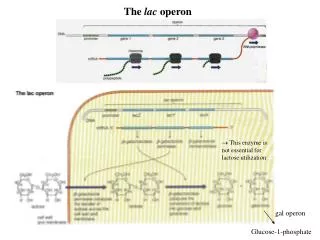
Glucose-1-phosphate
The lac operon. This enzyme is not essential for lactose utilization. gal operon. Glucose-1-phosphate. La presenza di lattosio e/o glucosio controlla l’espressione dei geni lac. lac operon is induced only when lactose is present. - lacI gene is located outside the operon
318 views • 8 slides
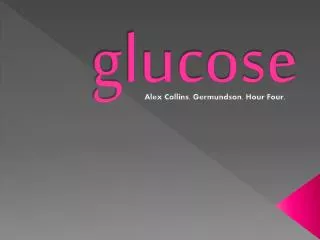
glucose. Alex Collins. Germundson. Hour Four. Chemical Name- Glucose Chemical Formula- GlC Common Name- Dextrose Synonyms- Cellulose, Maltose, Lactose, Sucrose Chemical Formula- C6H12O6 Formula Weight- 180g/ mol. (6 carbons at 12 g/ mol =72g) (12 H at 1 g/ mol =12g)
252 views • 6 slides
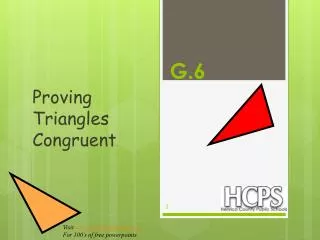
Proving Triangles Congruent. G.6. Visit www.worldofteaching.com For 100’s of free powerpoints. F. B. A. C. E. D. The Idea of Congruence. Two geometric figures with exactly the same size and shape. How much do you need to know. . . . . . about two triangles
574 views • 47 slides
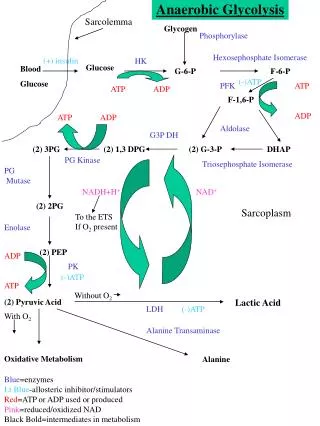
Anaerobic Glycolysis. Sarcolemma. Glycogen. Phosphorylase. Hexosephosphate Isomerase. (+) insulin. HK. Glucose. Blood Glucose. G-6-P. F-6-P. (-)ATP. PFK. ATP ADP. ATP ADP. F-1,6-P. ATP ADP. Aldolase. G3P DH. (2) 3PG. (2) 1,3 DPG. (2) G-3-P. DHAP. PG Kinase.
276 views • 4 slides
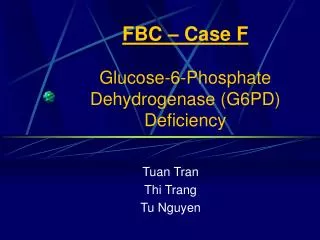
FBC – Case F Glucose-6-Phosphate Dehydrogenase (G6PD) Deficiency
FBC – Case F Glucose-6-Phosphate Dehydrogenase (G6PD) Deficiency. Tuan Tran Thi Trang Tu Nguyen. Patient History. Discussion Issues. Variations of G6PD Clinical features of G6PD Management of G6PD Potential adverse effects of patients medication (Bactrim and Aspirin) on his FMC
1.24k views • 29 slides
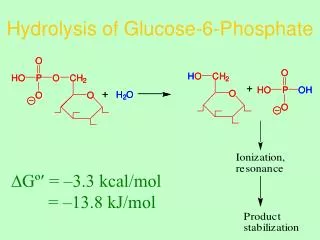
Hydrolysis of Glucose-6-Phosphate
Hydrolysis of Glucose-6-Phosphate. ∆Gº = –3.3 kcal/mol. = –13.8 kJ/mol. High ∆Gº Hydrolysis Compounds. ∆Gº = –14.8 kcal/mol = –61.9 kJ/mol. High ∆Gº Hydrolysis Compounds. ∆Gº = –11.8 kcal/mol = –49.3 kJ/mol. High ∆Gº Hydrolysis Compounds. ∆Gº = –10.3 kcal/mol = –43 kJ/mol.
629 views • 30 slides
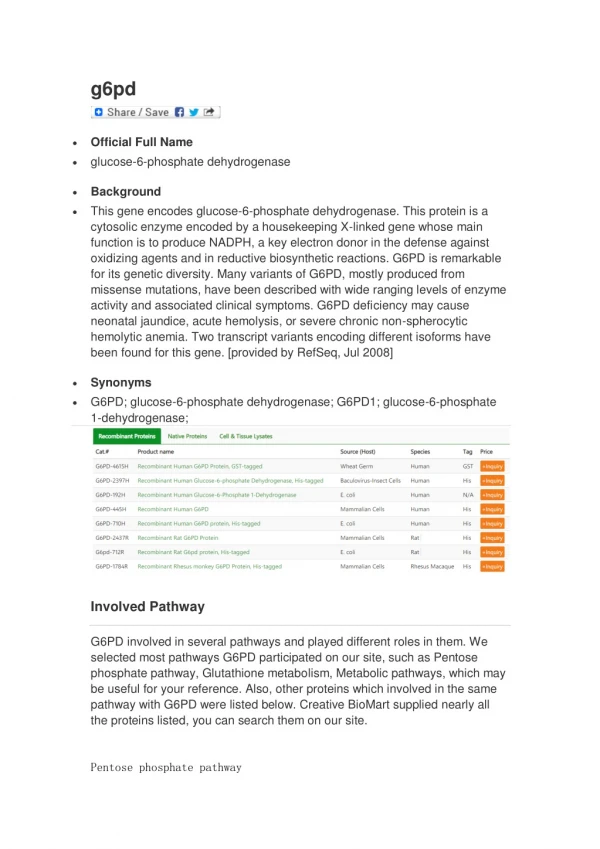
Official Full Name glucose-6-phosphate dehydrogenase
37 views • 3 slides
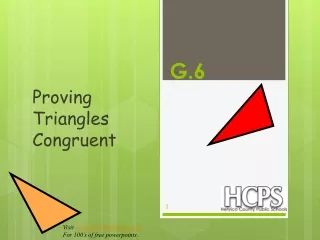
481 views • 47 slides
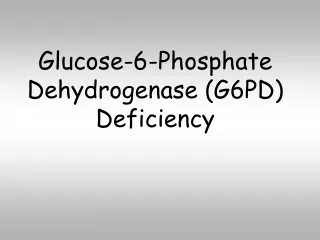
Glucose-6-Phosphate Dehydrogenase (G6PD) Deficiency
Glucose-6-Phosphate Dehydrogenase (G6PD) Deficiency. Introduction. GENETICS. INHERITANCE. RACE. RACE. INHERITANCE. G6PD dehydrogenase gene is carried on the X chromosome Females produce the same amount of G6PD enzyme as males There are variant alleles of the G6PD gene.
342 views • 20 slides

An official website of the United States government
The .gov means it’s official. Federal government websites often end in .gov or .mil. Before sharing sensitive information, make sure you’re on a federal government site.
The site is secure. The https:// ensures that you are connecting to the official website and that any information you provide is encrypted and transmitted securely.
- Publications
- Account settings
Preview improvements coming to the PMC website in October 2024. Learn More or Try it out now .
- Advanced Search
- Journal List
- Asian J Transfus Sci
- v.7(2); Jul-Dec 2013
Interesting case of G6PD deficiency anemia with severe hemolysis
Anupam chhabra.
Department of Transfusion Medicine, Pushpanjali Crosslay Hospital, Ghaziabad, India
Pankaj N. Choudhary
1 Department of Internal Medicine, Pushpanjali Crosslay Hospital, Ghaziabad, India
Ashok Grover
Severe hemolysis was observed in a critically ill patient with G6Pd deficiency where the causative trigger could not be identified. We describe one young patient with severe hemolysis treated with two cycles of plasmapheresis which proved to be an effective tool in the treatment. The patient presented with diffuse pain abdomen, vomiting, yellowish discoloration of sclera and skin and acute breathlessness. Hemoglobin 5.4 mg/dl and total (T) serum bilirubin 17.08 mg/dl: Direct (D) 4.10 mg/dl and Indirect (I) 12.98 mg/dl. Subsequently patient started passing black color urine. As the patient developed severe hemolysis and the trigger agent of hemolysis was unknown, two cycles of plasmapheresis were performed with the aim to remove unknown causative agent. Consequently no trace of hemolysis was found and patient stabilized. Plasmapheresis can be used to treat G6PD deficient patients with severe hemolysis due to unidentified trigger agent.
Introduction
Glucose-6-phosphate dehydrogenase (G6PD) is a house keeping enzyme critical in the redox metabolism of all aerobic cells. G6PD deficiency is the most common human enzyme defect. Though majority remains clinically asymptomatic the risk of developing AHA still remains. The three known trigger factors being (i) favism beans (ii) infections (iii) drugs like antimalarial, sulphonamides/sulphones, antibacterial/ antibiotics/antipyretic/analgesics etc.[ 1 ] We describe the case of young male patient with G6PD deficiency, critically ill with severe hemolysis treated with plasmapheresis, demonstrated sufficient recovery.

Case Report
A 23 year old male patient presented with diffused pain abdomen and vomiting for two days, yellowish discoloration of urine, sclera and skin for one day, acute breathlessness for four hours, fever (100.4°F), pallor, moderate icterus, high pulse rate(124/minute), respiratory rate of 28/minute, cyanosis and reduced oxygen saturation, Hemoglobin 9.6 gm/dl, TLC 38,840/μl with neutrophilia, reticulocyte count 5%, serum bilirubin (T) 24.95 mg/dl, serum bilirubin (D) 3.43 mg/dl, serum bilirubin (I) 21.52 mg/dl , SGPT 61.39 IU/L, SGOT122.48 IU/L,GGT 39.37 IU/L, blood urea 68.62 mg/dl, S.Creatinine 0.62 mg/dl, G6PD (Qualitative)-discoloration in more than 60 minutes, urine – red/brown, negative screening for malarial parasite and dengue.
In Systemic Examination: Chest: Air entry diminished over bases, CVS: Normal, per Abdomen: mild distension (+) and liver was just palpable which was confirmed by ultrasonography.
Provisional diagnosis: Sepsis with hemolytic jaundice (G6PD def)
Intra venous Meropenam, Teicoplanin administered along with BIPAP support and O2 inhalation.
On day two patient’s condition deteriorated. Hemoglobin 5.4 mg/dl and total bilirubin 17.08 mg/dl, s.bilirubin (D) 4.10, s.bilirubin (I) 12.98 mg/dl. Treated with four units of leukocyte reduced packed RBCs. Hemoglobin increased to 8.0 gm/dl but patient started passing black color urine.
Repeat blood grouping, auto control, direct and indirect coombs test were negative.
Final diagnosis: G6PD deficiency with hemolysis.
Patient was administered two cycles of TPE (carried out on Hemonetics Corporation (USA)-MCS+). Total volume processed 9023 ml, total plasma volume extracted 5392 ml, total volume substituted 5393 ml. Color of the extracted product was Black [ Figure 1 ]. Improvement in general condition and parameters noted. Hemoglobin improved to 10.3 gm/dl and Total and Indirect Bilirubin 2.63 gm/dl and 1.53 gm/dl respectively. No trace of hemolysis found. Patient found to be stable and discharged subsequently.

Extracted Plasma
As the patient developed severe hemolysis and the trigger agent of hemolysis was unknown, two cycles of TPE were performed with the aim to remove unknown causative agent of hemolysis.
Plasmapheresis can be used to treat patients with severe hemolysis in the presence of unidentified trigger agent. Though there is no mention of TPE in the treatment of severe hemolysis due to G6PD deficiency in guidelines,[ 2 ] plasmapheresis can be used as an effective tool as demonstrated.
Source of Support: Nil
Conflict of Interest: None declared.
- Case report
- Open access
- Published: 06 May 2008
Kernicterus by glucose-6-phosphate dehydrogenase deficiency: a case report and review of the literature
- Gladys Cossio de Gurrola 1 ,
- Juan José Araúz 1 ,
- Elfilda Durán 1 ,
- Maribel Aguilar-Medina 2 ,
- Rosalío Ramos-Payán 2 ,
- Noemí García-Magallanes 2 ,
- Gerardo Vaca Pacheco 3 &
- Eliakym Arámbula Meraz 2
Journal of Medical Case Reports volume 2 , Article number: 146 ( 2008 ) Cite this article
11k Accesses
15 Citations
3 Altmetric
Metrics details
Introduction
Glucose-6-phosphate dehydrogenase deficiency is an X-linked recessive disease that causes acute or chronic hemolytic anemia and potentially leads to severe jaundice in response to oxidative agents. This deficiency is the most common human innate error of metabolism, affecting more than 400 million people worldwide.
Case presentation
Here, we present the first documented case of kernicterus in Panama, in a glucose-6-phosphate dehydrogenase-deficient newborn clothed in naphthalene-impregnated garments, resulting in reduced psychomotor development, neurosensory hypoacousia, absence of speech and poor reflex of the pupil to light.
Mutational analysis revealed the glucose-6-phosphate dehydrogenase Mediterranean polymorphic variant, which explained the development of kernicterus after exposition of naphthalene. As the use of naphthalene in stored clothes is a common practice, glucose-6-phosphate dehydrogenase testing in neonatal screening could prevent severe clinical consequences.
Peer Review reports
Glucose-6-phosphate dehydrogenase (G6PD) is a housekeeping enzyme that catalyzes the first step in the pentose phosphate pathway, providing reducing power in the form of nicotinamide adenosine dinucleotide phosphate (NADPH). This metabolic pathway is the only source of NADPH in erythrocytes and is therefore the mechanism by which the cell damage caused by oxidative stress is avoided [ 1 , 2 ].
Individuals deficient in G6PD are usually asymptomatic; however, in some cases exposure to chemicals (for example, naphthalene) and drugs (including sulfamides, antipyretics, nitrofurane, primaquine and chloroquine) can induce massive intravascular hemolysis. Among the clinical forms of this enzymatic deficiency are jaundice, acute hemolytic anemia and chronic nonspherocytic hemolytic anemia. A more severe consequence of neonatal hyperbilirubinemia is kernicterus, a neurological syndrome caused by the deposition of bilirubin in the brain tissues, which results in severe consequences and even death [ 2 – 4 ].
G6PD deficiency is an X-linked recessive disease and is the most common human innate error of metabolism, affecting more than 400 million people worldwide [ 5 ]. The G6PD gene is highly polymorphic and more than 140 mutations have been described. In the Mediterranean, Middle East, India, China and Southeast Asia, the distribution of multiple alleles account for the total prevalence. There are no reports concerning the prevalence of this enzymatic deficiency in Panama; however, our preliminary studies indicate a high prevalence of G6PD deficiency in this country (unpublished data).
Here we present a kernicterus case in a G6PD-deficient newborn resulting in severe neurological damage.
Clinical history
A 4-day-old boy was admitted to the Hospital del Niño in Panama. He was the first child of a healthy young woman from a normal vaginal birth at term. He had an Apgar score of 9 at 1 minute and 9 at 5 minutes, a birth weight of 3.5 kg, body length of 53 cm and a 35 cm cephalic circumference. The newborn was hospitalized when clinical examination revealed 4+ jaundice, hypoactivity, hyporexia and generalized tonic-clonic seizures, requiring management with anticonvulsives, phototherapy and exchange transfusion. A history of use of naphthalene-impregnated clothes was recorded. Analysis of clinical symptoms and laboratory tests diagnosed the proband with kernicterus by G6PD deficiency. Despite clinical management, after 5 years the patient presented with reduced psychomotor development, neurosensory hypoacousia, absence of speech and poor reflex of the pupil to light.
Laboratory data
Upon admission the patient presented total bilirubin values of 42.6 mg/dl (41.8 mg/dl from indirect bilirubin), 11.8 g/dl hemoglobin, 8% reticulocytes, O (Rh+) and B (Rh+) blood types for child and mother, respectively, and negative direct Coombs. G6PD testing was reported as deficient, and the quantification of the serum levels of this enzyme was 0.278 U/gHb (normal value range 4.6 to 13.5 U/gHb). Cranial magnetic resonance imaging demonstrated hyperintense basal ganglia lesions on T2-weighted images (Figure 1 ).
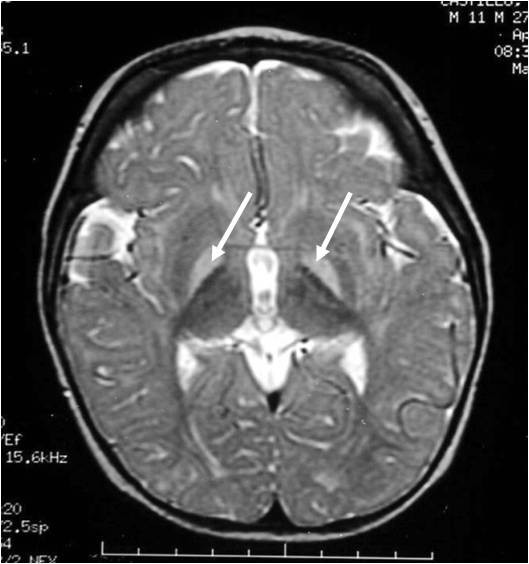
Magnetic resonance imaging of the head . Hyperintense basal ganglia lesions on T2-weighted images.
Mutational analysis
Determination of the G6PD polymorphic variant was achieved by polymerase chain reaction and restriction fragment length polymorphism (PCR-RFLP) as described elsewhere [ 6 ]. Genomic DNA from the patient and one heterozygote G6PD Mediterranean variant control were obtained from heparinized peripheral blood with the salting out method described by Miller et al. [ 7 ]. We used 100 ng of DNA to amplify the region flanking nucleotide 563 containing the polymorphism. Amplified products were then digested with the restriction enzyme Mbo II and the digestion mix was electrophoresed in 10% polyacrylamide gel. Analysis showed the G6PD Mediterranean polymorphic variant genotype (Figure 2 ), which explained the G6PD deficiency phenotype.
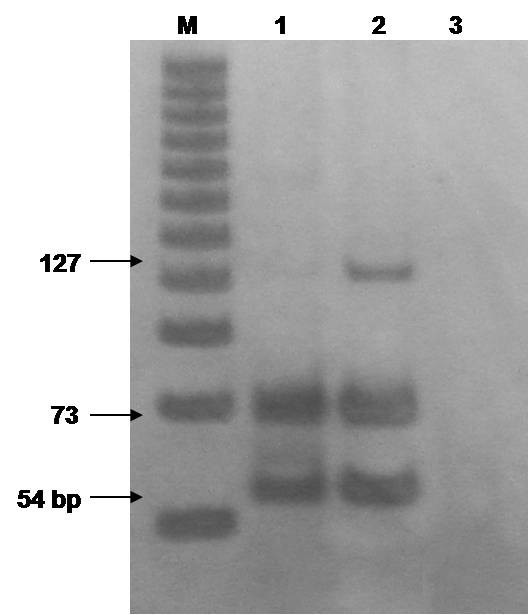
Polymerase chain reaction and restriction fragment length polymorphism determination . The G6PD gene was amplified by polymerase chain reaction and digested with Mbo II . Lane 1, patient; lane 2, heterozygous control; lane 3, non-template control. M, 25 bp molecular marker.
G6PD deficiency is an X-linked recessive disease and is the most common human innate error of metabolism, affecting more than 400 million people worldwide [ 5 ]. At the Hospital del Niño of Panama, we have observed a high prevalence of G6PD deficiency in our patients; however, there are no reports concerning the prevalence of this enzymatic deficiency in this country.
Here we presented the first documented case of kernicterus in Panama in a G6PD-deficient newborn. The proband presented with hyperbilirubinemia (total bilirubin of 42.6 mg/dl) and was treated with phototherapy and two exchange transfusions. G6PD deficiency testing was positive explaining the symptoms and clinical signs. Mutational analysis demonstrated the G6PD Mediterranean polymorphic variant genotype (Figure 2 ).
The use of naphthalene-impregnated clothes prior to the episodes of seizure explained the development of severe jaundice that led to kernicterus. As the use of naphthalene in stored clothes is a common practice, so testing for G6PD as part of neonatal screening could prevent this severe clinical consequence.
Written informed consent was obtained from the patient's next-of-kin for publication of this case report and accompanying images. A copy of the written consent is available for review by the Editor-in-Chief of this journal.
Beutler E: G6PD deficiency. Blood. 1994, 84: 3613-3636.
CAS PubMed Google Scholar
Luzzatto L, Mehta A, Vulliamy T: Glucose-6-phosphate dehydrogenase deficiency. The Metabolic and Molecular Basis of Inherited Disease. Edited by: Scriver ChR, Beaudet AL, Sly WS, Valle D. 2001, New York: McGraw Hill, 4517-4553. 8
Google Scholar
Beutler E: Study of glucose-6-phosphate dehydrogenase: history and molecular biology. Am J Hematol. 1993, 42: 53-58. 10.1002/ajh.2830420111.
Article CAS PubMed Google Scholar
Kaplan M, Beutler E, Ureman HJ, Hammerman C, Levi-Lahad E, Renbaum P, Stevenson DK: Neonatal hiperbilirubinemia in glucose-6-phosphate dehydrogenase deficient heterozygotes. Pediatrics. 1999, 104: 68-74. 10.1542/peds.104.1.68.
Luzzatto L: Glucose-6-phosphate dehydrogenase deficiency: from genotype to phenotype. Haematologica. 2006, 91: 1303-1306.
Vaca G, Arambula E, Monsalvo A, Medina C, Nunez C, Sandoval L, Lopez-Guido B: Glucose-6-phosphate dehydrogenase (G-6-PD) mutations in Mexico: four new G-6-PD variants. Blood Cells Mol Dis. 2003, 31: 112-120. 10.1016/S1079-9796(03)00119-0.
Miller SA, Dykes DD, Polesky HF: A simple salting out procedure for extracting DNA from human nucleated cells. Nucleic Acids Res. 1988, 16: 1215-10.1093/nar/16.3.1215.
Article CAS PubMed PubMed Central Google Scholar
Download references
Acknowledgements
EAM, RRP and MAM are SNI fellows. This work was supported by the General Coordination of Research and Postgraduate (CGIP-PROFAPI) Universidad Autónoma de Sinaloa and CECYT (State Council of Science and Technology) from Sinaloa, México.
Author information
Authors and affiliations.
Servicio de Genética, Hospital del Niño de Panamá, Panama
Gladys Cossio de Gurrola, Juan José Araúz & Elfilda Durán
Laboratorio de Biología Molecular, Doctorado en Biotecnología, Facultad de Ciencias Químico Biológicas, Universidad Autónoma de Sinaloa, Culiacán, Sinaloa, Mexico
Maribel Aguilar-Medina, Rosalío Ramos-Payán, Noemí García-Magallanes & Eliakym Arámbula Meraz
Centro de Investigación Biomédica de Occidente IMSS, Guadalajara, Jalisco, Mexico
Gerardo Vaca Pacheco
You can also search for this author in PubMed Google Scholar
Corresponding author
Correspondence to Eliakym Arámbula Meraz .
Additional information
Competing interests.
The authors declare that they have no competing interests.
Authors' contributions
GCG provided genetic counseling to the parents. JJA and ED collected the data. MAM, EAM and GVP conducted the data analysis, interpreted experiments and revised the manuscript. MAM, RRP, NGM and EAM performed genetic studies and elaboration, and helped to draft the manuscript. All authors read and approved the final manuscript.
Authors’ original submitted files for images
Below are the links to the authors’ original submitted files for images.
Authors’ original file for figure 1
Authors’ original file for figure 2, rights and permissions.
Open Access This article is published under license to BioMed Central Ltd. This is an Open Access article is distributed under the terms of the Creative Commons Attribution License ( https://creativecommons.org/licenses/by/2.0 ), which permits unrestricted use, distribution, and reproduction in any medium, provided the original work is properly cited.
Reprints and permissions
About this article
Cite this article.
Cossio de Gurrola, G., Araúz, J.J., Durán, E. et al. Kernicterus by glucose-6-phosphate dehydrogenase deficiency: a case report and review of the literature. J Med Case Reports 2 , 146 (2008). https://doi.org/10.1186/1752-1947-2-146
Download citation
Received : 07 November 2007
Accepted : 06 May 2008
Published : 06 May 2008
DOI : https://doi.org/10.1186/1752-1947-2-146
Share this article
Anyone you share the following link with will be able to read this content:
Sorry, a shareable link is not currently available for this article.
Provided by the Springer Nature SharedIt content-sharing initiative
- Hemolytic Anemia
- G6PD Deficiency
- Neonatal Screening
- Kernicterus
Journal of Medical Case Reports
ISSN: 1752-1947
- Submission enquiries: Access here and click Contact Us
- General enquiries: [email protected]
A 5-year-old child with hemolytic anemia caused by glucose-6-phosphate dehydrogenase deficiency: a case report
- Bambang Edi Susyanto ,
Bambang Edi Susyanto
Search for the other articles from the author in: Google Scholar | PubMed | BMJ Journal
- Gina Puspita ,
Gina Puspita
- Suryanto Suryanto ,
Suryanto Suryanto
- DOI: https://doi.org/10.15562/bmj.v10i3.2877  |
- Published: 2021-12-30
- Related Content
Introduction : Glucose-6-phosphate dehydrogenase (G6PD) is an enzyme that plays an essential role in the human body cell. It is found in the cytoplasm of cells and functions to prevent cellular damage from reactive oxygen species. G6PD deficiency can be highly variable in its clinical presentation. It can cause acute or chronic hemolytic anemia and hyperbilirubinemia. Severe hemolytic can cause mortality in children. So, it is crucial to be aware during the diagnosis of hemolytic anemia at any age. The hemolytic occurred is usually precipitated by exposure to medication or intake of fava bean. This report is aimed to describe the diagnosis and treatment of a child who appears with acute hemolytic anemia due to favism.
Case description: A 5-year-old boy came to the emergency department with a chief complaint of pale and fatigue since a day before admission in PKU Muhammadiyah Gamping Hospital. No family history of hemolytic anemia or parental consanguinity. He appeared icteric and with severe anemia. We found that he consumed fava beans twelve hours prior to the onset of the symptoms was reported. The laboratory findings were as follows: Hb 4.9 g/dl, total bilirubin 6.17 mg/dl, indirect bilirubin 5.49 mg/dl and a negative Coomb’s test result then obtained. The patient then is transfused with PRC, and the level of Hb became 9.2 g/dl and total bilirubin 0.2 mg/dl. The blood smear result was met hemolytic anemia and bacterial infection. The level of G6PD result obtained four days after was low, 7.5 U/gr Hb (10.0-14.2).
Conclusion: The severe hemolytic anemia in the patient was proven caused by G6PD deficiency.
- Zhao X, Li Z, Zhang X. G6PD-MutDB: a mutation and phenotype database of glucose-6-phosphate (G6PD) deficiency. J Bioinform Comput Biol. 2010;8 Suppl 1:101–9.
- Ge T, Yang J, Zhou S, Wang Y, Li Y, Tong X. The Role of the Pentose Phosphate Pathway in Diabetes and Cancer [Internet]. Vol. 11, Frontiers in Endocrinology . 2020. p. 365. Available from: https://www.frontiersin.org/article/10.3389/fendo.2020.00365
- Nkhoma ET, Poole C, Vannappagari V, Hall SA, Beutler E. The global prevalence of glucose-6-phosphate dehydrogenase deficiency: a systematic review and meta-analysis. Blood Cells Mol Dis. 2009;42(3):267–78.
- Kaban RK, Wijaya V. Prevalence of glucose-6-phosphate dehydrogenase (G6PD) deficiency in neonates in Bunda Women’s and Children’s Hospital, Jakarta, Indonesia. Paediatr Indones. 2011;51(1):29–33.
- Pinto A, MacDonald A, Cleto E, Almeida MF, Ramos PC, Rocha JC. A case report of a 4-year-old child with glucose-6-phosphate dehydrogenase deficiency: An evidence based approach to nutritional management. Turk J Pediatr. 2017;59(2):189–92.
- Sackey K. Hemolytic anemia: Part 1. Pediatr Rev. 1999;20(5):152–8; quiz 159.
- Noronha SA. Acquired and Congenital Hemolytic Anemia. Pediatr Rev. 2016;37(6):235–46.
- Chen Y, Xiu W, Dong Y, Wang J, Zhao H, Su Y, et al. Mutation of glucose-6-phosphate dehydrogenase deficiency in Chinese Han children in eastern Fujian. Medicine (Baltimore). 2018;97(30):e11553.
- Cappellini MD, Fiorelli G. Glucose-6-phosphate dehydrogenase deficiency. Lancet (London, England). 2008;371(9606):64–74.
- Luzzatto L, Arese P. Favism and Glucose-6-Phosphate Dehydrogenase Deficiency. N Engl J Med. 2018;378(1):60–71.
- Frank JE. Diagnosis and management of G6PD deficiency. Am Fam Physician. 2005;72(7):1277–82.
How to Cite
Search panel.
- Bali Medical Journal, Bali-Indonesia
- +62 812-3999-2269
- [email protected]
- Journal Information
- Editorial Board
- Abstracting & Indexing
- Privacy Statement
- Author Guidelines
- Open-Access Licence

Select a Community
- MB 1 Preclinical Medical Students
- MB 2/3 Clinical Medical Students
- ORTHO Orthopaedic Surgery
Are you sure you want to trigger topic in your Anconeus AI algorithm?
You are done for today with this topic.
Would you like to start learning session with this topic items scheduled for future?
G6PD Deficiency
.jpg)
- A 13-year-old African-American boy presents to his pediatrician for jaundice. He states that he had tried some new Mediterranean food at school today. A day later, he felt fatigued and tired easily after minimal activity and reported back pain. On physical exam, he has scleral icterus. His hemoglobin was found to be 8 g/dL with increased reticulocyte count, increased indirect bilirubin, and decreased haptoglobin. He is given 1 unit of blood.
- glucose-6-phosphate dehydrogenase (G6PD) deficiency is an X-linked genetic disorder that causes an intrinsic hemolytic anemia
- 7.1% worldwide
- most common enzyme disorder of erythrocytes
- more severe in males than females
- sub-Saharan Africa
- Middle East
- Southeast Asia
- Mediterranean regions
- Pacific islands
- G6PD gene encoding the G6PD enzyme
- generates nicotinamide dinucleotide phosphate (NADPH), which protects red blood cells against oxidative stress
- in red blood cells (without mitochondria), this pathway is the only source of NADPH
- nitrofurantoin
- sulfa drugs
- fava bean ingestion
- precipitation of hemoglobin (manifested as Heinz bodies)
- erythrocyte membrane damage, both extravascular and intravascular hemolysis
- G6PD deficiency thought to decrease risk of severe malaria
- neonatal hyperbilirubinemia on day 2-4
- kernicterus is rare
- complete blood count and reticulocyte count
- bite cells (degmacytes)
- Heinz bodies
- ↑ indirect bilirubin
- ↓ haptoglobin
- ↑ lactate dehydrogenase
- hemoglobinuria
- most sensitive
- methemoglobin reduction test
- a normal G6PD level immediately after hemolysis does not rule out G6PD deficiency
- spectrophotometry analysis
- molecular diagnosis (DNA analysis)
- jaundice at birth (rather than delayed onset of jaundice)
- normal G6PD enzyme activity
- spherocytosis seen on peripheral blood smear
- avoid oxidative stressors
- if hemoglobin
- neonatal hyperbilirubinemia
- Recurrence of acute hemolysis
- typically asymptomatic until exposed to oxidative stressors
- - G6PD Deficiency
Please Login to add comment
Thank you for visiting nature.com. You are using a browser version with limited support for CSS. To obtain the best experience, we recommend you use a more up to date browser (or turn off compatibility mode in Internet Explorer). In the meantime, to ensure continued support, we are displaying the site without styles and JavaScript.
- View all journals
- My Account Login
- Explore content
- About the journal
- Publish with us
- Sign up for alerts
- Open access
- Published: 06 April 2017
Association of glucose-6-phosphate dehydrogenase deficiency and malaria: a systematic review and meta-analysis
- Evaristus Chibunna Mbanefo 1 , 2 na1 ,
- Ali Mahmoud Ahmed 3 na1 ,
- Afaf Titouna 1 na1 ,
- Ahmed Elmaraezy 3 na1 ,
- Nguyen Thi Huyen Trang 4 ,
- Nguyen Phuoc Long 5 ,
- Nguyen Hoang Anh 6 ,
- Tran Diem Nghi 6 ,
- Bui The Hung 5 ,
- Mai Van Hieu 6 ,
- Nguyen Ky Anh 5 ,
- Nguyen Tien Huy 7 , 8 &
- Kenji Hirayama 1
Scientific Reports volume 7 , Article number: 45963 ( 2017 ) Cite this article
11k Accesses
71 Citations
3 Altmetric
Metrics details
Glucose-6-Phosphate Dehydrogenase (G6PD) deficiency overlaps with malaria endemicity although it predisposes carriers to hemolysis. This fact supports the protection hypothesis against malaria. The aim of this systematic review is to assess the presence and the extent of protective association between G6PD deficiency and malaria. Thirteen databases were searched for papers reporting any G6PD alteration in malaria patients. Twenty-eight of the included 30 studies were eligible for the meta-analysis. Results showed absence of negative association between G6PD deficiency and uncomplicated falciparum malaria (odds ratio (OR), 0.77; 95% confidence interval (CI), 0.59–1.02; p = 0.07). However, this negative association happened in Africa (OR, 0.59; 95% CI, 0.40–0.86; p = 0.007) but not in Asia (OR, 1.24; 95% CI, 0.96–1.61; p = 0.10), and in the heterozygotes (OR, 0.70; 95% CI, 0.57–0.87; p = 0.001) but not the homo/hemizygous (OR, 0.70; 95% CI, 0.46–1.07; p = 0.10). There was no association between G6PD deficiency and total severe malaria (OR, 0.82; 95% CI, 0.61–1.11; p = 0.20). Similarly, there was no association with other malaria species. G6PD deficiency can potentially protect against uncomplicated malaria in African countries, but not severe malaria. Interestingly, this protection was mainly in heterozygous, being x-linked thus related to gender.
Similar content being viewed by others
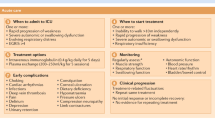
Diagnosis and management of Guillain–Barré syndrome in ten steps
Sonja E. Leonhard, Melissa R. Mandarakas, … Bart C. Jacobs
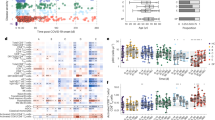
Iron dysregulation and inflammatory stress erythropoiesis associates with long-term outcome of COVID-19
Aimee L. Hanson, Matthew P. Mulè, … Kenneth G. C. Smith
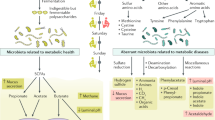
Gut microbiota in human metabolic health and disease
Yong Fan & Oluf Pedersen
Introduction
Glucose-6-phosphate dehydrogenase (G6PD) deficiency is an incomplete hereditary X-linked hemolytic disease. This enzymopathy is widespread in the tropics and subtropics, synchronizing with endemic or formerly endemic malaria 1 ; which suggests that G6PD deficiency may have arisen, spread, or maintained in frequency through natural selection by malaria 2 , 3 . Although it is hard to detect G6PD deficient patients as most affected people are asymptomatic until they are exposed to triggers 4 , more than 400 million people are thought to be G6PD deficient 2 .
G6PD catalyzes the reaction in the pentose phosphate pathway that generates reduced form of NADPH, which is in turn responsible for glutathione (GSH) homeostasis 5 . GSH is an antioxidant, and together, these processes make cells more able to resist and control oxidative stress 2 . Inability of the erythrocytes to maintain GSH homeostasis results in oxidative stress and affects the integrity of the RBCs, giving rise to hemolysis. Optimum RBC redox status is required by malaria parasites for their survival, replication, and development 6 . This factor is diminished in G6PD deficient RBCs, supporting the protection hypothesis.
Although circumstantial evidence accumulated to support the hypothesis that G6PD deficiency is protective against severe fatal malaria 1 , 7 , 8 , 9 ; there have been several arguments for 7 , 10 , 11 , 12 and against 13 , 14 , 15 . Some researchers also argued that perhaps malaria may not be the only factor affecting the deficiency gene locus 16 . It remains to be clarified whether a direct association exists between G6PD deficiency and protection from malaria 11 , 17 . This systematic review and meta-analysis was undertaken to integrate all the informative studies focusing on the association between malaria and genetically determined G6PD deficiency to assess the presence and the extent of this association.
Study selection
The initial search on public databases yielded 1600 study reports after excluding duplicates. Titles and abstracts screening resulted in exclusion of 1364 studies based on the exclusion criteria. Only 236 studies were considered eligible for inclusion for full-text review. Further 197 studies were excluded after full-text reading. A total of 39 studies were included, which comprises 49 data sets due to multi-center study reports. However, nine studies whose data either cannot be extracted, overlapped, or combined with the rest of the data, and thus were excluded. Among the 30 included studies, the species of Plasmodium are only Plasmodium falciparum in 17 studies 3 , 5 , 7 , 10 , 11 , 18 , 19 , 20 , 21 , 22 , 23 , 24 , 25 , 26 , 27 , 28 , 29 , only Plasmodium vivax in one study 30 , and combined species ( P. falciparum with P. vivax, P. falciparum with P. malariae, P. vivax with P. malariae , and P. falciparum with P. vivax and P. malariae ) in eight studies 14 , 15 , 31 , 32 , 33 , 34 , 35 , 36 . Jalloh 2004 32 , Tantular 1999 36 , and Kruatrachue 1962 15 reported separate data for P. vivax which meta-analyzed with that of Leslie 2010 30 . The species cannot be determined in four studies 37 , 38 , 39 , 40 . Finally, a total of 28 studies containing 34 datasets were included in the quantitative data synthesis and meta-analysis ( Fig. 1 ).
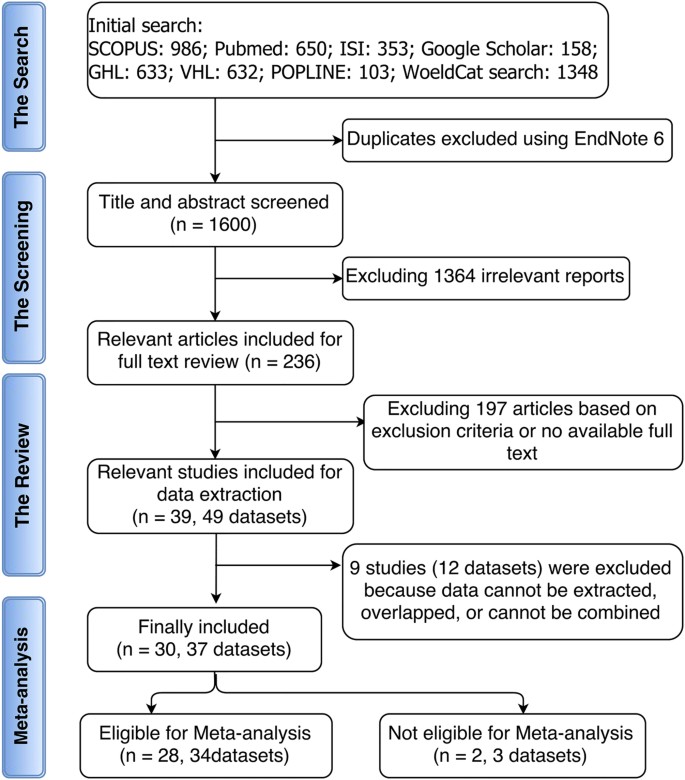
The database search using the search strategy was cleaned up to exclude duplicates. Titles and abstracts were initially screened to include all studies (published before January 2014) describing any association between G6PD deficiency and malaria. In addition to not meeting the inclusion criteria, reviews, case studies, editor correspondences, and studies whose data could not be retrieved were excluded during the full-text review.
Characteristics of included studies
The characteristics of the 30 included studies are described in details in Supplementary Table S1 . The extracted items are described in the methodology section. Seventeen (17) studies with 21 datasets were on patients in Africa, 11 studies containing 14 datasets were performed in Asia while one study each was reported from Brazil and Papua New Guinea. All included studies used prospective method for data collection. Seven (7) studies were case-control studies; one was randomized double-blinded clinical trial while the rest were cross-sectional studies. Sixteen (16) studies were performed on children and infants only, while the other studies included children and adults. Only three studies used random sampling, the other adopted consecutive method for patient recruitment.
Association of G6PD deficiency with protection from uncomplicated P. falciparum malaria
A meta-analysis was performed to assess the frequencies of G6PD deficiency between uncomplicated P. falciparum malaria and malaria negative individuals. The odds ratios (OR) from 19 datasets obtained from 14 studies 3 , 5 , 7 , 10 , 14 , 15 , 18 , 20 , 22 , 24 , 29 , 31 , 32 , 36 were pooled for this meta-analysis. There was significant heterogeneity among the studies; thus, random effect model was applied for the meta-analysis. The combined OR revealed absence of negative association between G6PD deficiency and uncomplicated malaria (OR, 0.77; 95% confidence interval (CI), 0.59–1.02; n, 19; p = 0.07) ( Fig. 2a ). There was publication bias in favor of studies with negative association ( Fig. 2b ). When the cut and fill method as proposed by Duval et al . 41 was applied, the condition negative association was further lost (OR, 0.88; 95% CI, 0.66–1.17; n, 22; p = 0.435).
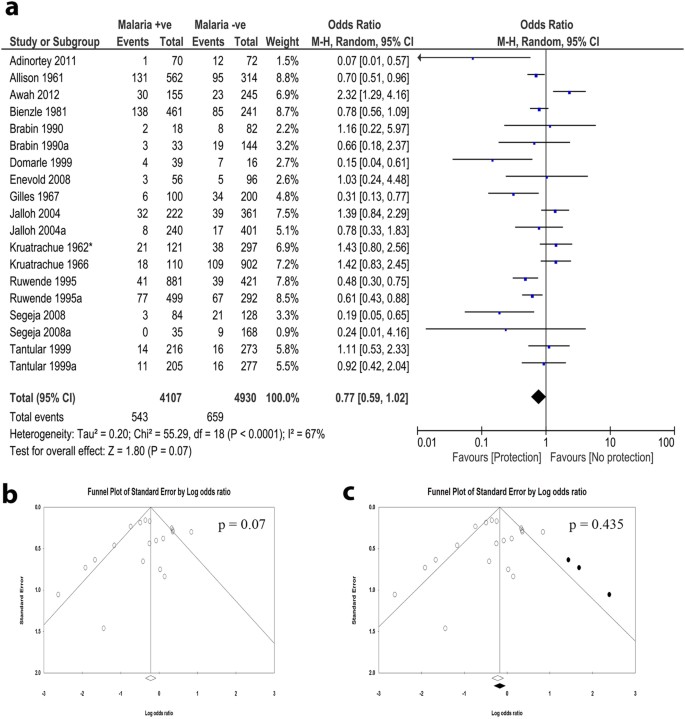
( a ) The forest plot of meta-analysis assessing the association between G6PD deficiency and prevalence of P. falciparum malaria. The comparators were malaria negative individuals. The observed results revealed absence of negative association between G6PD deficiency and P. falciparum malaria prevalence. (b) Funnel plot revealed the presence of publication bias towards negative association. (c) Trim and fill analysis led to further tilting away from the negative association, confirming the presence of publication bias among the included studies.
Sensitivity analysis was performed to investigate the effect of a single study on the outcome measures. We observed significant effects of single studies on the meta-analysis result. Interestingly, the exclusion of any of four studies 10 , 14 , 15 , 32 led to appearance of significant negative association in the meta-analysis ( Supplementary Table S2 ). The cumulative meta-analysis also showed that the inclusion of single studies, especially four studies 10 , 14 , 18 , 31 , affected the outcome effect measures ( Supplementary Table S3 ).
Subgroup analysis shows that protection from uncomplicated P. falciparum malaria is significantly observed in heterozygotes
Subgroup analysis was performed to identify the effect of population subgroups on the meta-analysis. We grouped data into genotypes by separately analyzing studies that reported different genotypes. When homo/hemizygous or heterozygous were analyzed separately, the outcome effect measures were significant for heterozygous (OR, 0.70; 95% CI, 0.57–0.87; n, 6; p = 0.001), but not for homo/hemizygous (OR, 0.70; 95% CI, 0.46–1.07; n, 10; P = 0.10) indicating that the association is only present in heterozygous subgroup ( Fig. 3a ). However, there was a publication bias observed by funnel plot in favor of the protection ( Fig. 3b ). Trim and fill methods of Duval indicated that this publication bias is so minimal to affect the significant association in the heterozygous subgroup (OR, 0.71; 95% CI, 0.57–0.88; n, 7; P = 0.002) ( Fig. 3c ). Also, sensitivity analysis showed there was no single study effect on this association ( Supplementary Table S4 ). Subgroup analysis by age revealed negative association with children only (OR, 0.72; 95% CI, 0.53–0.98; n, 9; P = 0.04) but not with children and adult subgroup ( Supplementary Fig. S1 ). When the genders were analyzed separately, the significant association was only present with female subgroup (OR, 0.70; 95% CI, 0.50–0.98; n, 7; P = 0.04) ( Supplementary Fig. S2 ). This corroborates the significant association for heterozygous subgroup since G6PD enzymopathy is sex-linked.
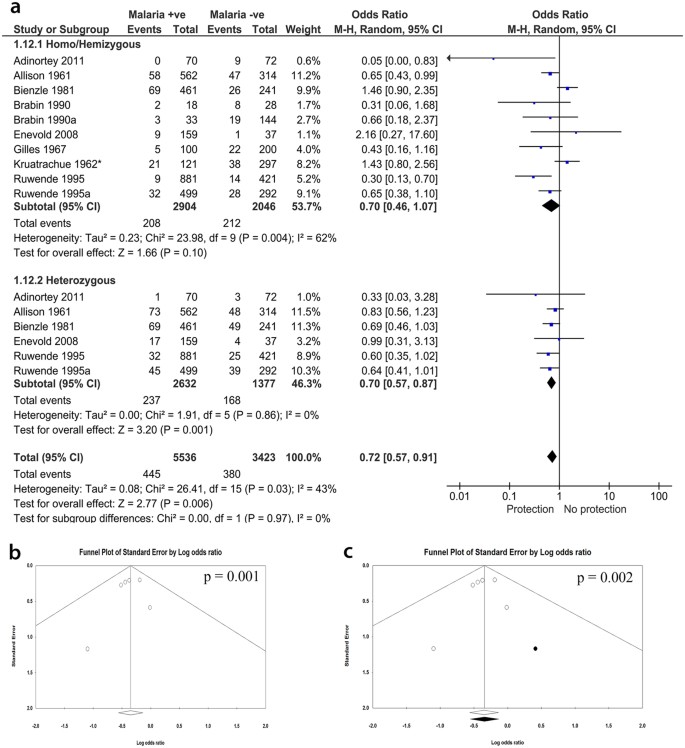
(Subgroup analysis by genotypes): homo/hemi or heterozygous). ( a ) Present the meta-analysis forest plot of the association of G6PD deficiency and falciparum malaria, grouped by genotypes. The heterozygous females were compared to a combined group comprising hemizygous males and homozygous female. There was a strong negative association between G6PD deficiency and malaria for the heterozygous. ( b ) Funnel plot of the heterozygous subgroup represents presence of publication bias towards negative association. ( c ) Trim and fill analysis indicate that the publication bias is too minimal to affect the significant negative association.
Subgroup analysis by the location of the participants
Interestingly, when the studies were grouped according to the continents where the studies were performed, we observed that the negative association between G6PD and malaria was only observed for studies performed in Africa (OR, 0.59; 95% CI, 0.40–0.86; n, 11; P = 0.007) ( Fig. 4a ). The studies performed in Asia showed no significant association (OR, 1.24; 95% CI, 0.96–1.61; n, 6; P = 0.1) ( Fig. 4a ). However, although sensitivity analysis did not show any single study or small study effect on the observed negative association, funnel plot showed evidence of publication bias in favor of studies with negative association. When the cut and fill method as proposed by Duval et al . 41 was applied, the statistical significance of the association was lost (OR, 0.74; 95% CI, 0.49–1.10; n, 14; P = 0.122) ( Fig. 4b and c ).
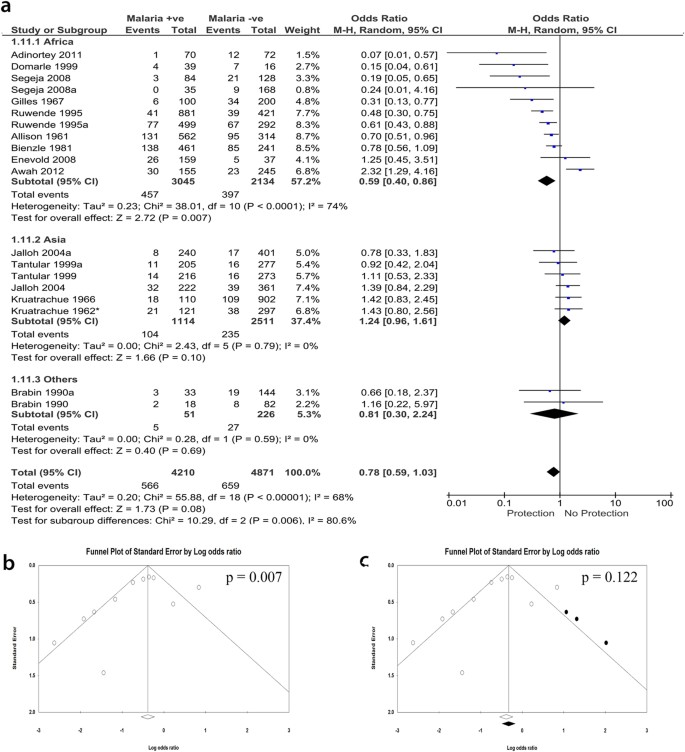
(Subgroup analysis by continents where each study was performed (Africa, Asia, or others (Papua New Guinea)). ( a ) The forest plot of the subgroup analysis showing an association of G6PD deficiency and falciparum malaria, sub-grouped into the continents where study site is located. A statistically significant negative association was observed from the meta-analysis of studies on African subjects. No association was observed for studies performed in Asia. Also, the two data sets of a study from Papua New Guinea showed no association. ( b ) Funnel plot of studies performed in African. ( c) Trim and fill analysis showing presence of publication bias in studies performed in Africa.
Association of G6PD deficiency with severe falciparum malaria
Having identified association between G6PD deficiency and mild P. falciparum malaria, we assessed whether G6PD deficiency is also associated with protection from severe P. falciparum malaria. For this analysis, the comparator was uncomplicated falciparum malaria cases. Different severe falciparum malaria symptoms were considered together and then separately in relation to uncomplicated malaria. There was no association between G6PD deficiency and severe falciparum malaria, in relation to uncomplicated malaria (OR, 0.82, 95% CI, 0.61 to 1.11; n, 114; P = 0.20) ( Supplementary Fig. S3 ). Even when the types of severe falciparum malaria were considered separately, we observed significant negative association between G6PD deficiency and hyperparasitemia (OR, 0.73; 95% CI, 0.56–0.95; n, 8; P = 0.02), but not between G6PD deficiency and severe anemia or cerebral malaria ( Supplementary Fig. S3 ). When doing sensitivity analysis to investigate the effect of one study removal on the observed negative association between G6PD and hyperparasitemia, there was a significant effect of single studies on the observed results. Exclusion of one of two studies, Gilles 1967 24 and Allison 1961 7 , led to loss of the observed significant association ( Supplementary Table S5 ). The analysis in this section did not show evidence of publication bias as evident in the good symmetry of the funnel plots, except for the hyper-parasitemia, with which, we observed a minimal publication bias that did not affect the results ( Supplementary Fig. S3 ).
G6PD deficiency was not associated with malaria due to other species of Plasmodium
We also investigated the association of G6PD with other species of Plasmodium including P. vivax, P. malariae or combined co-infections. For P. vivax malaria, the OR from six datasets obtained from four papers 15 , 30 , 32 , 36 showed that there was no association between G6PD and vivax malaria (OR, 0.87; 95% CI, 0.28–2.66; n, 6; P = 0.81), however, the two non-analyzable papers, Louicharoen 2009 34 and Khim 2013 33 , reported significant association of G6PD with P. vivax . Similarly, there was no negative association between G6PD and malaria in patients infected with P. malariae, as reported by three datasets from two papers 32 , 36 (OR, 1.83; 95% CI, 0.47–7.06; n, 3; P = 0.38) ( Fig. 5a and c ).
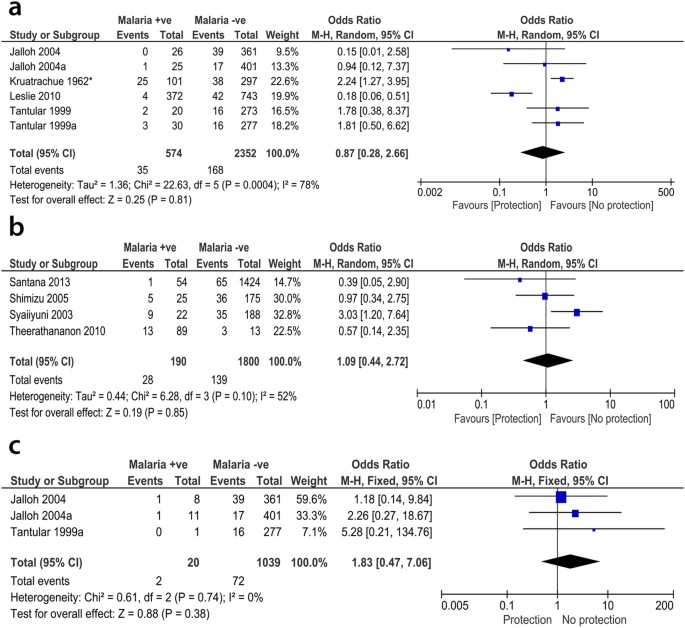
There was no negative association between G6PD and P. vivax ( a ), P. malariae ( c ), or even nondetermined species ( b ).
When combined co-infection with both P. falciparum and P. vivax was considered, the OR from five datasets derived from three papers 14 , 32 , 36 also showed no negative association (OR, 0.95; 95% CI, 0.50–1.80; n, 5; P = 0.87). Besides the previous co-infection, the OR from five datasets of three papers 32 , 35 , 36 also indicated no association with co-infection by P. falciparum and P. malariae (OR, 1.56; 95% CI, 0.29–8.34; n, 5; P = 0.60). Similarly, the pooled OR from four datasets reported in Jalloh 2004 32 and Tantular 1999 36 showed no association when combined P. vivax and P. malariae (OR, 1.17; 95% CI, 0.22–6.35; n, 3; P = 0.85) or combined P. falciparum, P. vivax, and P. malariae (OR, 1.90; 95% CI, 0.42–8.51; n, 4; P = 0.40) co-infections were considered ( Supplementary Fig. S4 ). We could not determine the species of Plasmodium reported in four analyzable studies 37 , 38 , 39 , 40 . Nevertheless, the pooled OR from these studies did not show significant association (OR, 1.09; 95% CI, 0.44–2.72; n, 2; P = 0.85) ( Fig. 5b ).
We observed absence of negative association between G6PD deficiency not only with uncomplicated falciparum malaria but also with severe malaria ( Fig. 2 ). However, single studies may have dramatically affected this analysis and inference. For instance, the exclusion of any of four studies 10 , 14 , 15 , 32 led to appearance of negative association in the meta-analysis. There was also evidence of publication bias in favor of negative association (protection). Only one study by Adinortey et al . 5 was published in a non-peer-reviewed journal among the included studies, however, it does not affect the meta-analysis as shown in the sensitivity analysis. Similarly, there was no significant association between G6PD deficiency and other species of malaria, including P. vivax and P. malariae or combinations of any two or all the three species. Due to the insufficient number of reports on these species, we could not make any evident conclusion on the exact relationship especially after considering the results of the two non-analyzable papers. Therefore, more studies investigating the association between these species and G6PD deficiency are highly recommended. A negative association was found in studies performed in Africa as compared to Asia and other continents, albeit with presence of publication bias. These results may provide evidence in support of the G6PD-malaria protection hypothesis in Africa. G6PD deficiency is endemic in Africa and the African G6PD deficient patients have relatively higher enzyme activity and milder consequences than Mediterranean or Asian patients 3 , 42 . This may be one possible explanation the observed G6PD associated protect from falciparum malaria in Africa. The population history, archeology, and human genetics indicate that the age of this selection by malaria is more than 5,000 years old in Africa 3 , 43 . Moreover, the frequencies of deficiency alleles in most G6PD deficient populations in endemic areas do not exceed 40% 3 , 44 . Interestingly, the pooled data from Asian countries show no significant association. There may be a confounding relationship between location and the observed negative association in the children subgroup because of the skewing effect of African studies in favor of negative association ( Supplementary Fig. S1 ) 14 , 15 , 39 , 40 . This suggests that other than endemicity, ethnicity could modify the effect of G6PD deficiency in the study. While the female homozygous and male hemizygous genotypes showed no association with uncomplicated malaria protection, the female heterozygous genotype showed highly significant association with protection from malaria. Unlike in homo/hemizygous subgroup, the association observed for heterozygous subgroup had no indication of heterogeneity or publication bias. This association with heterozygous females is highly supported by the study of Uyoga et al . 45 which also indicated that hemizygous males are not only unprotected from malaria, but may be at high risk of sever malaria. This is due to the fact that G6PD-deficient red blood cells are prone to early destruction by oxygen free radicals 46 . Usanga and Luzzatto hypothesized a prestigious mechanism that explains the association with heterozygous females 47 . They observed that the parasite undergoes habitual changes when passing through successive G6PD-deficient red cells in order to be more adaptive. These changes resulted in production of the parasite’s own enzyme which in turn resulted in survivability and replication of the parasite 48 . As the heterozygous females are genetically mosaic, the parasite failed to survive 47 . Supportingly, when subgrouping the patients according to the enzymatic activity, our analysis revealed that the activity of the enzyme has no effect on the association, although only two analyzable papers reported the enzymatic activity for P. falciparum .
Studies have demonstrated the presence of G6PD variants in geographically diverse locations, endemic or formerly endemic for malaria; suggesting the likelihood of selection by malaria 2 . However, if malaria were the sole selecting factor on the G6PD gene with no other deleterious factor, an increase in the proportion of the genotype conferring G6PD enzymopathy would be expected to replace the normal allele 1 , 49 . The absence of clear evidence of this selection supports the hypothesis that the net protection observed is dependent on the balance between the malaria selection and other deleterious effects of G6PD enzymopathy 16 . It further confirms that there may be more than one selection factors on this gene, with the effect of the advantageous factors (e.g. malaria) countering the effects of factors that confer net disadvantage (e.g. hemolysis) 7 , 8 , 16 . As a matter of fact, homozygous females and hemizygous males are more severely affected by the hemolytic effects of G6PD deficiency 45 , 49 . This may, therefore, explain the better protection from malaria in the heterozygous females.
Conversely, G6PD deficiency was not associated with protection from severe falciparum malaria. Severe malaria is characterized by severe anemia, hyperparasitemia, and cerebral malaria. The net negative odds ratios observed for severe anemia and cerebral malaria were not statistically significant. Peculiarly, the hyperparasitemia showed significant negative association with G6PD deficiency in relation to low parasitemia. There was minimal publication bias in favor of protection that did not affect the significant association. Association with hyperparasitemia may however not only indicate severity of infection but also reinfection rate. These severity factors are associated with or arguably exacerbated by hemolysis, a characteristic feature of G6PD deficiency. However, the hemolytic effect of G6PD deficiency is self-limiting, affecting mainly older erythrocytes than the newly formed ones; thereby establishing a balance between the factors. Thus, the concept of protection from severe malaria or absence thereof remains conflicting 10 , 17 , 50 .
The inherent disadvantage of G6PD deficiency due to the associated hemolysis could be one of the factors that account for the absence of consensus on the G6PD-malaria protection hypothesis. Also, the presence of other factors that could protect from malaria, especially hemoglobinopathies like sickle cell anemia (HbS) which also overlaps with G6PD and malaria endemicity, is considered one of the major confounders of our analysis and limitations of this study.
To recapitulate, we inferred from this analysis that G6PD deficiency may not generally protect against uncomplicated falciparum malaria, P. vivax, or P. malariae . However, the analysis was sensitive to the effect of individual studies exclusion of which resulted in negative association with uncomplicated falciparum malaria, albeit with evidence of publication bias in favor of malaria protection. The protection was found only in African countries but not in Asian. Comparing the genotypes, the negative association was found only in heterozygous females, but not in a combined group comprising homozygous females and hemizygous males. Similarly, the negative association was present in studies including only children but not with combined group comprising children and adults. With the exception of hyperparasitemia, G6PD deficiency showed no association with severe malaria. Further studies are needed to investigate the mechanism of association with heterozygous females.
Registration of study protocol
This study was performed according to the recommendations of the PRISMA statement 51 during all steps of the study. The protocol for this study was prepared prior to the start of the study and was registered in PROSPERO with identification number CRD42014007282.
Study eligibility criteria
Only original articles that report the association of any G6PD alteration in enzymatic activity and genetic variant and malaria were included. There was no restriction made with respect to language, publication year and study design. Case reports, reviews, theses, conference proceedings were excluded. Two reviewers independently extracted the data and subsequently verified by two other reviewers. Any disagreements were resolved by discussion and consensus between the reviewers and a third party, if necessary.
Search strategy
Thirteen electronic databases were searched including; PubMed, Scopus, Google Scholar, ISI, WHO Global Health Library (GHL), Cochrane Review Library, IBECS, POPline, Virtual Health Library (VHL), WorldCat, New York Academy of Medicine Grey Literature Report, System for Information on Grey Literature in Europe (SIGLE), and African Journals Online databases. Searches were conducted to find relevant studies published before January 2014. The initial search strings were done using PubMed and Scopus databases using the broad search term: “((Glucose-6-phosphate dehydrogenase OR G6PD) AND (Malaria[Mesh] OR Antimalarial[Mesh]) and (INDEXTERMS (glucose-6-phosphate dehydrogenase OR G6PD) AND (malaria OR antimalarial))”. Subsequently, this search string was modified to contain search terms suitable for each database. The details of search strategy and terms used in each database are presented in Supplementary File S1 .
Study inclusion criteria and study selection
All original studies (published before January 2014) describing association between G6PD deficiency and malaria were included in this systematic review. All eligible studies irrespective of publication type, study design, language and publication date were considered in the qualitative systematic review. There was no restriction on certain population, age, race, ethnicity, or geographic area. Only variables reported by two or more studies and whose primary data can be extracted were included in the meta-analysis. We limited included studies to those performed on human subjects. Reviews, case studies, case series, editor correspondences, news, letters, book chapters and studies whose data could not be reliably retrieved or extracted were excluded. We omitted studies whose data is overlapped with another included study. Two reviewers independently performed initial screening and study selection. Preliminary assessment of the title and abstracts was performed to identify relevant articles. Thereafter, full texts of eligible articles were downloaded and reviewed for qualitative analysis and potential inclusion in the data synthesis. Inclusion of a study by both reviewers was conclusive while discrepancies and disagreements as regards study eligibility were resolved by discussion and/or consensus with a third reviewer.
Data extraction
The data extracted from the included reports include: the first author, year of publication year of data, study design, data collection (prospective or retrospective), country and city of origin, characteristics of participant population (gender, age, and the type of malaria), method for genetic variants detection, number of included individuals (case and control groups), outcomes and times of evaluation. When there was no data available or if there were obvious errors (such as typographical errors, incorrect calculation, or incorrect factors designations) in the original publication, two successive emails were sent to corresponding authors for clarification. When no clarification or genotype information was obtained after at least two emails, we will report such studies as “no data available”. Identification and elimination of potentially overlapped datasets were done under extensive care, especially for multiple reports by the same group reporting the same factors on the same subjects.
Meta-analyses
Meta-analysis to pool data from eligible studies was performed on both RevMan v5.2 52 and Comprehensive Meta-Analysis v2 software. 2 × 2 contingency tables were generated and the odds ratio with the corresponding 95% CI were calculated for dichotomous outcomes. For outcomes with continuous variables, the input data were mean and standard deviation (SD) with the standardized mean difference (SMD) as the effect measure. When standard deviation was not available, it was computed with the calculator function in RevMan v5.2 by inputting other supplied data (e.g. mean, standard error of mean, p -value etc.), if available. Forest plots showing the respective OR or SMD with their corresponding 95% CI for each dataset and for the pooled data were generated. The overall effect was measured by Z-statistics with statistical significance set at p < 0.05. More clarifications and in-depth analysis were achieved through subgroup analysis.
Test of heterogeneity between studies
The Cochrane Q ( Chi 2 test) and I 2 statistics 53 were used to assess inconsistency among studies. For Chi 2 test of heterogeneity, statistical significance was set as p < 0.10. Fixed-effects model was adopted when there was a lack of significant heterogeneity (in Chi 2 , p > 0.10 or I 2 values < 50%), otherwise heterogeneity among studies is assumed and random-effects model with weighting of the studies was applied 54 .
Sensitivity analysis and assessment of publication bias among studies
For sensitivity analysis, each individual study in each meta-analysis was sequentially excluded in the analysis to examine the effect of a single study on the outcome of the meta-analysis. Begg’s funnel plots were used to initially assess publication bias across the reviewed studies 55 , 56 . The funnel plots were created by plotting the OR against the standard error of the logarithm of OR. When there was a publication bias, cut and fill method as proposed by Duval and Tweedie 41 was conducted to indicate the effect of this publication bias on the estimated effect size.
Additional Information
How to cite this article : Chibunna Mbanefo, E. et al . Association of glucose-6-phosphate dehydrogenase deficiency and malaria: a systematic review and meta-analysis. Sci. Rep. 7 , 45963; doi: 10.1038/srep45963 (2017).
Publisher's note: Springer Nature remains neutral with regard to jurisdictional claims in published maps and institutional affiliations.
Allison, A. C. Malaria and glucose-6-phosphate dehydrogenase deficiency. Nature 197 , 609–609 (1963).
ADS CAS PubMed Google Scholar
Ruwende, C. & Hill, A. Glucose-6-phosphate dehydrogenase deficiency and malaria. Journal of molecular medicine 76 , 581–588 (1998).
CAS PubMed Google Scholar
Ruwende, C., Khoo, S. C., Snow, R. W. & Yates, S. N. R. Natural selection of hemi-and heterozygotes for G6PD deficiency in Africa by resistance to severe malaria. Nature 376 , 246 (1995).
Brandt, O., Rieger, A., Geusau, A. & Stingl, G. Peas, beans, and the Pythagorean theorem–the relevance of glucose‐6‐phosphate dehydrogenase deficiency in dermatology. JDDG: Journal der Deutschen Dermatologischen Gesellschaft 6 , 534–539 (2008).
PubMed Google Scholar
Adinortey, M. B. et al. The significance of glucose-6-phosphate dehydrogenase deficiency in plasmodium falciparum malaria: A case study of patients visiting the Central Regional Hospital, Cape Coast, Ghana. World Journal of Medical Sciences 6 , 126–130 (2011).
Google Scholar
Vega-Rodríguez, J. et al. The glutathione biosynthetic pathway of Plasmodium is essential for mosquito transmission. PLoS Pathog 5 , e1000302 (2009).
PubMed PubMed Central Google Scholar
Allison, A. C. & Clyde, D. F. Malaria in African children with deficient erythrocyte glucose-6-phosphate dehydrogenase. British Medical Journal 1 , 1346 (1961).
CAS PubMed PubMed Central Google Scholar
Allison, A. C. & Clyde, D. F. Malaria and glucose-6-phosphate dehydrogenase. British medical journal 2 , 521 (1961).
PubMed Central Google Scholar
Bottini, E., Gloria-Bottini, F. & Maggioni, G. On the relation between malaria and G-6-PD deficiency. Journal of medical genetics 15 , 363–365 (1978).
Awah, F. M., Chukwuemeka, G., Olalekan, S. I., Azeke, A. E. & Nneka, M. A possible protective role of glucose-6-phosphate dehydrogenase deficiency and sickle haemoglobin genes against severe malaria in Madonna University, Elele Community. Journal of Medicine and Medical Sciences 3 , 375–381 (2012).
Awah, F. M. & Uzoegwu, P. N. Malaria Protection In Glucose-6-Phosphate Dehydrogenase Deficient Individuals In Bamenda Population Of Cameroon. Global Journal of Pure and Applied Sciences 14 , 343–347 (2008).
Harris, R. & Gilles, H. M. Glucose‐6‐phosphate dehydrogenase deficiency in the peoples of the Niger Delta. Annals of human genetics 25 , 199–206 (1962).
Kidson, C. & Gorman, J. G. A challenge to the concept of selection by malaria in glucose-6-phosphate dehydrogenase deficiency. Nature 196 , 49–51 (1962).
Kruatrachue, M., Bhaibulaya, M., Clongkamnaukorn, K., Harinasuta, C. & Organization, W. H. Re-examination of the relationship of erythrocyte Glucose-6-Phosphate Dehydrogenase and malaria in Thailand (1966).
Kruatrachue, M., Charoenlarp, P., Chongsuphajaisiddhi, T. & Harinasuta, C. Erythrocyte glucose-6-phosphate dehydrogenase and malaria in Thailand. The Lancet 280 , 1183–1186 (1962).
Bernstein, S. & Bowman, J. G6PD/Malaria hypothesis: a balanced or transient polymorphism. The Lancet 315 , 485 (1980).
Gilles, H. M. & Taylor, B. G. The existence of the glucose-6-phosphate dehydrogenase deficiency trait in Nigeria and its clinical implications. Annals of Tropical Medicine & Parasitology 55 , 64–69 (1961).
CAS Google Scholar
Bienzle, U. Glucose-6-phosphate dehydrogenase deficiency. Part 1: Tropical Africa. Clinics in haematology 10 , 785–799 (1981).
Bienzle, U., Guggenmoos-Holzmann, I. & Luzzatto, L. Malaria and erythrocyte glucose-6-phosphate dehydrogenase variants in West Africa. The American journal of tropical medicine and hygiene 28 , 619–621 (1979).
Domarle, O. et al. Factors influencing resistance to reinfection with Plasmodium falciparum. The American journal of tropical medicine and hygiene 61 , 926–931 (1999).
Dunyo, S. et al. Randomized trial of safety and effectiveness of chlorproguanil-dapsone and lumefantrine-artemether for uncomplicated malaria in children in the Gambia. PLoS One 6 , e17371 (2011).
ADS CAS PubMed PubMed Central Google Scholar
Enevold, A. et al. Reduced risk of uncomplicated malaria episodes in children with alpha+-thalassemia in northeastern Tanzania. The American journal of tropical medicine and hygiene 78 , 714–720 (2008).
Fanello, C. I. et al. High risk of severe anaemia after chlorproguanil-dapsone+artesunate antimalarial treatment in patients with G6PD (A-) deficiency. PLoS One 3 , e4031 (2008).
ADS PubMed PubMed Central Google Scholar
Gilles, H. M. et al. Glucose-6-phosphate-dehydrogenase deficiency, sickling, and malaria in African children in South Western Nigeria. The lancet 289 , 138–140 (1967).
Guindo, A., Fairhurst, R. M., Doumbo, O. K., Wellems, T. E. & Diallo, D. A. X-linked G6PD deficiency protects hemizygous males but not heterozygous females against severe malaria. PLoS Med 4 , e66 (2007).
Lell, B. et al. The role of red blood cell polymorphisms in resistance and susceptibility to malaria. Clinical Infectious Diseases 28 , 794–799 (1999).
Oo, M., Tin-Shwe, M.-T. & O’Sullivan, W. J. Genetic red cell disorders and severity of falciparum malaria in Myanmar. Bulletin of the World Health Organization 73 , 659 (1995).
Orimadegun, A. E. & Sodeinde, O. Glucose-6-phosphate dehydrogenase status and severity of malarial anaemia in Nigerian children. The Journal of Infection in Developing Countries 5 , 792–798 (2011).
Segeja, M. D. et al. Prevalence of glucose-6-phosphate dehydrogenase deficiency and haemoglobin S in high and moderate malaria transmission areas of Muheza, north-eastern Tanzania. Tanzania journal of health research 10 , 9–13 (2008).
Leslie, T. et al. The impact of phenotypic and genotypic G6PD deficiency on risk of Plasmodium vivax infection: a case-control study amongst Afghan refugees in Pakistan. PLoS Med 7 , e1000283 (2010).
Brabin, L. & Brabin, B. J. Malaria and glucose 6-phosphate dehydrogenase deficiency in populations with high and low spleen rates in Madang, Papua New Guinea. Human heredity 40 , 15–21 (1990).
Jalloh, A. et al. Rapid epidemiologic assessment of glucose‐6‐phosphate dehydrogenase deficiency in malaria‐endemic areas in Southeast Asia using a novel diagnostic kit. Tropical Medicine & International Health 9 , 615–623 (2004).
Khim, N. et al. G6PD deficiency in Plasmodium falciparum and Plasmodium vivax malaria-infected Cambodian patients. Malaria journal 12 , 171 (2013).
Louicharoen, C. et al. Positively selected G6PD-Mahidol mutation reduces Plasmodium vivax density in Southeast Asians. Science 326 , 1546–1549 (2009).
Nkuo-Akenji, T. K., Wepngong, P. & Akoachere, J.-F. Effects of ABO/Rh blood groups, G-6-PD enzyme activity and haemoglobin. African journal of health sciences 11 , 93–97 (2004).
Tantular, I. S. et al. Field trials of a rapid test for G6PD deficiency in combination with a rapid diagnosis of malaria. Tropical Medicine & International Health 4 , 245–250 (1999).
Onuma, W. T. J. J. F., Tepanata, Z., Napaporn, P. N. H. A. S., Harnyuttanakorn, S. P. & Kanchanakhan, N. Prevalence of G6PD Deficiency in malaria endemic area: case study in bongti sub-district, sai yok district, kanchanaburi province, Thailand. J Health Res 24 , 55–62 (2010).
Santana, M. S. et al. Glucose-6-phosphate dehydrogenase deficient variants are associated with reduced susceptibility to malaria in the Brazilian Amazon. Transactions of the Royal Society of Tropical Medicine and Hygiene 107 , 301–306 (2013).
Shimizu, H., Tamam, M., Soemantri, A. & Ishida, T. Glucose-6-phosphate dehydrogenase deficiency and Southeast Asian ovalocytosis in asymptomatic Plasmodium carriers in Sumba island, Indonesia. Journal of human genetics 50 , 420–424 (2005).
Syaiiyuni, R. Hubungan defisiensi Glucose-6-phosphate dehydrogenase (G-6-PD) dengan kepadatan parasit malaria pada anak usia sekolah di daerah endemis malaria, Program Pendidikan Pasca sarjana Universitas Diponegoro , (2003).
Duval, S. & Tweedie, R. Trim and fill: a simple funnel‐plot–based method of testing and adjusting for publication bias in meta‐analysis. Biometrics 56 , 455–463 (2000).
CAS PubMed MATH Google Scholar
Scriver, C. R. et al. The Metabolic and Molecular Bases of Inherited Disease . 8th edit McGraw-Hill. New York (2001).
Wernsdorfer, W. H. & McGregor, I. Malaria: principles and practice of malariology . Vol. 1 (Churchill Livingstone Edinburgh, 1988).
Livingstone, F. B. Frequencies of hemoglobin variants: thalassemia, the glucose-6-phosphate dehydrogenase deficiency, G6PD variants, and ovalocytosis in human populations . (Oxford University Press, USA, 1985).
Uyoga, S. et al. Glucose-6-phosphate dehydrogenase deficiency and the risk of malaria and other diseases in children in Kenya: a case-control and a cohort study. The Lancet Haematology 2 , e437–e444 (2015).
Luzzatto, L. G6PD deficiency: a polymorphism balanced by heterozygote advantage against malaria. The Lancet Haematology 2 , e400–e401 (2015).
Usanga, E. A. & Luzzatto, L. Adaptation of Plasmodium falciparum to glucose 6-phosphate dehydrogenase-deficient host red cells by production of parasite-encoded enzyme , (1985).
Yoshida, A. & Roth, E. F. Jr. Glucose-6-phosphate dehydrogenase of malaria parasite Plasmodium falciparum. Blood 69 , 1528–1530 (1987).
Bienzle, U., Lucas, A., Ayeni, O. & Luzzatto, L. Glucose-6-phosphate dehydrogenase and malaria: greater resistance of females heterozygous for enzyme deficiency and of males with non-deficient variant. The Lancet 299 , 107–110 (1972).
Ruwende, C., Khoo, S. C., Snow, R. W., Green, L. S. & Danubio, M. E. Protection against severe malaria for glucose-6-phosphate dehydrogenase deficiency hemizygotes and heterozygotes in Africa. Adaptation to Malaria: The Interaction of Biology and Culture , 73–86 (1997).
Liberati, A. et al. The PRISMA statement for reporting systematic reviews and meta-analyses of studies that evaluate health care interventions: explanation and elaboration. PLoS medicine 6 , e1000100, doi: 10.1371/journal.pmed.1000100 (2009).
Article PubMed PubMed Central Google Scholar
Deeks, J. J., Altman, D. G. & Bradburn, M. J. In Systematic Reviews in Health Care: Meta-Analysis in Context (eds Egger M., Smith G. D. & Altman D. G. ) (BMJ Publishing Group, 2008).
Higgins, J. P., Thompson, S. G., Deeks, J. J. & Altman, D. G. Measuring inconsistency in meta-analyses. Bmj 327 , 557–560, doi: 10.1136/bmj.327.7414.557 (2003).
Higgins, J., Thompson, S. G., Deeks, J. J. & Altman, D. G. Measuring inconsistency in meta-analyses [journal article as teaching resource, deposited by John Flynn]. British medical journal 327 , 557–560 (2003).
Begg, C. B. & Mazumdar, M. Operating characteristics of a rank correlation test for publication bias. Biometrics , 1088–1101 (1994).
Egger, M., Smith, G. D., Schneider, M. & Minder, C. Bias in meta-analysis detected by a simple, graphical test. Bmj 315 , 629–634 (1997).
Download references
Acknowledgements
This work was supported in part by a “Grant-in-Aid for Scientific Research (B)” (16H05844, 2016–2019 for Nguyen Tien Huy) from Ministry of Education, Culture, Sports, Science and Technology (MEXT) of Japan and by the Japan Initiative for Global Research Network on Infectious Diseases (J-GRID) for Kenji Hirayama. The funders had no role in the study design, data collection and analysis, decision to publish or preparation of the manuscript. We thank the reviewers for their instructive comments which helped us to improve our study.
Author information
Evaristus Chibunna Mbanefo, Ali Mahmoud Ahmed, Afaf Titouna and Ahmed Elmaraezy: These authors contributed equally to this work.
Authors and Affiliations
Department of Immunogenetics, Institute of Tropical Medicine (NEKKEN), Nagasaki University, Sakamoto, Nagasaki, Japan
Evaristus Chibunna Mbanefo, Afaf Titouna & Kenji Hirayama
Department of Parasitology and Entomology, Nnamdi Azikiwe University, P.M.B. Awka, Nigeria
Evaristus Chibunna Mbanefo
Faculty of Medicine, Al-Azhar University, Cairo, Egypt
Ali Mahmoud Ahmed & Ahmed Elmaraezy
University of Medicine and Pharmacy at Hue City, Hue City, Vietnam
Nguyen Thi Huyen Trang
University of Medicine and Pharmacy at Ho Chi Minh City, Hong Bang, Ho Chi Minh, Vietnam
Nguyen Phuoc Long, Bui The Hung & Nguyen Ky Anh
School of Medicine, Vietnam National University, Ho Chi Minh City, Ho Chi Minh, Vietnam
Nguyen Hoang Anh, Tran Diem Nghi & Mai Van Hieu
Evidence Based Medicine Research Group & Faculty of Applied Sciences, Ton Duc Thang University, Ho Chi Minh City, Vietnam
Nguyen Tien Huy
Department of Clinical Product Development, Institute of Tropical Medicine (NEKKEN), Nagasaki University, Sakamoto, Nagasaki, Japan
You can also search for this author in PubMed Google Scholar
Contributions
E.C.M., A.M.A. and A.T. contributed in all steps including study screening, data extraction, analysis, and manuscript preparation; A.E. participated in data extraction and data analysis, manuscript writing, and made the supplemental tables; N.T.H.T., N.P.L., and N.H.A. wrote the protocol also participated in title and abstract screening, full-text screening and data extraction; T.D.N. and P.T.H. searched databases, participated in title and abstract screening, full-text screening and data extraction; M.V.H. and N.K.A. reviewed the protocol, participated in title and abstract screening and data extraction and N.T.H. proposed the search terms, manage the work, and reviewed data extraction, data analysis and manuscript; K.H. provided general supervision, reviewed data extraction, data analysis and manuscript.
Corresponding authors
Correspondence to Nguyen Tien Huy or Kenji Hirayama .
Ethics declarations
Competing interests.
The authors declare no competing financial interests.
Supplementary information
Supplementary information (pdf 3318 kb), rights and permissions.
This work is licensed under a Creative Commons Attribution 4.0 International License. The images or other third party material in this article are included in the article’s Creative Commons license, unless indicated otherwise in the credit line; if the material is not included under the Creative Commons license, users will need to obtain permission from the license holder to reproduce the material. To view a copy of this license, visit http://creativecommons.org/licenses/by/4.0/
Reprints and permissions
About this article
Cite this article.
Mbanefo, E., Ahmed, A., Titouna, A. et al. Association of glucose-6-phosphate dehydrogenase deficiency and malaria: a systematic review and meta-analysis. Sci Rep 7 , 45963 (2017). https://doi.org/10.1038/srep45963
Download citation
Received : 20 September 2016
Accepted : 07 March 2017
Published : 06 April 2017
DOI : https://doi.org/10.1038/srep45963
Share this article
Anyone you share the following link with will be able to read this content:
Sorry, a shareable link is not currently available for this article.
Provided by the Springer Nature SharedIt content-sharing initiative
This article is cited by
Genetic analysis and molecular basis of g6pd deficiency among malaria patients in thailand: implications for safe use of 8-aminoquinolines.
- Usa Boonyuen
- Beatriz Aira C. Jacob
- Shamini Chandran
Malaria Journal (2024)
First Insight into Drug Resistance Genetic Markers, Glucose-6-Phosphate Dehydrogenase and Phylogenetic Patterns of Misdiagnosed Plasmodium vivax Malaria in Far North Region, Cameroon
- Loick Pradel Kojom Foko
- Jahnvi Jakhan
- Vineeta Singh
Current Microbiology (2024)
Hemoglobinopathies, merozoite surface protein-2 gene polymorphisms, and acquisition of Epstein Barr virus among infants in Western Kenya
- Perez K. Olewe
- Shehu Shagari Awandu
- Collins Ouma
BMC Cancer (2023)
Genetic basis and spatial distribution of glucose-6-phosphate dehydrogenase deficiency in ecuadorian ethnic groups: a malaria perspective
- Sebastián Atarihuana
- Jennifer Gallardo-Condor
- Alejandro Cabrera-Andrade
Malaria Journal (2023)
Retinopathy of Prematurity and Glucose-6-Phosphate Dehydrogenase Activity: A Case-Control Study
- Rajarajan Paulpandian
- Sourabh Dutta
- Praveen Kumar
Indian Journal of Pediatrics (2023)
By submitting a comment you agree to abide by our Terms and Community Guidelines . If you find something abusive or that does not comply with our terms or guidelines please flag it as inappropriate.
Quick links
- Explore articles by subject
- Guide to authors
- Editorial policies
Sign up for the Nature Briefing newsletter — what matters in science, free to your inbox daily.

Study of Glucose-6-Phosphate Dehydrogenase Deficiency: 5 Years Retrospective Egyptian Study
Affiliations.
- 1 Pediatric Department, Faculty of Medicine, Tanta University, Gharbia, Egypt.
- 2 Public Health Department, Faculty of Medicine, Tanta University, Gharbia, Egypt.
- PMID: 28982343
- DOI: 10.2174/1871530317666171003160350
Background: Glucose-6-phosphate dehydrogenase (G6PD) deficiency is the most common enzyme deficiency worldwide that causes a spectrum of diseases including neonatal hyperbilirubinemia, acute and chronic hemolysis after exposure to oxidative stress.
Aim of the work: This five years retrospective study was carried out to study the demographic, clinical and laboratory data of 1000 patients with G6PD deficiency anemia registered in Hematology Unit, Pediatric Department, Tanta University Hospital.
Patients and methods: Data were collected from patient's files, from November 2011 to November 2016, using the pre-designed questionnaires to obtain the complete history, clinical presentation and laboratory investigations including the complete blood count, red blood cells morphology, liver and renal functions and quantitative assay of G6PD enzyme activity by spectrophotometric method.
Results: Males were more commonly affected than females (932 males versus 68 females). The highest prevalence of hemolytic crisis in G6PD deficiency patients was found within the age group of 1-3 years (920 patients; 92%) with mean age of the first presentation of 22.8±15.54 months. Patients presented mainly with pallor (1000 patients; 100%), dark red urine (896 patients; 89.6%) and jaundice (878 patients; 87.8%) after 24-72 hours of exposure to the precipitating factors (mean: 36±17.73 hours). Diets were the most common precipitating factor of hemolysis in patients with G6PD deficiency (834 patients; 83.4% of studied cases) especially fava beans (326 patients; 32.6%) and falafel (194 patients; 19.4%) which were the most common precipitating food products causing hemolysis followed by chick pea (108 patients; 10.8%), broad bean (76 patients; 7.6%), green pea (44 patients; 4.4%), pea nuts (38 patients; 3.8%), lentil (28 patients; 2.8%), and lastly black eyed peas (20 patients; 2 %). Infections were the 2nd most common cause of hemolysis (124 patients; 12.4%) including pneumonia (34 patients; 3.4%), tonsillitis (32 patients; 3.2%), typhoid fever (28 patients; 2.8%), hepatitis A (18 patients; 1.8%) and urinary tract infection (12 patients; 1.2%). Drugs were the least common cause of hemolysis (42 patients; 4.2%) including diclofenac sodium (24 patients; 2.4%), ibuprofen (8 patients; 0.8%), acetylsalicylic acid (4 patients; 0.4%), co-trimoxazole (4 patients; 0.4%) and nitrofurantion (2 patients; 0.2%). There was normocytic normochromic anemia with reticulocytosis and Heinz bodies in pre-transfusion complete blood picture in all studied cases. G6PD assay show marked decrease in enzyme level at time of presentation in all cases with the commonest G6PD enzyme level of 3-4 U/gm Hb (592 patients; 59.2%).
Conclusion and recommendations: G6PD deficiency anemia presented mainly with pallor, dark red urine and jaundice after exposure to certain diets, drugs and diseases and therefore patients with G6PD deficiency should avoid exposure to these precipitating factors of hemolysis. We can also recommend large neonatal screening programs to detect cases of G6PD deficiency before the occurrence of acute hemolysis and molecular studies to detect G6PD enzyme variant in Egypt.
Keywords: G6PD deficiency anemia; enzymopathy; heinz bodies; hemolytic crisis; red blood cell enzymes; retrospective study..
Copyright© Bentham Science Publishers; For any queries, please email at [email protected].
- Age Factors
- Anemia, Hemolytic / epidemiology
- Anemia, Hemolytic / etiology*
- Anemia, Hemolytic / physiopathology
- Cicer / adverse effects
- Egypt / epidemiology
- Family Health
- Foodborne Diseases / epidemiology
- Foodborne Diseases / etiology*
- Foodborne Diseases / physiopathology
- Glucosephosphate Dehydrogenase Deficiency / blood
- Glucosephosphate Dehydrogenase Deficiency / physiopathology*
- Hepatic Insufficiency / etiology*
- Hepatic Insufficiency / physiopathology
- Hospitals, University
- Kidney / physiopathology
- Liver / physiopathology
- Renal Insufficiency / etiology*
- Renal Insufficiency / physiopathology
- Retrospective Studies
- Seeds / adverse effects
- Severity of Illness Index
- Sex Factors
- Vicia faba / adverse effects

IMAGES
VIDEO
COMMENTS
Among inborn errors of metabolism, the phrase by which inherited disorders were formerly known, glucose-6-phosphate dehydrogenase (G6PD) deficiency was the first red cell enzymopathy to be identified (), and it proved unique in at least 2 ways.First, G6PD deficiency is a polymorphic genetic trait with worldwide distribution (a current estimate is that over 500 million people are affected), but ...
Glucose-6-phosphate dehydrogenase (G6PD) is an enzyme found in the cytoplasm of all cells in the body. It is a housekeeping enzyme that plays a vital role in the prevention of cellular damage from reactive oxygen species (ROS). It does this by providing substrates to prevent oxidative damage. Erythrocytes are particularly vulnerable to ROS due to their role in oxygen transport and inability to ...
Case Report. We present a case report of a 4-year-old child with glucose-6-phosphate dehydrogenase (G6PD) deficiency from . Centro Hospitalar do Porto, CHP, Porto, Portugal. Written informed consent was given by the child's mother. A male child, African descent, born at 38 . weeks, had normal neonatal anthropometry (body weight 3130 g; length ...
A case of G6PD deficiency. A 43-year-old man of Mediterranean descent presented to his primary care provider for a routine visit. During the examination, the provider noted some mild splenomegaly ...
A case report of a 4-year-old child with glucose-6-phosphate dehydrogenase deficiency: An evidence based approach to nutritional management. Turk J Pediatr 2017; 59: 189-192. The objective was to describe the nutritional management of a 4-year-old child with glucose-6-phosphate dehydrogenase (G6PD) deficiency.
Download ppt "Case Study 1: G6PD David Grkovicious Andrew Wattals Tim Cryal." Similar presentations . Practical Hematology Lab Glucose 6 Phosphate Dehydrogenase. Sickle Cell Anemia. Sickle cell anemia - abnormally shaped red blood cells that are shaped like a sickle (or crescent). Hard and sticky, sickle cells.
G6PD catalyzes nicotinamide adenine dinucleotide phosphate ... family studies, ... Kernicterus and G6PD deficiency—a case series from Oman. J Trop Pediatr. 2003;49:74-7. ...
INTRODUCTION. Glucose-6-phosphate dehydrogenase (G6PD) deficiency is an inherited disorder caused by a genetic defect in the red blood cell (RBC) enzyme G6PD, which generates NADPH and protects RBCs from oxidative injury. G6PD deficiency is the most common enzymatic disorder of RBCs.
Glucose-6-phosphatase dehydrogenase (G6PD) deficiency is the most common enzyme deficiency in humans, affecting 400 million people worldwide. ... (G6PD) Deficiency Clinical Presentation. Updated: Jun 27, 2023 Author: Srikanth Nagalla, MD, MS, FACP; Chief Editor: Emmanuel C Besa, ... A retrospective observational study. Medicine (Baltimore ...
glucose-6-phosphate dehydrogenase (G6PD) deficiency is an X-linked genetic disorder that causes an intrinsic hemolytic anemia. Epidemiology. prevalence. 7.1% worldwide. most common enzyme disorder of erythrocytes. demographics. more severe in males than females. common in areas where malaria is endemic.
G6PD deficiency renders RBCs susceptible to oxidative stress, which shortens RBC survival. Hemolysis occurs following an oxidative challenge, commonly after fever, acute viral or bacterial infections, and diabetic ketoacidosis Diabetic Ketoacidosis (DKA) Diabetic ketoacidosis (DKA) is an acute metabolic complication of diabetes characterized by hyperglycemia, hyperketonemia, and metabolic ...
Background Glucose-6-phosphate dehydrogenase deficiency is a rarely recognized predisposing factor for rhabdomyolysis. Rhabdomyolysis with coronavirus disease 2019 has been increasingly seen during the pandemic. We report the uncommon occurrence of coronavirus disease 2019 pneumonia, severe rhabdomyolysis, and acute renal failure in the setting of glucose-6-phosphate dehydrogenase deficiency ...
Presentation Transcript. Glucose-6-Phosphate Dehydrogenase (G-6-PD) Introduction • G6PD deficiency is an allelic abnormality which is inherited in an X-linked recessive fashion. • G6PD deficiency is also known as "favism" since G6PD deficient individuals are also sometimes allergic to fava beans. • Glucose-6-Phosphate Dehydrogenase (G6PD ...
Glucose-6-phosphate dehydrogenase (G6PD) is a house keeping enzyme critical in the redox metabolism of all aerobic cells. G6PD deficiency is the most common human enzyme defect. Though majority remains clinically asymptomatic the risk of developing AHA still remains. The three known trigger factors being (i) favism beans (ii) infections (iii ...
Case presentation. Here, we present the first documented case of kernicterus in Panama, in a glucose-6-phosphate dehydrogenase-deficient newborn clothed in naphthalene-impregnated garments, resulting in reduced psychomotor development, neurosensory hypoacousia, absence of speech and poor reflex of the pupil to light. Conclusion
Introduction: Glucose-6-phosphate dehydrogenase (G6PD) is an enzyme that plays an essential role in the human body cell. It is found in the cytoplasm of cells and functions to prevent cellular damage from reactive oxygen species. G6PD deficiency can be highly variable in its clinical presentation. It can cause acute or chronic hemolytic anemia and hyperbilirubinemia.
Clinical definition. glucose-6-phosphate dehydrogenase (G6PD) deficiency is an X-linked genetic disorder that causes an intrinsic hemolytic anemia. Epidemiology. Prevalence. 7.1% worldwide. most common enzyme disorder of erythrocytes. Demographics. more severe in males than females. common in areas where malaria is endemic.
The impact of phenotypic and genotypic G6PD deficiency on risk of Plasmodium vivax infection: a case-control study amongst Afghan refugees in Pakistan. PLoS Med 7 , e1000283 (2010).
28982343. 10.2174/1871530317666171003160350. Glucose-6-phosphate dehydrogenase (G6PD) deficiency is the most common enzyme deficiency worldwide that causes a spectrum of diseases including neonatal hyperbilirubinemia, acute and chronic hemolysis after exposure to oxidative stress. This five years retrospective study was carried out to study the ...
Most people with G6PD deficiency don't develop symptoms. In some instances, though, G6PD deficiency can cause serious medical conditions such as hemolytic anemia in adults and severe jaundice in newborns. Sometimes, anemia symptoms develop very quickly, causing hemolytic crisis symptoms that require immediate medical attention.